Heart failure (HF) is a common reason for hospital admissions, especially in older adults. Most patients hospitalized for HF have previously been diagnosed with HF but have subsequently developed progressive clinical and hemodynamic decompensation that leads to admission. All patients admitted to the hospital with HF should undergo a rapid clinical evaluation for risk stratification and to determine the degree of elevation in cardiac filling pressures and the adequacy of systemic perfusion. In addition, effective management should include a careful search for correctable or reversible factors. In selected cases, hemodynamic monitoring with a pulmonary artery catheter (PAC) may be indicated for the purpose of initiating and titrating positive inotropic, vasodilator, and diuretic therapy. Clinical and hemodynamic goals of therapy should be set, and specific pharmacologic agents must be chosen to meet these goals. In cases of refractory HF, evaluation for ventricular assist device (VAD) implantation or cardiac transplantation should be performed in select candidates. For those with end-stage HF who are not VAD or transplant candidates, strategies that focus on symptom management and end-of-life care are appropriate.
Terminology
Acute decompensated heart failure has been defined as HF with a relatively rapid onset of signs and symptoms, resulting in hospitalization or unplanned office or emergency department visits. A more recent term, acute heart failure syndromes , is defined more broadly as new onset or gradual or rapidly worsening HF that requires urgent therapy. Most of these episodes result from worsening or decompensation of chronic HF. Stage D heart failure , as defined by the American College of Cardiology/American Heart Association (ACC/AHA) guidelines, designates patients with refractory HF who might be eligible for specialized advanced treatment strategies (e.g., mechanical circulatory support [MCS], continuous inotropic infusions) or end-of-life care ( Figure 14-1 ). Approximately 20% of hospitalizations are due to new-onset HF, also termed acute heart failure , which may be caused by an acute coronary syndrome, uncontrolled hypertension, or acute valvular dysfunction. Acute pulmonary edema is a clinical term used to describe patients who are seen with rapid worsening of symptoms associated with physical and radiologic findings of pulmonary congestion as a result of acute elevation of the pulmonary capillary wedge pressure.

Epidemiology
It is estimated that 5,700,000 adults (2.4%) aged 20 years or older in the United States are being treated for HF, and 620,000 new cases are diagnosed each year. The prevalence of HF increases dramatically with age, occurring in 1% to 2% of people aged 40 to 59 years and in up to 12% of individuals older than 80 years. The annual rates per 1000 population of new HF events for white men are 15.2 for those aged 65 to 74 years, 31.7 for those aged 75 to 84 years, and 65.2 for those 85 years and older. Between 1979 and 2006, the number of HF discharges rose from 390,000 to 1,100,000—an increase of 182% ( Figure 14-2 ). Approximately 80% of all HF admissions occur in patients older than 65 years; as a result, HF is the leading discharge diagnosis among Medicare beneficiaries, with an average length of stay of 5.3 days. Hospitalizations are common after an HF diagnosis in the community, with 83% hospitalized once and 43% hospitalized at least four times.

Over the past decade, large registries have defined the demographics and concomitant diseases associated with acute decompensated HF ( Table 14-1 ). Approximately half of patients are women, and nearly 75% have a history of HF. Hypertension and coronary artery disease are present in more than 50% of patients, and 30% or more of hospitalized HF patients come to medical attention with diabetes, atrial fibrillation, and renal dysfunction.
ADHERE (N = 107,920) | EHFS (N = 11,327) | OPTIMIZE-HF (N = 48,612) | |
---|---|---|---|
Mean age (yr) | 75 | 71 | 73 |
Women (%) | 52 | 47 | 52 |
Prior heart failure (%) | 75 | 65 | 88 |
LVEF <40% (%) | 59 | 35 | 52 |
CAD (%) | 57 | 68 | 46 |
Hypertension (%) | 72 | 53 | 71 |
Diabetes (%) | 44 | 27 | 42 |
Atrial fibrillation (%) | 31 | 43 | 31 |
Renal dysfunction (%) | 30 | 17 | 20 |
Admission to the hospital with HF is associated with poor short- and long-term outcomes. The Acute Decompensated Heart Failure National Registry (ADHERE) tracked outcomes of over 107,000 HF hospitalizations and found an in-hospital mortality rate of 4.0%. In the EuroHeart Survey of more than 11,000 admissions, 6.9% of patients died during an HF hospitalization. In community-based studies, crude mortality rates following an HF hospitalization range from 8% to 14% at 30 days and increase to 26% to 37% at 1 year. For survivors, readmission with HF is common, ranging from 20% to 25% at 60 days and increasing to nearly 50% at 6 months. With each subsequent admission, the risk of dying increases. In a Canadian cohort of more than 14,000 patients, median survival after the first, second, third, and fourth HF hospitalization was 2.4, 1.4, 1.0, and 0.6 years, respectively ( Figure 14-3 ).

As a result of direct medical costs, disability, and loss of employment, HF has an enormous economic impact on the U.S. health care system. In 2003, $3.6 billion ($5,456 per discharge) was spent on Medicare beneficiaries for the in-hospital management of HF. Between 2000 and 2007, the mean costs to Medicare per patient for HF care in the last 6 months of life increased 26%—from $28,766 to $36,216—with chronic kidney and lung disease being independent predictors of higher costs. In 2010, the total estimated direct and indirect cost of HF in the United States was $39.2 billion. Notably, high-volume HF centers demonstrate improved quality of care and outcomes, but at higher costs, compared with low-volume hospitals.
Pathophysiology
Heart Failure with Reduced Versus Preserved Ejection Fraction
In patients with reduced systolic function (i.e., ejection fraction <40%), HF reflects an abnormality of contractile function, in which the end-systolic pressure/volume relationship is shifted downward. End-systolic volume, end-diastolic volume, and end-diastolic pressure are increased, and elevated end-diastolic filling pressures are transmitted to the pulmonary venous circulation, resulting in signs and symptoms of pulmonary congestion. In some patients, elevated right-sided filling pressures cause systemic venous and hepatic congestion, leading to symptoms of abdominal discomfort. Contractile dysfunction may result from both direct and indirect insults to the myocardium, including loss of myocytes following myocardial infarction (MI); chronic volume or pressure overload; exposure to toxins, such as alcohol or doxorubicin; or a primary myocardial process, such as idiopathic dilated cardiomyopathy. Decreased contractility results in a fall in stroke volume, leading to symptoms of decreased cardiac output.
Up to 50% of patients admitted to the hospital with HF have normal or near normal left ventricular (LV) systolic function (see Table 14-1 ) and are presumed to have an abnormality in diastolic function. The end-diastolic pressure/volume relationship may be shifted upward, reflecting impaired ventricular filling. Pulmonary vascular and systemic venous congestion results from an increase in left and right ventricular end-diastolic pressures, respectively. Diastolic dysfunction may be due to impaired early relaxation, increased chamber stiffness, or both of these, and it is frequently associated with hypertension and abnormal vascular compliance. Maladaptive volume regulation may also contribute to HF with preserved ejection fraction, and evidence suggests that in elderly hypertensive patients, volume expansion, renal dysfunction, and anemia play important roles in disease progression. Common underlying causes of HF with preserved ejection fraction include ischemia and LV hypertrophy; restrictive, infiltrative, and hypertrophic cardiomyopathies are encountered less frequently.
In an effort to promote standardization in practice, specific diagnostic criteria for heart failure with preserved ejection fraction, initially referred to as diastolic heart failure, were proposed. However, it is important to remember that HF with reduced ejection fraction and HF with preserved ejection fraction are not mutually exclusive. Although use of older terms, such as systolic and diastolic HF , underscored a predominant pathophysiologic mechanism operating in the individual patient, many if not most patients with HF have abnormalities of both systole and diastole. In patients with ischemic cardiomyopathy, for example, HF with reduced ejection fraction is caused by both chronic loss of myocardium secondary to infarction and the acute loss of contractility as a result of ischemia; diastolic dysfunction is due to reduced compliance caused by chronic replacement fibrosis and the acute reduction in distensibility by ischemia.
From a mechanistic standpoint, studies using implantable hemodynamic monitors have shown that elevated diastolic pressures play a pivotal role in the underlying pathophysiology of acute decompensation in patients with either reduced or preserved ejection fraction. Furthermore, for the purposes of in-hospital management, similar principles are often applied to patients regardless of ejection fraction. Although most of the approved diagnostic tools and treatment strategies have been validated in patients with reduced ejection fraction, recent acute HF trials have aimed to enroll a more heterogeneous population. In the ADHERE national registry, in-hospital mortality rate was lower in patients with preserved ejection fraction (2.8% vs. 3.9%), although intensive care unit (ICU) stay and total length of stay were similar. In the Organized Program to Initiate Lifesaving Treatment in Hospitalized Patients with Heart Failure (OPTIMIZE-HF), patients with an ejection fraction of 40% or higher also had similar lengths of stay and lower in-hospital mortality rates (2.9% vs. 3.9%), with no difference in death or rehospitalization rates at 60 to 90 days. More complete discussions of HF with preserved ejection fraction may be found in Chapter 12 and elsewhere.
Acute Compensatory Mechanisms
In the presence of a primary abnormality in contractile function, the heart depends on a number of compensatory mechanisms to maintain adequate cardiac output and vital organ perfusion (see Braunwald, Chapter 25 ). Acutely, ventricular performance may be maintained within normal limits by an increase in preload: the higher the preload, the greater the force of ventricular contraction and the greater the stroke volume. However, the Frank-Starling mechanism is advantageous only if the relationship between preload and contractility is positive. Although this may hold true in the setting of acute ischemia with normal chamber dimensions, in chronic systolic HF associated with LV dilation, the ventricular function curve may be flat at higher diastolic volumes ( Figure 14-4, A ). Increased filling may result in little augmentation in cardiac output, and further elevation in end-diastolic pressures may result in increased wall stress, decreased subendocardial perfusion, and worsening mitral regurgitation. Hemodynamic studies in patients with acute decompensated HF have shown that maximal stroke volumes can be achieved by lowering pulmonary capillary wedge pressure (PCWP) into the normal range with parenteral vasodilator ( Figure 14-4, B ) and diuretic therapy. This improvement in ventricular function may be due in large part to a reduction in secondary mitral or tricuspid regurgitation or both, which often complicates dilated ischemic or nonischemic cardiomyopathy but may also reflect improved myocardial oxygen supply and demand.

In addition to the beat-to-beat alterations in contractile function determined by the Frank-Starling mechanism, short-term stabilization of cardiac output or blood pressure may be achieved by activation of the renin-angiotensin-aldosterone system (RAAS) and sympathetic nervous system. Decreased perfusion pressure sensed by carotid sinus and aortic arch receptors results in increased sympathetic and decreased parasympathetic nervous system activity. Consequences of an increase in circulating catecholamines during cardiac decompensation include increased heart rate and contractility to augment cardiac output, systemic vasoconstriction to increase preload and maintain systolic blood pressure, and redistribution of blood flow away from the skin and splanchnic beds to the heart and central nervous system. Activation of the RAAS acts in concert with increased sympathetic activity to maintain arterial pressure. Elevated levels of circulating angiotensin II result in systemic vasoconstriction and intravascular volume expansion, and the latter leads to increased aldosterone secretion by the adrenal cortex and sodium and water retention by the kidney. Elevated circulating levels of arginine vasopressin and endothelin may also contribute to volume expansion and vasoconstriction in acute decompensated HF. In response to an increase in intracardiac pressure and volume, atrial and B-type natriuretic peptides are secreted from cardiac chambers and act as counterregulatory hormones by causing vasodilation, diuresis, natriuresis, and aldosterone and endothelin antagonism.
Myocardial Injury
Circulating levels of cardiac troponin are increased in the serum of patients with advanced HF and may increase further during episodes of acute decompensation in the absence of ischemia. Furthermore, elevated cardiac troponins predict adverse short-term outcomes, including worsening HF and in-hospital mortality, independent of ischemia ( Figure 14-5 ). Even patients with negative troponin levels on admission may develop positive levels during admission, and troponin conversion predicts worse outcomes at 60 days that are not significantly different from the risk of positive troponin at baseline. These clinical observations raise the possibility that acute HF syndromes are associated with an accelerated loss of cardiac myocytes, which in turn contributes to ventricular remodeling and disease progression. Underlying mechanisms of myocyte loss include necrosis and apoptosis. In addition to ischemia, several apoptotic stimuli are active in the setting of acute decompensation, including oxidative stress, mechanical strain, and neurohormones (e.g., norepinephrine and angiotensin).

Common Precipitating Factors of Heart Failure
Patients with HF may be asymptomatic or mildly symptomatic, either because the cardiac impairment is mild or because compensatory mechanisms help to balance or normalize cardiac function. Symptoms of HF that necessitate hospital admission may develop when one or more precipitating factors increases cardiac workload and disrupts the balance in favor of decompensation ( Box 14-1 ). Specific factors may be identified in 50% to 90% of admissions and are often correctable. In a report from the Randomized Evaluation of Strategies for Left Ventricular Dysfunction (RESOLVED) pilot study, the most commonly identified causes of HF exacerbation included excessive salt intake in 22%, other noncardiac disorders in 20%, arrhythmias in 13%, and upper respiratory tract infections in 11%. In the OPTIMIZE-HF registry, one or more precipitating factors was identified in 61% of patients, with pneumonia (15%), ischemia (15%), and arrhythmia (14%) being the most common.

Myocardial ischemia
Uncontrolled hypertension
Tachyarrhythmias or bradyarrhythmias
Nonadherence with medications and/or salt and fluid restriction
Heavy alcohol consumption
Periodic hypoxia (e.g., as a result of sleep apnea, COPD)
Anemia
Recent viral illness
Noncardiac medication causing fluid retention (e.g., NSAIDs, pregabalin)
Acute kidney injury or worsening renal function with diuretic refractoriness
Hyperthyroidism
COPD, chronic obstructive pulmonary disease; NSAIDs, nonsteroidal antiinflammatory drugs.
In a study of 435 patients admitted nonelectively to an urban hospital with the diagnosis of HF, acute chest pain and noncompliance with medications or diet were the precipitating factors in 33% and 21%, respectively. The rate of nonadherence with HF medications is particularly high among the elderly, although it has improved modestly over time. Despite a higher risk profile, nonadherent patients have been shown to have a lower in-hospital mortality rate and shorter length of stay, suggesting that it may be easier to achieve clinical stability by reinstituting sodium and/or fluid restriction and resuming medical therapy. Failure to take prescribed HF medications and adhere to dietary restrictions may result in progressive fluid retention and worsening congestion; however, inadequate therapy is not always the result of patient nonadherence. Physician underutilization of RAAS inhibitors and β-blockers in patients with HF is well documented. Likewise, digoxin may be withdrawn because of concerns about toxicity, or the diuretic dose may be decreased in the face of worsening renal function, thereby precipitating HF hospitalization.
Cardiac arrhythmias are common in patients with HF and often precipitate or exacerbate episodes of moderate to severe decompensation. Atrial fibrillation with rapid ventricular response results in elevated atrial pressures and may further reduce cardiac output in a patient with limited baseline systolic reserve. In patients with ischemic heart disease, tachycardia may induce or intensify ischemia and result in worsening systolic or diastolic function or both by increasing myocardial oxygen demands. In addition, a regular, narrow complex tachycardia may occasionally be misinterpreted as sinus in origin, when in fact atrial flutter or atrioventricular nodal reentry tachycardia is present. In some cases, atrial arrhythmias may be the primary cause of a tachycardia-mediated cardiomyopathy, which is potentially reversible with effective antiarrhythmic therapy or radiofrequency ablation. New onset of atrial arrhythmias warrants assessment of thyroid function, particularly in the elderly.
Although less common than atrial arrhythmias, sustained or paroxysmal nonsustained ventricular tachycardia often associated with implantable cardioverter-defibrillator (ICD) therapies may precipitate admission to the hospital in a patient with impaired LV function. In patients with HF and either reduced or preserved ejection fraction, symptomatic bradyarrhythmias may be due to intrinsic sinus or atrioventricular nodal dysfunction, adverse drug effects, or electrolyte abnormalities (e.g., hyperkalemia). Early recognition and aggressive management of cardiac arrhythmias may be critical to achieving the goal of recompensation; however, it should be remembered that in addition to precipitating HF, arrhythmias may be caused by HF, or they may result from proarrhythmic effects of drugs used in the management of HF. Although not an arrhythmia per se, ventricular dyssynchrony induced by right ventricular (RV) pacing is associated with an increased risk of HF hospitalization and atrial fibrillation and warrants consideration of biventricular pacing.
Myocardial ischemia or infarction should be considered as a possible precipitant, not only in patients with known ischemic heart disease presenting with HF, but also in patients with other forms of heart disease. For example, patients with previous HF secondary to hypertension or valvular heart disease may be well compensated until they develop unstable angina or an acute coronary syndrome. Diabetic patients with silent ischemia or infarction may also come to medical attention with insidious HF. Infection is another common precipitating cause of HF. In one study, 11% of patients with an exacerbation of HF had pneumonia. Common viral infections, including influenza A and respiratory syncytial virus (RSV), may also cause acute-on-chronic impairment of ventricular function and precipitate an admission with acute decompensated HF. It is not known whether direct viral infection of the myocardium, myocardial inflammation, or myocardial dysfunction secondary to circulating or local cytokines plays a significant role in such patients. Guidelines recommend that all patients with current or prior symptoms of HF receive yearly influenza vaccinations, which have been shown to be safe and effective in reducing the risk of hospitalization for pneumonia and death, especially in older patients.
Comorbid conditions common in patients with HF that may exacerbate symptoms leading to hospitalization include uncontrolled hypertension and anemia. In addition, the development of acute kidney injury or worsening renal function with or without diuretic refractoriness (see Cardiorenal Syndrome below) may further impair the ability of patients with HF to excrete sodium or free water and thus may exacerbate fluid retention. Other potentially correctable factors contributing to acute decompensation in HF include excessive alcohol consumption and medications that either depress myocardial function (β-blockers, amiodarone) or cause salt and water retention (nonsteroidal antiinflammatory drugs, thiazolidinediones, pregabalin). It is essential to search for these and other less common precipitating causes, such as hyperthyroidism or pulmonary embolism, in patients admitted to the hospital with HF because failure to recognize such conditions may lead to refractory or recurrent HF.
General Management
Initial Patient Evaluation
Regardless of the etiology or underlying precipitant, patients admitted to the hospital for the treatment of HF have the potential for hemodynamic deterioration, including the development of cardiogenic shock. Therefore, a rapid bedside evaluation of circulatory status and cardiac rhythm and assessment for ischemia should be performed even before the medical records or diagnostic studies are reviewed. In addition to ruling out an acute coronary syndrome that may require urgent revascularization or thrombolysis, evidence for marked elevation of intracardiac filling pressures and/or inadequacy of systemic perfusion should be sought. In selected cases, positive inotropic or vasopressor therapy may need to be initiated prior to obtaining additional diagnostic data or invasive hemodynamic measurements. However, in the majority of patients who are clinically more stable, a complete review of the medical history and a detailed physical exam may provide important information regarding the underlying cause and acute precipitants of HF as well as appropriate targets of therapy.
Risk Stratification
Clinical information obtained at the time of an HF admission may help to predict outcomes during the hospitalization and after discharge. Chin and Goldman first sought to determine the risk of a major complication or death in 435 patients admitted to an urban university hospital with worsening HF. Two thirds of the patients had a previous history of HF. Compared with those with new onset HF, these patients were older and had more comorbidities, lower LV ejection fraction (LVEF), and lower initial blood pressure. In a multivariate analysis, independent predictors of in-hospital death or major complications noted at the time of admission were a systolic blood pressure less than 90 mm Hg, respiratory rate greater than 30 breaths/min, serum sodium less than or equal to 135 mEq/L, and ischemic electrocardiographic changes not known to be old.
Using data from the ADHERE registry, Fonarow and colleagues sought to develop a practical bedside tool for risk stratification of patients with acute decompensated HF. Derivation and validation cohorts of more than 65,000 patient records were subjected to classification and regression tree (CART) analysis to identify predictors of in-hospital mortality. Based on this empirical analysis, the single best predictor for mortality was an elevated admission level of blood urea nitrogen (BUN) of 43 mg/dL or higher; followed by systolic blood pressure less than 115 mm Hg; and serum creatinine of 2.75 mg/dL or higher. A simple risk tree identified patient groups with mortality rates ranging from 2.3% to 19.8% ( Table 14-2 ). Similarly, the OPTIMIZE-HF investigators developed a risk-prediction nomogram based on data derived from 48,612 HF admissions. Multivariable predictors of mortality included age, heart rate, systolic blood pressure, sodium, creatinine, HF as a primary cause of hospitalization, and presence or absence of LV systolic dysfunction ( Table 14-3 , Figure 14-6 ). Additional clinical and laboratory predictors of adverse outcomes obtained at the time of hospital admission include presence of congestion, reduced LVEF, ischemic etiology, anemia, elevated natriuretic peptide levels, and positive cardiac troponins.
BUN ≥43 mg/dL | SBP <115 mm Hg | CREATININE ≥2.75 mg/dL | MORTALITY RATE (%) |
---|---|---|---|
− | − | − | 2.3 |
+ | − | − | 5.7 |
− | + | − | 5.7 |
+ | + | − | 13.2 |
+ | + | + | 19.8 |
PARAMETER | SCORE |
---|---|
Age (yr) | |
20 | 0 |
25 | 2 |
30 | 3 |
35 | 5 |
40 | 6 |
45 | 8 |
50 | 9 |
55 | 11 |
60 | 13 |
65 | 14 |
70 | 16 |
75 | 17 |
80 | 19 |
85 | 20 |
90 | 22 |
95 | 24 |
Heart Rate (beats/min) | |
65 | 0 |
70 | 1 |
75 | 1 |
80 | 2 |
85 | 3 |
90 | 4 |
95 | 4 |
100 | 5 |
105 | 6 |
110 | 6 |
SBP (mm Hg) | |
50 | 22 |
60 | 20 |
70 | 18 |
80 | 16 |
90 | 14 |
100 | 12 |
110 | 10 |
120 | 8 |
130 | 6 |
140 | 4 |
150 | 2 |
160 | 0 |
Sodium (mEq/L) | |
110 | 13 |
115 | 11 |
120 | 9 |
125 | 7 |
130 | 4 |
135 | 2 |
140 | 0 |
145 | 2 |
150 | 4 |
160 | 8 |
165 | 10 |
170 | 12 |
Serum Creatinine (mg/dL) | |
0 | 0 |
0.5 | 2 |
1 | 5 |
1.5 | 7 |
2 | 10 |
2.5 | 12 |
3 | 15 |
3.5 | 17 |
Primary Cause of Admission | |
Heart failure | 0 |
Other | 3 |
Left Ventricular Systolic Dysfunction | |
No | 0 |
Yes | 1 |

Clinical Assessment of Intracardiac Filling Pressures
Most hospitalizations for acute decompensated HF occur because of congestion rather than low cardiac output. Although congestion is often readily apparent in patients with de novo or acute HF, marked elevation in filling pressures may be underappreciated in patients with chronic HF. In one study of nonedematous HF patients, unrecognized hypervolemia was present in 65% and predicted reduced survival. Recent studies using implantable hemodynamic monitors demonstrate that cardiac filling pressures gradually rise over the days and weeks preceding an HF admission, with signs and symptoms lagging behind or not appreciated at all. Increased capacity for pulmonary lymphatic drainage resulting from chronic pulmonary congestion may lead to clinical situations in which PCWPs exceeding 30 to 35 mm Hg are tolerated. Conversely, a history of dyspnea on exertion is common in patients with HF and lacks the specificity to detect elevated cardiac filling pressures. Alternative mechanisms of dyspnea include decreased pulmonary function, increased ventilatory drive, and respiratory muscle fatigue. Not surprisingly, investigators have been unable to demonstrate a close correlation between symptoms or exercise tolerance and measures of resting LV performance in patients with HF. However, dyspnea on minimal exertion or at rest, including orthopnea and paroxysmal nocturnal dyspnea, are more specific historic clues to increased left-sided filling pressures in patients with known LV failure. In patients with biventricular HF, abdominal discomfort, early satiety, nausea, vomiting, and anorexia suggest increased right-sided cardiac filling pressures. In addition, changes in baseline symptoms in an individual patient may suggest a clinically important worsening of HF.
Rales are a relatively specific but insensitive sign of pulmonary vascular congestion. In a series of patients with chronic HF and reduced ejection fraction, rales were present in only 60% of patients with an elevated PCWP. However, rales were also detected in 16% of patients with normal PCWP and may be due to coexistent pulmonary or pleural disease. In both the ADHERE and OPTIMIZE-HF registries, rales were present in approximately two thirds of patients with acute decompensated HF. Signs of increased right-sided filling pressures—including jugular venous distension, ascites, and peripheral edema—are generally reliable indicators of increased left-sided filling pressures. However, in specific clinical situations, such as with right ventricular infarction or pulmonary embolism, jugular venous distension may occur without signs of left-sided congestion. Recent data also suggest that mismatch between right- and left-heart pressures may be present in up to one third of acute HF patients. Significant interobserver variability regarding the extent of jugular venous elevation further limits its usefulness at the bedside. Both ascites and peripheral edema are less sensitive signs of volume overload than jugular venous distension, particularly in young adults, and may be preceded by the development of tender hepatomegaly. A third heart sound may suggest the presence of previously undiagnosed cardiomyopathy and carries prognostic value, but it is neither sensitive nor specific for acute decompensated HF. Increased intensity of the pulmonic component of the second heart sound in association with a holosystolic murmur suggests pulmonary hypertension as a result of chronic HF and associated mitral regurgitation, tricuspid regurgitation, or both.
Rarely, experienced examiners may use the bedside Valsalva maneuver with sphygmomanometric determination of arterial blood pressure response to help clarify the LV filling pressure. However, arrhythmias and limited patient effort because of dyspnea or discomfort may interfere with this exam. An automated device that quantifies the blood pressure response to the Valsalva maneuver, as well as contrast-enhanced and tissue Doppler echocardiographic techniques, have been developed for the noninvasive determination of left ventricular filling pressures. In addition, intrathoracic impedance monitoring, plasma volume analysis, and lung ultrasound are newer methods to assess fluid status in HF patients; however, these techniques may lack accuracy in a heterogeneous population of patients with acute decompensated HF, and prospective data demonstrating the utility of these tools in patient management are lacking.
Like the physical exam, routine chest radiography has been shown to be an insensitive clinical tool for demonstrating the presence of worsening HF. In an initial report, 68% of patients with advanced HF and PCWP of 25 mm Hg or more had minimal or no evidence of pulmonary congestion on admission chest radiograph. In the ADHERE registry, 15,937 of 85,376 patients (19%) had no signs of congestion on emergency department chest radiography, and these patients were more likely to be given a non-HF diagnosis. When present, however, interstitial or alveolar edema suggests markedly elevated filling pressures. Bilateral or unilateral pleural effusions are common in acute decompensated HF and may not necessarily respond to diuresis. In these instances, ultrasound-guided thoracentesis or chest tube thoracostomy may be necessary to relieve respiratory distress and improve arterial oxygenation.
Clinical Assessment of Systemic Perfusion
Fatigue and weakness, which are commonly reported in patients with HF, may be due to reduced cardiac output and poor perfusion of skeletal muscles. In addition, abnormal endothelium-dependent vasodilation respiratory muscle fatigue and altered skeletal muscle structure and function may contribute to exercise intolerance in HF. However, fatigue is a nonspecific symptom that may be caused by many noncardiopulmonary diseases (anemia, chronic kidney disease, hypothyroidism, depression), disordered sleep (from sleep apnea, restless legs), drugs (β-blockers, sedatives), or electrolyte abnormalities. If a patient is admitted to the hospital with acute decompensated HF, mental obtundation or anuria is highly suggestive of critically reduced perfusion. A more subtle history of altered mentation, such as lack of concentration or memory, may be obtained from a family member. In severe low-output states, the patient may appear anxious or diaphoretic or may exhibit signs of air hunger. Patients with advanced HF may be cachectic from a low-output state and may have a catabolic/anabolic imbalance, whereas those with more recent onset HF will be well nourished. Other physical signs of acute hypoperfusion include cool extremities, digital or circumoral cyanosis, and marked hypotension with a rapid, thready pulse. Pulsus alternans signifies advanced myocardial disease and tends to be present during tachycardia.
When measuring blood pressure noninvasively, it is important to deflate the cuff slowly and listen closely to define the systolic blood pressure and pulse pressure, especially in patients with atrial fibrillation. Prior studies have shown that when the pulse pressure is less than 25% of the systolic blood pressure, the likelihood is high that the cardiac index is below 2.2 L/min/m 2 in such a patient. Thus, in someone with severe HF, a blood pressure of 100/90 mm Hg may be more worrisome than a blood pressure of 80/50 mm Hg. However, data from the Evaluation Study of Congestive Heart Failure and Pulmonary Artery Catheterization Effectiveness (ESCAPE) demonstrated a weak relationship between estimated and measured cardiac index.
Laboratory Assessment
Routine chemistry and hematology studies obtained on admission may be helpful in the initial assessment of patients with acute decompensated HF and may assist in formulating a therapeutic plan. Dilutional hyponatremia may result from a combination of sodium restriction, aggressive diuresis, and an impaired ability to excrete free water. Increased circulating levels of renin and vasopressin are also important mediators of hyponatremia in HF. Although angiontensin-converting enzyme (ACE) inhibitors alone or in combination with loop diuretics may acutely correct hyponatremia in severely ill patients with HF, overactivity of the RAAS may predispose these same patients to the development of hypotension, worsening renal function, and hyperkalemia upon initiation of, or rechallenge with, an ACE inhibitor. Use of nonsteroidal antiinflammatory agents should be avoided in hyponatremic patients, as they may aggravate hemodynamic abnormalities and cause worsening renal function.
Prolonged, high-dose diuretics may predispose patients to hypokalemia and hypomagnesemia, both of which are associated with an increased risk of ventricular arrhythmias. Hyperkalemia may result from marked reductions in glomerular filtration rate (GFR) and inadequate delivery of sodium to the distal renal tubule. Excess total body potassium may be exacerbated by the use of RAAS inhibitors, potassium-sparing diuretics, or potassium supplements. The risk of hyperkalemic arrhythmias appears to be highest during potassium replacement, when intracellular potassium concentrations are still low; and hyperkalemia is a common cause of iatrogenic morbidity, and even mortality, during an HF hospitalization. Other laboratory abnormalities that may complicate management of acute decompensated HF include increased liver enzymes, hyperbilirubinemia, and elevated international normalized ratio. If right-sided HF is long-standing, cardiac cirrhosis may result in hypoalbuminemia and an exacerbation of extravascular fluid accumulation as well as inappropriate vasodilation. Low serum prealbumin levels and elevated C-reactive protein levels are proinflammatory markers associated with cachexia in patients with end-stage HF.
In patients with chronic HF, BUN, and serum creatinine levels are elevated secondary to reductions in renal blood flow and glomerular filtration rate (GFR). Decreased renal perfusion can precipitate bouts of acute tubular necrosis and lead to reduced renal mass. Other factors contributing to the presentation of combined cardiac and renal dysfunction, or cardiorenal syndrome, include an altered balance of vasoconstrictor and vasodilating hormones and comorbidities such as diabetes, hypertension, and peripheral arterial disease. In patients with advanced HF, several commonly used classes of drugs, including inhibitors of the RAAS and diuretics, may exacerbate renal impairment. During an HF hospitalization, between 15% and 30% of patients will develop worsening renal function, defined variably as an increase in serum creatinine by 0.3 mg/dL or more or reduction in estimated GFR by 25% or more. The mechanisms underlying worsening renal function remain unknown but are thought to include diuretic-induced reductions in GFR, neurohormonal activation, and a fall in systolic blood pressure. From a clinical standpoint, worsening renal function may be transient or persistent and often results in interruption of diuretic therapy and discontinuation of other disease-modifying agents such as ACE inhibitors and aldosterone receptor antagonists ( Box 14-2 ). Most studies show that worsening renal function is an independent predictor of increased length of stay and excess mortality both in the hospital and after discharge, although emerging data suggest that baseline renal dysfunction primarily impacts outcomes.

Hospital discharge is delayed.
Diuretic doses are decreased, often despite persistent congestion.
ACE inhibitors, ARBs, and aldosterone receptor antagonists are discontinued.
Noncardiac medications (e.g., antibiotics) are dose-adjusted.
Positive inotropic agents are initiated to increase renal perfusion.
A pulmonary artery catheter is placed to assess hemodynamics.
A urinary catheter is placed to record urine output.
Renal ultrasound is ordered to rule out postobstructive uropathy.
ACE, angiotensin-converting enzyme; ARB, angiotensin receptor blocker.
B-Type Natriuretic Peptide
B-type natriuretic peptide (BNP) is synthesized and secreted by the cardiac ventricles in response to an increase in wall stress or filling pressure. N-terminal pro-BNP (NT-proBNP) is an inactive fragment that results from cleavage of BNP from its prohormone. Both BNP and NT-proBNP can be used to help diagnose and risk stratify patients with acute decompensated HF according to risk. In patients with chronic HF and reduced ejection fraction, BNP levels are elevated in relation to disease severity and provide strong independent prognostic information. Plasma natriuretic peptide levels are also elevated in patients with HF and preserved ejection fraction, correlate with wall stress, and can predict morbidity and mortality. In the acute setting, low or normal BNP (<100 pg/mL) or NT-proBNP (<500 pg/mL) levels have a reasonably high negative predictive value for excluding HF as the cause of dyspnea. According to current practice guidelines, BNP or NT-proBNP levels should be measured in patients being evaluated for dyspnea in which the contribution of HF is not known, but these must be interpreted in the context of all available clinical data.
In one study of patients presenting to the emergency room with shortness of breath, patients with HF and preserved ejection fraction had lower BNP levels compared with those with HF and reduced ejection fraction (median, 413 pg/mL vs. 812 pg/mL, respectively; Figure 14-7 ). However, BNP added only modest discriminatory value in differentiating these two groups. In patients with advanced HF, BNP levels correlate only modestly with LV filling pressures, and the diagnostic accuracy of BNP for predicting an elevated PCWP is limited by a high false-negative rate. BNP levels decrease in parallel with a reduction in filling pressures during acute diuretic and vasoactive therapy. Change in BNP ( Figure 14-8, A ) and predischarge BNP ( Figure 14-8, B ), as well as in NT-proBNP, are independent predictors of subsequent events. In practice, however, natriuretic peptide levels can vary substantially among individuals with the same degree of HF (see Table 14-4 ). For example, natriuretic peptide levels are higher in women, increase with age and renal dysfunction, and decrease with obesity. Currently, no proven role has been established for natriuretic peptide–guided therapy in the inpatient or outpatient setting, although a BNP level obtained at the time of presentation to the emergency room may decrease resource utilization. Novel biomarkers that include ST2, galectin-3, and neutrophil gelatinase-associated lipocalin are currently under development for use in the diagnosis and treatment of acute decompensated HF.


INCREASED LEVELS | DECREASED LEVELS |
---|---|
Older age | Overweight and obesity |
Female sex Acute or chronic kidney disease Non–heart failure conditions Myocardial infarction Pulmonary embolism Cor pulmonale | Heart failure with preserved ejection fraction |
Pharmacologic therapy | |
Angiotensin receptor blockers | |
β-Blockers | |
Spironolactone | |
Device therapy | |
Cardiac resynchronization therapy | |
Ventricular assist device |
Noninvasive Versus Invasive Management
Although practice patterns differ greatly, the majority of patients with acute decompensated HF can be managed safely and effectively on a cardiac telemetry unit without invasive monitoring. Admission to an intensive care unit should be reserved for patients with 1) hemodynamic instability that requires titration of vasoactive therapy, placement of a PAC, or mechanical circulatory support; 2) unstable cardiac rhythms that require cardioversion, defibrillation, or temporary pacing; 3) acute respiratory failure that requires noninvasive or mechanical ventilation; or 4) severe renal impairment that requires electrolyte management and urgent consideration of dialysis. For all patients, clinical goals of therapy should be set ( Table 14-5 ), including the relief of congestion-related symptoms, such as orthopnea and abdominal fullness, and improvement in symptoms of low cardiac output (fatigue, anorexia) if present. Physical findings of volume overload—including jugular venous distension, lower extremity edema, hepatomegaly, and ascites—should also be targets of therapy. Despite optimal care, not all patients will be free of congestion at the time of discharge, including patients in whom diuresis is limited by worsening renal function, hypotension, or both. If possible, the patient should be discharged with a systolic blood pressure above 80 mm Hg and warm extremities.
GOAL | CLINICAL ENDPOINT | HEMODYNAMIC ENDPOINT |
---|---|---|
Decrease left-sided filling pressure | Absence of orthopnea, dyspnea on minimal exertion | PCWP ≤16 mm Hg |
Decrease right-sided filling pressure | Absence of gastrointestinal symptoms Resolution of edema or hepatomegaly JVP <8 cm H 2 O | RAP ≤8 mm Hg |
Decrease peripheral resistance | Warm extremities | SVR 1000-1200 dynes/sec/cm –5 |
Maintain adequate perfusion pressure | SBP ≥80 mm Hg Stable (or improved) renal function | SBP ≥80 mm Hg |
Increase cardiac output | Proportional pulse pressure >25% | CI ≥2.2 L/min/m 2 |
Restore normal oxygenation | Oxygen saturation on room air >90% | NA |
The insertion of a PAC for the purpose of hemodynamic monitoring may be helpful in selected patients but is not recommended for the routine management of acute decompensated HF. As described above, a reasonable noninvasive assessment of volume status and adequacy of perfusion may be obtained by history and physical exam. If severely elevated filling pressures or critically reduced perfusion are evident, placement of a PAC should not take precedence over initiation of appropriate therapy, such as parenteral diuretics, positive inotropic drugs, or vasopressor agents. In selected cases, hemodynamic measurements will be helpful in the choice and titration of parenteral agents to optimize loading conditions and improve clinical status rapidly. However, routine clinical use of the PAC for HF management has not proven effective in observational or controlled clinical studies.
In an observational study of critically ill patients with a variety of conditions—including acute respiratory failure, multisystem organ failure, and HF—right-heart catheterization was associated with an increased 30-day mortality and increased utilization of resources. However, this study was methodologically flawed; control for the collection, interpretation, and use of hemodynamic data was lacking, and post hoc matching was used. In the subgroup of patients with HF, the relative hazard of death was 1.02 ( P = .94).
The ESCAPE trial sought to determine whether PAC use is safe and whether it improves clinical outcomes in patients hospitalized with severe HF. In this study, 433 patients were randomized to receive therapy guided by clinical assessment and PAC or by clinical assessment alone. The target of therapy in both groups was resolution of congestion, with additional hemodynamic targets (right atrial pressure 8 mm Hg, PCWP 15 mm Hg) set for the invasive group. Therapy in both groups led to substantial reduction in signs and symptoms of congestion, but no difference in morbidity or mortality was observed in the two groups ( Table 14-6 ). In a meta-analysis of randomized clinical trials in critically ill patients that included data from ESCAPE, use of the PAC had no effect on hospital length of stay or mortality.
VARIABLE | PAC GROUP | CLIN GROUP | P VALUE |
---|---|---|---|
Days alive out of hospital (mean) | 133 | 135 | .99 |
Mortality at 180 days (%) | 20.0 | 17.4 | .95 |
Hospitalizations per patient (median) | 2.0 | 2.0 | NA |
Δ Global symptom score | 25 ± 25 | 24 ± 24 | .45 |
Weight loss (kg) | 4.0 ± 5.4 | 3.4 ± 4.2 | .32 |
Δ Creatinine (mg/dL) | 0.0 ± 0.4 | 0.1 ± 0.1 | .02 |
Line infection (%) | 1.9 | 0 | .03 |
Despite these negative findings, there may be selected indications for invasive hemodynamic monitoring during treatment of acute decompensated HF, especially in patients who are refractory to initial therapy or in those whose volume status is uncertain. These include evidence of severe hypoperfusion; marked congestion at rest, associated with acute ischemia, infarction, or renal failure; fluid retention refractory to high-dose or combination diuretics; hypoxemia with coexistent cardiac and pulmonary disease; and shock of unclear etiology. In addition, hemodynamic assessment is routine in considering patients for cardiac transplantation; and the degree and reversibility of pulmonary hypertension are important predictors of posttransplant outcomes. Similarly, invasive hemodynamic measurements are helpful in the evaluation of patients for VAD implantation, either as a bridge to cardiac transplant or as permanent therapy, and in their perioperative management (see Chapter 15 ). In patients with end-stage HF being considered for home inotropic support, documentation of an adequate hemodynamic response to the inotropic agent is recommended. The benefits of PAC use may be more evident at centers experienced with hemodynamic monitoring for advanced HF.
Hemodynamic Profiles
Initial clinical assessment of cardiac filling pressures and systemic perfusion can be used to categorize patients into broad hemodynamic profiles that help guide medical management and provide prognostic information. If the patient is found to be clinically well-compensated despite presenting with shortness of breath at rest, an evaluation for intermittent ischemia or paroxysmal arrhythmias is indicated. In addition, noncardiac causes of dyspnea, both pulmonary and extrapulmonary, as well as psychologic causes such as anxiety should be considered.
The most common hemodynamic profile encountered in patients with acute decompensated HF is pulmonary and/or systemic venous congestion with normal perfusion . In these patients, symptoms will generally respond to diuresis using an intravenous (IV) loop diuretic or the combination of a loop and thiazide diuretic. If the patient does not respond to initial attempts at diuresis, vasodilator therapy—either oral or IV—may require titration, as elevated filling pressures can be exacerbated by systemic and pulmonary vasoconstriction in addition to volume expansion. If the patient is already taking an ACE inhibitor or angiotensin receptor blocker (ARB), the dose should be titrated after an effective diuresis has been achieved, while monitoring closely for orthostatic hypotension and worsening renal function. In selected patients, a combination of vasodilators may be used, such as isosorbide dinitrate, hydralazine, or an ARB in addition to an ACE inhibitor. Large pleural effusions or ascites that do not respond to diuresis may require thoracentesis or paracentesis, respectively. Symptomatic goals of diuretic therapy include resolution of orthopnea and dyspnea at rest or on minimal exertion. Although symptoms of congestion often improve significantly within the first 12 to 24 hours of therapy, several additional days of hospitalization are often necessary to achieve optimal volume status. For patients in whom a PAC has been placed, hemodynamic goals are discussed below.
Hypoperfusion without pulmonary venous congestion , manifested by progressive fatigue and anorexia and often accompanied by azotemia, may precipitate hospitalization in patients with severe HF. Use of positive inotropic agents is often necessary when perfusion is critically limited, although open-label and controlled studies, as well as registry data, suggest that short-term exposure to an inotrope is associated with an increased risk of adverse events, including death. Use of parenteral vasodilators may be considered, if systemic vasoconstriction is suspected, but these are usually poorly tolerated if filling pressures are not also elevated. They can precipitate hypotension and lead to worsening renal function in the setting of low or normal cardiac filling pressures. In addition, further reflex neurohormonal activation can lead to clinically significant arrhythmias. In patients with underlying ischemic heart disease, decreased coronary artery perfusion pressure and reflex tachycardia may aggravate ischemia. Finally, some patients may develop significant hypoperfusion secondary to overdiuresis. In this situation, the judicious use of IV fluids may be considered, but patients should be observed closely to avoid the onset of congestive symptoms.
For patients who come to medical attention with a combination of pulmonary and/or systemic venous congestion and inadequate systemic perfusion , simultaneous reduction in filling pressures and optimization of systemic vascular resistance (SVR; see Figure 14-4, B ) can generally be accomplished with IV vasodilator therapy, with intermittent or continuous infusion diuretics, over 1 to 3 days. Once clinical goals are achieved, conversion to an oral regimen that maintains stability of clinical and laboratory parameters may require an additional 24 to 48 hours of treatment.
Hemodynamic Goals of Therapy
When a PAC has been placed to guide treatment, the ideal goal of parenteral therapy in the management of acute decompensated HF is to achieve an effective cardiac output at relatively normal filling pressures. For patients with severe hemodynamic compromise, a reduction in filling pressures and SVR is often associated with a 30% to 50% increase in forward stroke volume. Although the optimal hemodynamics for a given patient cannot be predicted with certainty, specific goals of hemodynamically guided therapy should be set (see Table 14-5 ). A PCWP less than or equal to 16 mm Hg is appropriate for most patients with chronic HF, whereas a slightly higher filling pressure may be necessary to maintain cardiac output in the setting of acute MI (i.e., decreased LV compliance without significant dilation). A right atrial pressure less than or equal to 8 mm Hg is optimal in most settings, as long as the PCWP is not reduced excessively. With exceptions such as RV infarction and pulmonary embolism associated with hypotension, a higher right atrial pressure may be required. Maintaining a cardiac index greater than or equal to 2.2 L/min/m 2 is often necessary to avoid cerebral, renal, or hepatic hypoperfusion. However, lower cardiac indices may cause relatively little organ dysfunction, if the low-output state has developed gradually. SVR is optimal in the range of 1000 to 1200 dynes/sec/cm –5 . Although cardiac output may be increased further with additional lowering of the SVR, in the range of 800 to 900 dynes/sec/cm –5 , a reflex increase in sympathetic tone may result in unwanted tachycardia, orthostatic hypotension, and renal dysfunction. Finally, the above goals should be aimed for while maintaining a systolic blood pressure of 80 mm Hg or greater and a mean arterial pressure of 60 mm Hg or greater.
It should be remembered that thermodilution measurements may overestimate the cardiac output in patients with low-output states, possibly because of a loss of the cold indicator across the myocardium. Thermodilution measurements may also be unreliable in the setting of significant tricuspid regurgitation or marked respiratory variation, although one study showed a good correlation between thermodilution and Fick outputs in patients with chronic HF and moderate to severe tricuspid regurgitation. Alternatively, mixed venous oxygen saturations may be used to calculate cardiac output using the Fick equation with oxygen consumption measured by a metabolic rate meter. Given that oxygen consumption may be influenced markedly by infection, other stresses, or sedation and that it has been shown to vary greatly among adults at the time of cardiac catheterization, the use of “assumed” Fick outputs are not recommended. An alternative is to follow changes in the mixed venous oxygen saturation to estimate cardiac output trends. For patients who require hemodynamic monitoring, but with a contraindication to PAC placement (e.g., poor venous access), several minimally invasive devices that measure cardiac output are now available, although most have been used to manage postoperative cardiac surgical patients, and none has been shown to improve outcomes in acute decompensated HF.
In choosing an initial parenteral agent, particular attention should be paid to the SVR (see the following discussion on specific agents). Patients with an elevated SVR will generally tolerate initiation of a vasodilator, such as nitroglycerin or nesiritide, without hypotension and will achieve significant hemodynamic benefit. On the other hand, patients with a low SVR, such as that secondary to concomitant sepsis or anesthesia, often will not tolerate further vasodilation without developing symptomatic hypotension. Dobutamine, which provides inotropic support in addition to mild vasodilation, is the preferred initial agent in this situation. An alternative choice would be dopamine at lower doses (3 to 5 µg/kg/min). Higher doses may increase filling pressures and elevate the SVR by stimulating α-adrenergic receptors. In patients with an SVR in the normal range, both vasodilators and positive inotropes are often effective and may be used in combination. An alternative to the combination of a vasodilator and inotrope is the phosphodiesterase (PDE) inhibitor milrinone, which exerts both direct inotropic and vasodilator effects in patients with acute decompensated HF. The incidence of hypotension is higher with milrinone as a result of greater peripheral vasodilation.
Fluid Management
Parenteral Diuretic Therapy
The initiation of an effective diuresis is integral to the management of elevated cardiac filling pressures associated with acute decompensated HF. For patients admitted to the hospital, this can best be accomplished with parenteral diuretics, as the IV route will more reliably deliver high drug concentrations to the renal tubular lumen. Furosemide is the most widely used loop diuretic, although bumetanide and torsemide are also available for patients with documented intolerance or unresponsiveness to furosemide. Furosemide can be given intravenously in doses ranging from 20 to 240 mg or higher depending on the patient’s diuretic history and as frequently as every 4 to 6 hours. If patients are already receiving loop diuretics, the initial IV dose should equal or exceed the chronic oral dose (ACC/AHA class I recommendation, level of evidence C). Addition of a thiazide-type diuretic, such as metolazone (2.5 to 10 mg orally) or chlorothiazide (250 to 500 mg IV), often potentiates the diuresis. A continuous infusion of loop diuretic (e.g., furosemide 5 to 40 mg/h) may be used to treat refractory fluid retention, but the benefit of this approach as an initial diuretic strategy remains unproven.
A Cochrane review of eight trials in 254 patients found that urine output was significantly greater with a continuous infusion, although the difference was modest (mean difference, 271 mL/day), and less tinnitus and hearing loss occurred with continuous infusion. The Diuretic Optimization Strategies in Acute Heart Failure (DOSE-AHF) study used a 2 × 2 factorial design and randomly assigned 308 patients within 24 hours of HF admission to receive high-dose versus low-dose and continuous versus bolus IV diuretics. No differences were observed in symptom relief ( Figure 14-9, A ), renal function, net fluid loss, or death and rehospitalization at 60 days ( Figure 14-9, B ) among patients receiving bolus versus continuous-infusion diuretics. Although the higher dose strategy was associated with greater diuresis and more favorable outcomes in some secondary measures, including weight loss and relief from dyspnea, it was also associated with transient worsening renal function. Although not demonstrated in DOSE-AHF, transient worsening renal function during treatment of acute decompensated HF may actually be associated with improved outcomes, when it is related to aggressive diuresis.

With regard to timing of the initiation of IV diuretics, the ACC/AHA guidelines strongly recommend that treatment begin in the emergency department or outpatient clinic, as early intervention may be associated with better outcomes. In an analysis from the ADHERE registry, delay in initiation of IV diuretics was associated with less symptom relief at discharge and a modest increased risk of in-hospital mortality, independent of other prognostic variables. The addition of hypertonic saline to IV furosemide may result in more rapid achievement of dry weight, but this novel strategy remains to be tested in a multicenter study.
For patients with hypokalemia that requires significant potassium supplementation, a potassium-sparing diuretic, such as spironolactone or triamterene, may be used; however, serum potassium levels should be followed closely to avoid the development of hyperkalemia, which can be precipitated by worsening renal function. Aldosterone receptor antagonists are additionally beneficial in the management of fluid retention associated with cirrhosis, and chronic therapy with spironolactone or eplerenone reduces morbidity and mortality in patients with New York Hospital Association (NYHA) class II to IV heart failure or LV dysfunction complicating acute MI (see Chapter 12 ). For patients with marked fluid overload unresponsive to diuretics, or for those who develop worsening renal function that requires discontinuation of diuretics, ultrafiltration or hemodialysis may be considered if there is coexistent renal failure; this will be discussed later in this chapter.
In the setting of acute pulmonary edema, the rapid clearance of congestion by parenteral diuretics is mediated by natriuresis and diuresis, decreased intravascular volume, and systemic vasodilation. In patients with acute decompensated HF, the goals of diuretic therapy include the elimination of edema and orthopnea (see Table 14-5 ). Reduction in atrial and ventricular diastolic pressures reduces wall stress and ventricular volumes and may improve subendocardial perfusion and reduce valvular regurgitation, respectively. Frequent reevaluation of clinical status and renal function, as well as hemodynamics if available, is necessary to guide frequency and duration of therapy. Mild worsening of renal function may be tolerated in order to achieve hemodynamic compensation, and diuretic-induced hemoconcentration may be a marker for improved outcomes in this group of patients. On the other hand, persistent worsening renal function may identify high-risk patients who require closer follow-up after discharge. Fluid and sodium restriction and cautious replacement of potassium and magnesium are important adjunctive measures that should not be overlooked. Once treatment goals are achieved, the patient should be converted to an oral diuretic regimen that maintains stable weight and renal function. Adverse effects of aggressive diuresis also include electrolyte abnormalities and associated arrhythmias, metabolic alkalosis, muscle cramps, and ototoxicity.
Ultrafiltration
Ultrafiltration removes excess plasma volume without causing a significant change in electrolytes. In patients with chronic HF and diuretic resistance, ultrafiltration may improve symptoms, neurohormones, and hemodynamics without hypotension. Reductions in peripheral and pulmonary edema and a subsequent increase in diuretic efficacy have been reported. Recent pilot studies in acute decompensated HF suggest that early ultrafiltration is associated with greater fluid removal and symptom relief than medical therapy alone and may decrease length of stay and readmission rates. The Ultrafiltration versus Intravenous Diuretics for Patients Hospitalized for Acute Decompensated Congestive Heart Failure (UNLOAD) trial randomized 200 patients within 24 hours of hospitalization for HF to ultrafiltration or IV diuretics. At 48 hours, weight loss (5.0 vs. 3.1 kg; P = .001) and net fluid loss (4.6 vs. 3.3 L; P = .001) were greater in the ultrafiltration group. At 90 days, ultrafiltration had reduced the risk of rehospitalization for HF compared with IV diuretics ( P = .037), but no differences were found between the two groups in symptoms, quality of life, 6-minute walk test, BNP levels, renal function, or hospital length of stay. It has been hypothesized that greater sodium removal during ultrafiltration (hypotonic plasma water) compared with furosemide (hypotonic urine) explains a sustained improvement. The Heart Failure Society of America (HFSA) guidelines acknowledge the apparent effectiveness of ultrafiltration but raise concerns about safety, cost, need for venous access, and nursing support. The ACC/AHA guidelines suggest consultation with a renal specialist before opting for any mechanical strategy to effect volume removal.
The effects of ultrafiltration on renal function and outcomes in patients with acute decompensated HF and cardiorenal syndrome are currently being tested in the multicenter randomized Cardiorenal Rescue Study in Acute Decompensated Heart Failure (CARRESS) trial sponsored by the National Institutes of Health (NIH). In this study, 200 patients with worsening renal function at the time of admission or during the first 7 days of inpatient therapy, defined as an increase in serum creatinine by at least 0.3 mg/dL, are being randomized to stepped pharmacologic care versus ultrafiltration. The change in serum creatinine and weight together as a “bivariate” endpoint will be assessed at 96 hours after enrollment.
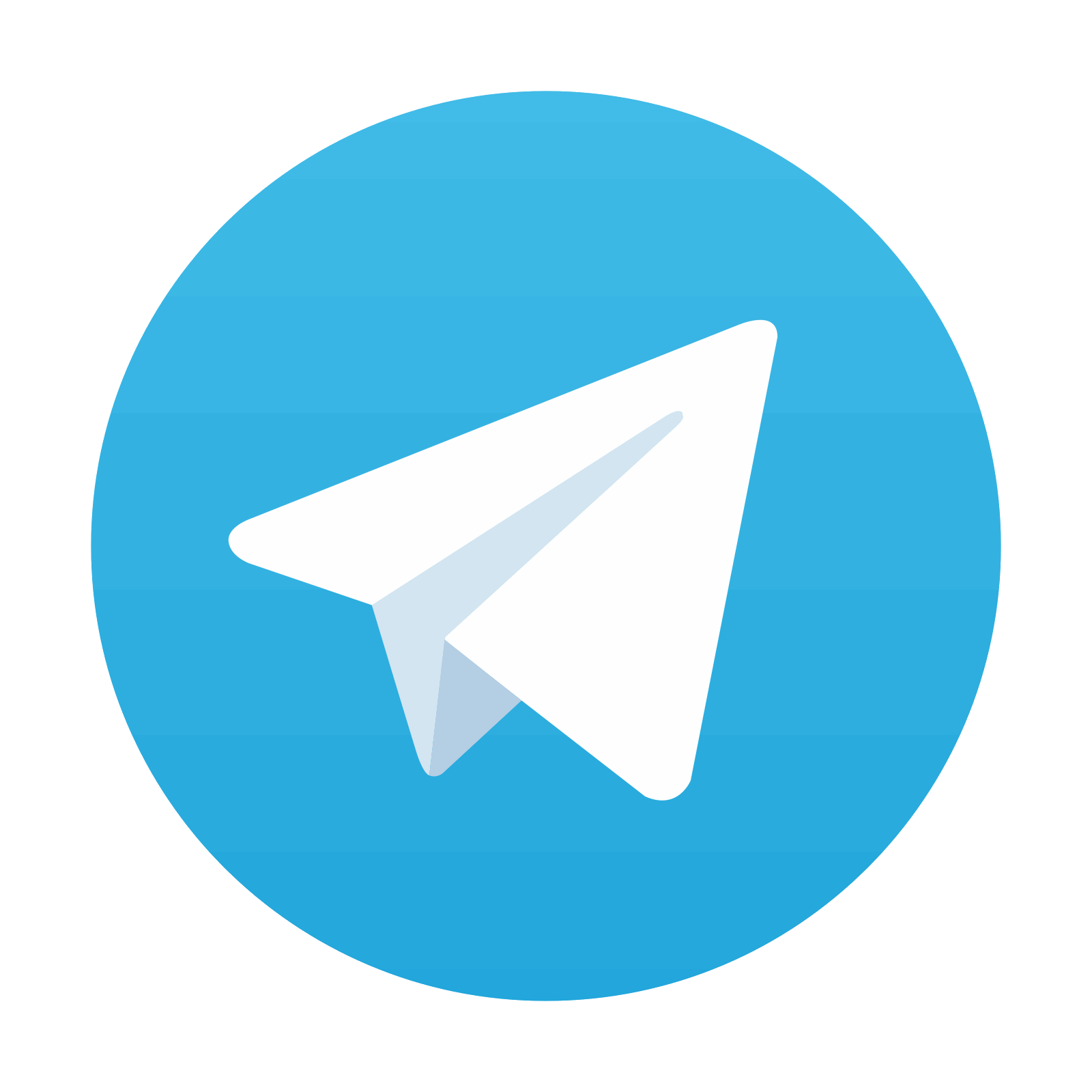
Stay updated, free articles. Join our Telegram channel

Full access? Get Clinical Tree
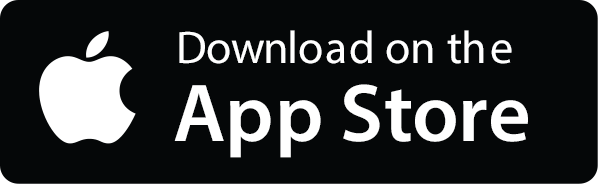
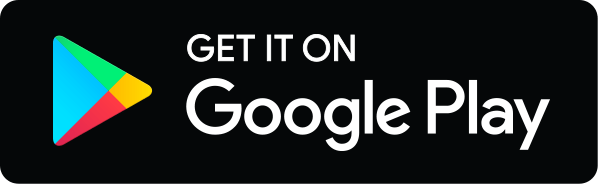