Right ventricular (RV) function after ST-segment elevation myocardial infarction (STEMI) has important prognostic implications. However, the changes in RV function over time after STEMI and the incidence of RV remodeling remain unknown. The present study evaluated changes in RV dimensions and function in contemporary patients with first STEMI and assessed the independent determinants of RV dysfunction at follow-up. Patients with first STEMI (n = 940, 60 ± 11 years, 77% men) treated with primary percutaneous coronary intervention underwent echocardiography at baseline and 6- and 12-month follow-up. The prevalence of RV dysfunction (tricuspid annular plane systolic excursion [TAPSE] ≤15 mm) decreased significantly at 6 months follow-up (from 15% to 8%, p <0.001) and the incidence of RV remodeling (increase in RV end-diastolic area [RVEDA] ≥20%) was observed in 200 patients (25%). Absolute changes in RVEDA were independently associated with absolute changes in wall motion score index and left ventricular (LV) remodeling (p <0.001 for both parameters), whereas absolute changes in TAPSE were independently related with absolute changes in wall motion score index and mitral regurgitation grade (p <0.001 for both parameters). Independent correlates of RV dysfunction at 6 months follow-up were multivessel coronary disease (odds ratio [OR] 2.13), peak cardiac troponin T (OR 1.05), angiotensin-converting enzyme inhibitors and/or angiotensin receptor blockers use (OR 0.27), baseline LV ejection fraction (OR 0.96) and baseline TAPSE (OR 0.88). In conclusion, despite the non-negligible incidence of RV remodeling in patients with first STEMI, RV function improves early after STEMI. Multivessel coronary disease, infarct size, baseline LV ejection fraction and TAPSE and the nonuse of angiotensin-converting enzyme inhibitors and/or angiotensin receptor blockers are independent determinants of RV dysfunction.
Right ventricular (RV) dysfunction portends a poor prognosis in patients after acute myocardial infarction. However, RV function may recover within days or weeks, especially after successful reperfusion. Changes in RV dimensions and function after ST-segment elevation myocardial infarction (STEMI) have received less attention than the left ventricle despite the possibility of RV remodeling and dysfunction after STEMI. The incidence of RV dysfunction in patients with STEMI may vary considerably depending on the imaging technique and definition used. Furthermore, the time course of RV remodeling and RV dysfunction development and the factors influencing these processes after STEMI remain unexplored in the era of primary percutaneous coronary intervention (PCI). Therefore, the aims of the present evaluation were to describe the changes in RV dimensions and function over time after STEMI in a contemporary cohort of patients treated with primary PCI according to current guidelines and to investigate the independent correlates of RV dysfunction at follow-up.
Methods
Patients admitted with a STEMI at the Leiden University Medical Center were included in an ongoing registry (MISSION!). All patients were treated with primary PCI according to the institutional protocol, which is based upon the recent European Society of Cardiology and American College of Cardiology and/or American Heart Association guidelines. In brief, this protocol includes optimal medical therapy initiated early during hospitalization, 2-dimensional echocardiography performed within 48 hours of admission, and standardized outpatient clinical follow-up at 6 and 12 months. Two-dimensional echocardiography was repeated at each outpatient visit. In the present evaluation, patients with a first STEMI with available echocardiograms at baseline and 6- and 12-month follow-up were included. Patients with previous revascularization (PCI and/or coronary artery bypass grafting) were excluded. Other exclusion criteria were clinical signs of heart failure at admission (Killip class III or IV), out-of-hospital cardiac arrest, pulmonary disease, lobectomy, and congenital heart disease. Clinical and echocardiographic data were collected at the departmental Cardiology Information System (EPD-Vision, Leiden University Medical Center, Leiden, The Netherlands) and the echocardiography database, respectively, and these were retrospectively analyzed. For this retrospective analysis of clinically acquired data, the institutional review board waived the need of patient written informed consent.
Echocardiographic images were acquired with the patient at rest in the left lateral decubitus position using a commercially available system (Vivid 7 and Vivid E9, General Electric-Vingmed, Horten, Norway). Data acquisition was performed with 3.5 MHz and M5S transducers in the standard parasternal and apical views. Standard M-mode, 2-dimensional, color, pulse-wave and continuous-wave Doppler images were obtained during breath hold and saved in cine-loop format. Data analysis was performed offline with commercially available postprocessing data software (EchoPac 112.0.1, General Electric Medical Systems, Horten, Norway).
LV function was assessed by measuring the LV end-systolic and end-diastolic volumes in the apical 4- and 2-chamber views, calculating the LV ejection fraction (LVEF) using the biplane Simpson’s method. The wall motion score index (WMSI) was calculated as the sum of the segment scores divided by the number of segments scored. Severity of mitral regurgitation was semiquantitatively assessed from the jet area of color flow Doppler images and the width of the vena contracta. LV diastolic function was evaluated by positioning the pulse-wave Doppler sample volume between the tips of the mitral leaflets and obtaining the spectral signal of the mitral valve inflow. Peak early (E) and late (A) diastolic velocities and E-wave deceleration time were measured. E′ was assessed with tissue Doppler imaging at the septal side of the mitral annulus in the apical 4-chamber view and E/E′ ratio was calculated.
RV dimensions were assessed by measuring RV end-diastolic area and end-systolic area in the apical 4-chamber view. RV remodeling was defined as an increase in RV end-diastolic area ≥20% from baseline to 6 months follow-up. According to current recommendations, RV function was evaluated by measuring the tricuspid annular plane systolic excursion (TAPSE) in the apical 4-chamber view using M-mode. RV dysfunction was defined as TAPSE ≤15 mm. Right atrial area was traced in the apical 4-chamber view at the end-systole. Severity of tricuspid regurgitation was evaluated based on ratio of the regurgitant jet and right atrial area. Systolic pulmonary artery pressure was estimated by adding RV pressure to right atrial pressure. RV pressure was estimated by calculating the systolic pressure gradient between the RV and right atrium by the maximum velocity of the regurgitant jet using the modified Bernoulli equation. Right atrial pressure was estimated by measuring the diameter and the inspiratory collapse of the inferior vena cava.
Continuous data are reported as mean ± SD or standard error and categorical data as frequencies and percentages. Absolute changes in RV dimensions and function during follow-up (from baseline to 6 and 12 months follow-up) were evaluated using linear mixed model analyses. Associations between absolute changes in RV dimensions and function and absolute changes in other echocardiographic parameters were assessed with linear mixed models, correcting for potential confounders. RV dysfunction (TAPSE ≤15 mm) was assessed at baseline and at 6 months follow-up. Changes in prevalence of RV dysfunction at baseline and at 6 months follow-up were analyzed with the McNemar’s test. Baseline clinical and echocardiographic differences between patients with and without RV dysfunction at 6 months follow-up were analyzed using the Student t test and chi-squared test. Univariate and multivariate logistic regression analyses were performed to identify independent baseline clinical and echocardiographic correlates of RV dysfunction at 6 months follow-up. All clinically relevant variables were included in a multivariate logistic regression model. LV end-diastolic volume, LV end-systolic volume, and LVEF were not included in the same model to avoid collinearity.
A 2-sided p value <0.05 was considered statistically significant. All statistical analyses were performed with SPSS software (version 20.0, SPSS Inc., Chicago, Illinois).
Results
A total of 940 patients (mean age 60 ± 11 years, 77% men) with a first STEMI treated with primary PCI and with complete echocardiographic data at baseline and 6- and 12-month follow-up were eligible for the present study ( Figure 1 ). The culprit vessel was the left anterior descending coronary artery in 47% of the patients, the right coronary artery in 38%, and the circumflex artery in 15%. Mean peak cardiac enzymes were 2,158 ± 3,029 U/L for creatine phosphokinase and 5.5 ± 5.4 μg/L for cardiac troponin T, respectively. At baseline, mean LV end-systolic and end-diastolic volumes, and LVEF were 56 ± 20 mL, 105 ± 32 mL, and 47 ± 8%, respectively. Mean WMSI was 1.45 ± 0.28 and moderate to severe mitral regurgitation was observed in 55 patients (6%). Mean RV end-diastolic and end-systolic areas were 18.3 ± 4.4 cm 2 and 10.5 ± 3.0 cm 2 , respectively. Baseline TAPSE was 19 ± 3.3 mm and moderate to severe tricuspid regurgitation was observed in 46 patients (5%).
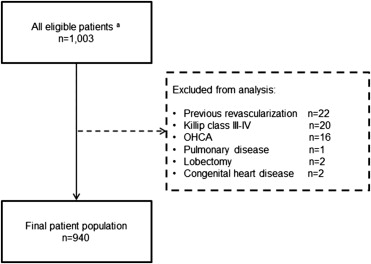
Table 1 lists the absolute changes in RV dimensions and function over time, from baseline to 6 and 12 months follow-up after STEMI. During the 12 months of follow-up, RV end-diastolic area increased modestly but significantly ( Figure 2 ). Based on the upper limit of normality of RV end-diastolic area (<19.0 cm 2 ), patients demonstrated on average dilated RVs at 6 and 12 months follow-up. In contrast, RV end-systolic area did not change over time (p = 0.07). RV remodeling (increase in RV end-diastolic area ≥20%) at 6 months follow-up was observed in 200 patients (25%).
Baseline | 6 months | 12 months | p ∗ | |
---|---|---|---|---|
Right ventricular end-diastolic area (cm 2 ) | 18.4 ± 0.2 | 19.2 ± 0.2 † | 19.0 ± 0.1 † | <0.001 |
Right ventricular end-systolic area (cm 2 ) | 10.5 ± 0.1 | 10.6 ± 0.1 | 10.5 ± 0.1 | 0.07 |
Tricuspid annular plane systolic excursion (mm) | 19 ± 0.1 | 20 ± 0.1 † | 20 ± 0.1 † | <0.001 |
Right atrial area (cm 2 ) | 12.9 ± 0.1 | 14.1 ± 0.1 † | 13.8 ± 0.1 † | <0.001 |
Tricuspid regurgitation grade | 0.52 ± 0.02 | 0.58 ± 0.02 † | 0.58 ± 0.02 † | 0.005 |
Estimated systolic pulmonary arterial pressure (mm Hg) | 24.6 ± 0.3 | 23.9 ± 0.3 | 24.2 ± 0.3 | 0.09 |
∗ p Values are given for the overall change over time.
† p <0.05 compared with baseline (adjusted for multiple comparisons).
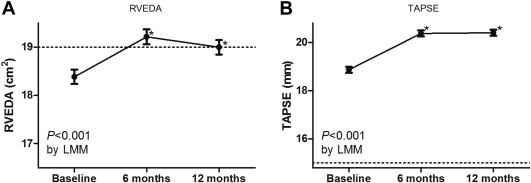
Significant univariate correlates of absolute change in RV end-diastolic area are listed in Table 2 . On multivariate analysis, absolute changes in LV end-diastolic volume and WMSI were independent correlates of absolute change in RV end-diastolic area. Of note, right coronary artery as culprit vessel was not significantly associated with absolute change in RV end-diastolic volume in the univariate analysis (p = 0.21).
Univariate analysis | Multivariate analysis ∗ | |||||
---|---|---|---|---|---|---|
β | 95% Confidence interval | p | β | 95% Confidence interval | p | |
Right ventricular end-diastolic area | ||||||
Change in LVESV (mL) | 0.030 | 0.023 to 0.037 | <0.001 | |||
Change in LVEDV (mL) | 0.026 | 0.021 to 0.030 | <0.001 | 0.028 | 0.023 to 0.032 | <0.001 |
Change in LVEF (%) | 0.0037 | −0.014 to 0.022 | 0.68 | |||
Change in WMSI | −1.09 | −0.53 to −1.66 | <0.001 | −1.51 | −0.95 to −2.06 | <0.001 |
Change in E/E′ ratio | −0.0018 | −0.026 to 0.022 | 0.88 | |||
Change in MR grade | 0.15 | −0.10 to 0.39 | 0.25 | |||
Tricuspid annular plane systolic excursion | ||||||
Change in LVESV (mL) | −0.0076 | −0.0014 to −0.014 | 0.016 | |||
Change in LVEDV (mL) | 0.0034 | −0.00062 to 0.0075 | 0.097 | |||
Change in LVEF (%) | 0.061 | 0.046 to 0.077 | <0.001 | |||
Change in WMSI | −2.01 | −1.53 to −2.49 | <0.001 | −1.89 | −1.42 to −2.37 | <0.001 |
Change in E/E′ ratio | −0.029 | −0.0081 to −0.050 | 0.007 | |||
Change in MR grade | −0.54 | −0.32 to −0.75 | <0.001 | −0.43 | −0.22 to −0.65 | <0.001 |
∗ In the 2 multivariate analyses, only changes in LVEDV and WMSI for change in RVEDA and only changes in WMSI and MR grade for change in TAPSE were included, respectively, as indicated by the bold text.
At 6 months follow-up, TAPSE measurement was feasible in 95% of patients. RV dysfunction at baseline (TAPSE ≤15 mm) was observed in 133 patients (15%) and 73 patients (8%) remained with RV dysfunction at 6 months follow-up (p <0.001 compared with baseline; Figure 2 ). Table 2 provides the significant univariate correlates of absolute change in RV function, measured by TAPSE. On multivariate analysis, absolute change in TAPSE was independently associated with absolute changes in WMSI and mitral regurgitation grade ( Table 2 ). No significant correlation was found between right coronary artery as culprit vessel and absolute change in TAPSE (univariate p = 0.94).
Baseline characteristics of patients with and without RV dysfunction at 6 months follow-up are listed in Table 3 . Patients with RV dysfunction at 6 months follow-up had a lesser prevalence of smoking and a greater prevalence of multivessel coronary disease compared with patients without RV dysfunction. Peak cardiac troponin T levels were higher in patients with RV dysfunction. At discharge, the use of angiotensin-converting enzyme inhibitors (ACEIs) and/or angiotensin receptor blockers (ARBs) was lesser in patients with RV dysfunction at 6 months follow-up. Patients with RV dysfunction showed larger LV end-systolic volumes and poorer LV functions at baseline compared with their counterparts. In patients with RV dysfunction, E/E′ ratio was higher and TAPSE was lower compared with patients without RV dysfunction at 6 months follow-up.
Variable | Right Ventricular Dysfunction | p ∗ | |
---|---|---|---|
Yes n = 73 | No n = 824 | ||
Age (years) | 61 ± 12 | 59 ± 11 | 0.18 |
Men | 57 (78%) | 636 (77%) | 0.86 |
Current smoker | 25 (34%) | 426 (52%) | 0.004 |
Diabetes mellitus | 7 (10%) | 66 (8%) | 0.64 |
Family history of coronary artery disease | 27 (38%) | 377 (46%) | 0.16 |
Hypercholesterolemia | 13 (18%) | 150 (18%) | 0.92 |
Hypertension | 28 (38%) | 258 (31%) | 0.22 |
Left anterior descending artery as culprit coronary artery | 38 (52%) | 377 (46%) | 0.30 |
Right coronary artery as culprit coronary artery | 26 (36%) | 323 (39%) | 0.55 |
Left circumflex as culprit coronary artery | 9 (12%) | 124 (15%) | 0.53 |
Multivessel coronary disease | 47 (64%) | 387 (47%) | 0.004 |
Thrombolysis In Myocardial Infarction 2–3 flow | 72 (99%) | 812 (99%) | 0.98 |
Peak creatine phosphokinase level (U/L) | 2,439 ± 1,965 | 2,142 ± 3,165 | 0.43 |
Peak cardiac troponin T level (μg/L) | 7.6 ± 6.3 | 5.3 ± 5.3 | 0.003 |
Echocardiography | |||
Left ventricular end-systolic volume (mL) | 64 ± 28 | 56 ± 19 | 0.01 |
Left ventricular end-diastolic volume (mL) | 113 ± 44 | 105 ± 31 | 0.15 |
Left ventricular ejection fraction (%) | 43 ± 8 | 47 ± 8 | <0.001 |
Wall motion score index | 1.55 ± 0.29 | 1.44 ± 0.28 | 0.001 |
E/A ratio | 1.01 ± 0.52 | 0.97 ± 0.34 | 0.50 |
Deceleration time (ms) | 199 ± 72 | 209 ± 69 | 0.27 |
E/E′ ratio | 14 ± 6 | 13 ± 6 | 0.02 |
Moderate to severe mitral regurgitation grade | 8 (11%) | 45 (6%) | 0.06 |
Right ventricular end-diastolic area (cm 2 ) | 17.9 ± 5.5 | 18.3 ± 4.3 | 0.42 |
Right ventricular end-systolic area (cm 2 ) | 10.4 ± 3.5 | 10.5 ± 2.9 | 0.93 |
Tricuspid annular plane systolic excursion (mm) | 17 ± 3.3 | 19 ± 3.2 | <0.001 |
Right atrial area (cm 2 ) | 12.7 ± 4.1 | 12.9 ± 3.8 | 0.58 |
Moderate to severe tricuspid regurgitation grade | 3 (4%) | 39 (5%) | 0.80 |
Estimated systolic pulmonary arterial pressure (mm Hg) | 24.6 ± 9.8 | 24.7 ± 8.9 | 0.88 |
Medication at discharge | |||
Angiotensin-converting enzyme-inhibitors and/or angiotensin receptor blockers | 69 (95%) | 810 (98%) | 0.03 |
Antiplatelets | 73 (100%) | 824 (100%) | 1.00 |
Beta-blockers | 69 (95%) | 777 (94%) | 0.94 |
Statins | 72 (99%) | 821 (100%) | 0.22 |
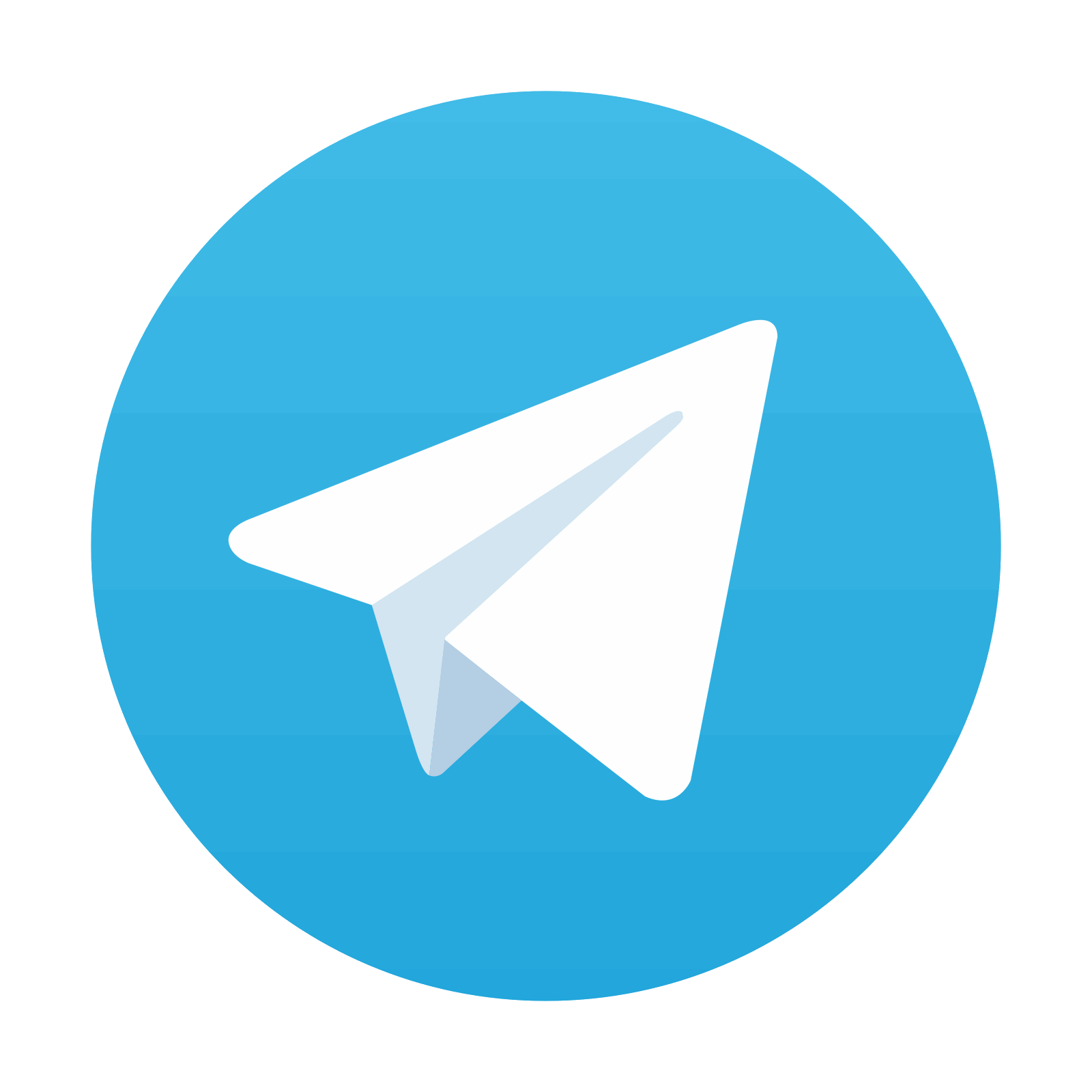
Stay updated, free articles. Join our Telegram channel

Full access? Get Clinical Tree
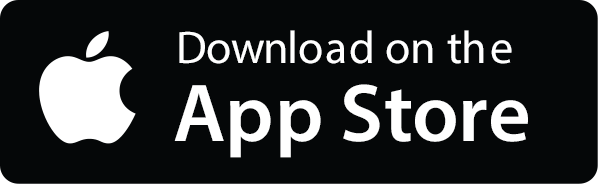
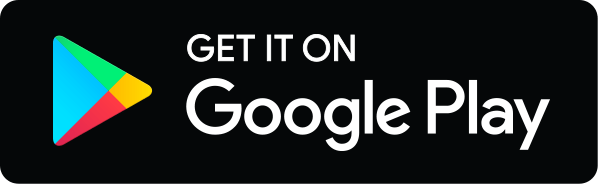
