Introduction
Chronic obstructive pulmonary disease (COPD) is a condition characterized by persistent airflow limitation that is usually progressive and is associated with a chronic enhanced inflammatory response in the airways and lungs to noxious particles and gases. In developed countries, cigarette smoking is the main etiologic factor, outweighing any of the other risk factors. However, in developing countries, the major cause is exposure to biomass fuels. The pathogenesis of COPD is strongly linked to the effects of cigarette smoke on the lungs. There is a general relationship between the extent of the smoking history and the severity of the airflow limitation; however, there is a huge individual variation. Fletcher and colleagues, in an 8-year prospective study of working men in west London, showed that the average decline in FEV 1 in smokers is faster (60 mL/year) than in nonsmokers (30 mL/year). However, smokers who develop COPD have an average decline in FEV 1 of greater than 60 mL/year, although only a proportion of smokers develop clinically significant COPD. It is from these studies that the concept of the “susceptible smoker” developed.
The pathogenesis of COPD is generally thought to involve an abnormal inflammatory response in the lungs to the inhalation of toxic particles and gases, derived from tobacco smoke, air pollution, or occupational exposures. All smokers develop lung inflammation, but this is enhanced and fails to resolve after smoking cessation in those who develop COPD. This suggests that, in smokers who develop COPD, there is abnormal regulation of the inflammatory response in the lungs. The susceptibility factors are still poorly understood and likely involve genetic and epigenetic factors, infections, altered immune regulation, or impaired resolution of inflammation and abnormal repair mechanisms. However, the relationship between the inflammatory responses in the lungs and the accelerated decline in FEV 1 , which characterizes this condition, is far from clear. Moreover, it is now well recognized that COPD is a heterogeneous condition with pathologic changes in the large and small airways (chronic bronchitis and bronchiolitis) and lung parenchyma (emphysema) that vary greatly in their expression among patients. Thus, the mechanisms resulting in these pathogenic changes are also likely to be different.
Pathologic Changes in COPD
Chronic Bronchitis
Chronic bronchitis is defined in clinical terms as the presence of cough and sputum production for most days over 3 months for 2 consecutive years. This clinical definition does not include the presence of airflow limitation. It is thought to result from an innate immune response to inhaled toxic particles and gases, particularly in tobacco smoke. Inflammation is present in the epithelium of the central airways and in the mucus-producing glands in chronic bronchitis. This airway inflammation is associated with increased mucus production, reduced mucociliary clearance, and increased permeability of the airspace epithelial barrier.
The contribution that mucus hypersecretion makes to the airflow limitation in COPD is still uncertain. In the early stages of COPD, its contribution is small because mucus production in smokers with normal lung function does not appear to predict later development of COPD. However, in the later stages of the disease, chronic mucus hypersecretion may accelerate the loss of FEV 1 due to an increased risk of exacerbations. Chronic mucus hypersecretion may result from an inflammatory response in the submucosal glands. Inflammatory cells release serine proteases that are potent secretagogues for mucus. Oxidants derived from cigarette smoke and released from inflammatory leukocytes may also stimulate the overproduction of mucin by induction of the MUC5AC gene.
Emphysema
Emphysema is defined as enlargement of the airspaces distal to the terminal bronchioles, due to destruction of the alveolar walls ( Fig. 43-1 ). Distal airspace enlargement with alveolar destruction reduces maximal expiratory airflow by decreasing the lung elastic recoil. The centrilobular or centriacinar form of emphysema results from dilatation or destruction of the respiratory bronchioles, is the type most closely associated with tobacco smoking, and is thought to be more associated with severe small-airway obstruction. The panlobular or panacinar form of emphysema, which is associated with α 1 -antitrypsin (α 1 -AT) deficiency, results in a more even dilatation and destruction of the entire acinus. Although one or the other of these types may predominate, there is great heterogeneity. The distribution of these types of emphysema is different with an upper lobe predominance common in centrilobular emphysema and lower lobe predominance in panlobular emphysema. The reason for this is not clear and whether different pathogenic mechanisms are involved is also unknown.

There is a relationship between the degree of emphysema and pack-years of smoking, but the relationship is not strong. Around 40% of smokers develop substantial lung destruction from emphysema, and emphysema can be found in some individuals who have normal lung function.
Small-Airway Disease
A major site of airflow limitation in COPD is the small conducting airways (<2 mm in diameter). Niewoehner and coworkers were the first to show that inflammation involving clusters of monocytes and macrophages could be found in the bronchioles of asymptomatic smokers who died of non–smoking-related causes out of hospital. More recent studies have shown that there are abnormalities in small airways in smokers with and without COPD. There is also a relationship between the severity of COPD and the extent of occlusion of the airway lumen by inflammatory mucus exudates. Inflammation and peribronchial fibrosis contribute to the fixed airway obstruction in the small airways in COPD, and chronic inflammation, resulting in the destruction of the alveolar attachments on the outer walls of the small airways, may also contribute.
Pathogenesis of COPD
Pulmonary Circulation
Sustained pulmonary hypertension develops late in the course of COPD, although pathologic changes in the pulmonary vasculature with intimal hyperplasia and muscularization of small pulmonary arteries can develop in mild COPD in heavy smokers with normal lung function ( Fig. 43-2 ). Factors that contribute to pulmonary hypertension include the following:
- ▪
Pulmonary arterial constriction as a result of hypoxia
- ▪
Endothelial dysfunction
- ▪
Remodeling (smooth muscle hypertrophy and hyperplasia) of the pulmonary arteries
- ▪
Destruction of the pulmonary capillary bed

The development of structural changes in the pulmonary arterioles leads to persistent pulmonary hypertension and right ventricular hypertrophy/enlargement and dysfunction (cor pulmonale) (see Chapter 59 ).
A summary of the pathologic changes in the lungs in COPD is given in Table 43-1 .
PROXIMAL AIRWAYS (CARTILAGINOUS, >2-MM DIAMETER) |
|
PERIPHERAL AIRWAYS (NONCARTILAGINOUS, <2-MM DIAMETER) |
|
LUNG PARENCHYMA (RESPIRATORY BRONCHIOLES AND ALVEOLI) |
|
PULMONARY VASCULATURE |
|
An overview of the pathogenic mechanisms in COPD is given in Figure 43-3 .

Inflammation in the Lungs of Smokers Without COPD
Tobacco smoke causes airway inflammatory responses within minutes or hours of exposure. One of the earliest manifestations is a breach in the vascular and airway barrier function, with rapid recruitment of circulating inflammatory cells to the lung. Inflammation is found in the peripheral airways of all smokers even before COPD is established and consists of mononuclear cell infiltrates and clusters of macrophages in the walls of the respiratory bronchioles. These lesions develop initially in the absence of any significant tissue destruction or fibrosis and may be reversible. An inflammatory process involving T lymphocytes and macrophages has also been described in the large airways of smokers with chronic bronchitis. Acute cigarette smoke exposure can result in tissue damage with degradation of extracellular matrix proteins and lipid peroxidation products.
These early inflammatory changes in the airways likely represent the nonspecific innate immune response to airway injury from tobacco smoke. It is unclear why some smokers develop structural abnormalities that eventually lead to clinically detectable COPD, whereas others continue to show an inflammatory infiltrate but maintain otherwise normal airways and lung parenchyma and only mild functional changes that do not become clinically relevant.
Smoking cessation alters the inflammatory response in the lungs in asymptomatic smokers and in patients with COPD. Cross-sectional studies of the effects of smoking cessation show that ex-smokers have less goblet cell hyperplasia and less squamous cell metaplasia in the small, but not the large, airways. Smooth muscle mass in the peripheral and central airways, fibrosis, deposition in the airway wall, fibrosis of the peripheral airways, and the degree of alveolar destruction is not different in asymptomatic ex-smokers and current smokers, nor is the inflammatory response different. There are limited longitudinal studies of the effects of smoking cessation on the inflammatory response in the lungs. Large airway inflammation indicating chronic bronchitis decreased by 3 months and disappeared after 6 months of smoking cessation. Inflammation as assessed in sputum and from bronchial biopsies decreased 1 year after smoking cessation in asymptomatic smokers.
Inflammation in the Lungs in COPD
Studies of lung or bronchial biopsies and induced sputum have shown that lung inflammation is present in all cigarette smokers. However, an enhanced or abnormal inflammatory response to inhaled particles or gases, beyond the normal protective inflammatory response, is a characteristic feature of COPD and has the potential to produce lung injury. Both the innate and adaptive inflammatory and immune responses are involved in the lung inflammation in COPD patients. Recent studies have characterized the inflammation in the lung in COPD in terms of its type, site, degree, and relationship to severity of disease.
Cellular Inflammatory Responses
Bronchial biopsies from smokers with symptoms of chronic bronchitis who have not developed airflow limitation demonstrate that the airway epithelium remains intact; however, the epithelium shows squamous metaplasia and there is an increase in goblet cells. In contrast to asthma, the epithelial reticular basement membrane is not thickened. In patients with mild to moderate COPD, there is an increase in inflammatory cell infiltration in the central airways, compared with nonsmokers or smokers who have not developed the disease. In the bronchial epithelium and submucosa in COPD patients, monocytes are the major cell with scanty neutrophils. Of the monocyte component, T lymphocytes predominate, mainly CD8 + cells (T-cytotoxic lymphocytes) and macrophages (CD68 + cells) ( eTable 43-1 ), in contrast to asthma, in which CD4 + T-helper (T helper 2) lymphocytes predominate. Thus, the ratio of CD8 + /CD4 + increases in COPD. Morphometric analyses of bronchial biopsies show that the ratios of CD8 + /CD4 + T cells are 1.3, 11.8, and 4.3 (mean/mm 3 ) in healthy smokers, stable chronic bronchitis, and exacerbated chronic bronchitis, respectively. CD8 + T cells are also observed in the sputum, bronchial glands, bronchial smooth muscle, and around lymphoid follicles. It has been suggested that the presence of increased CD8 + T lymphocytes differentiates between smokers with and without COPD and that there is a correlation between T-cell numbers, the smoking history, the amount of alveolar destruction, and the severity of airflow limitation. However, smokers with normal lung function also show an increased number of CD8 + cells compared with control nonsmokers.
CD45 | CD3 | PMN | EOS | Mast | CD68 | CD8 | CD4 | |
---|---|---|---|---|---|---|---|---|
Severe COPD | — | ↓ | ↑ | → | → | ↑ | ↓ | → |
Mild/moderate COPD | ↑ | ↑ → | → ↑ | → | → | ↑ → | ↑ → | → |
Control Smokers | → | → ↑ | → | → | → | → | ↑ → | → |
Control Nonsmokers | → | → | → | → | → | → | → | → |
The mechanism by which CD8 + T lymphocytes accumulate in the airways in COPD is not fully understood. There is increased expression of CXCR3 on T cells in the peripheral airways in COPD patients. CXCR3 is a receptor activated by interferon-inducible protein (IP)-10, and the expression of IP-10 itself is increased in bronchiolar epithelial cells. This could contribute to the accumulation of CD8 + cells, which preferentially express CXCR3. Circulating CD8 + cells are also increased in number in COPD patients. These changes suggest chronic immune stimulation. CD8 + cells have a well-recognized role in respiratory viral infections, contributing to viral clearance. It may be that chronic colonization of the lower respiratory tract of COPD patients by bacterial and viral pathogens is responsible for this enhanced inflammatory response. An increase in B lymphocytes and in bronchial-associated lymphoid tissue in small airways also is present as the disease progresses, suggesting an adaptive immune response. It is possible that cigarette smoke damages airway cells, creating new autoantigens that drive the immuno-inflammatory response.
The role of T cells in the pathogenesis of COPD is not fully understood. CD8 + cells have the potential to release tumor necrosis factor (TNF)-α, perforins, and granzymes. CD8 + T lymphocytes isolated from the sputum of smokers are activated and release perforin. In addition, CD8 + cells activate the Fas/Fas ligand apoptotic pathway and there is an association between CD8 + cells and alveolar epithelial cell apoptosis in subjects with emphysema.
Increased numbers of activated neutrophils are found in both bronchoalveolar lavage (BAL) fluid and sputum from patients with COPD. The mechanism of neutrophil passage into the airway lumen in COPD is not entirely clear. Cigarette smoking is known to increase circulating neutrophil counts and to cause sequestration of neutrophils in the lung capillaries by decreasing their deformability. Cigarette smoke also has a direct stimulatory effect on granulocyte production in the bone marrow, possibly mediated by granulocyte macrophage colony–stimulating factor (GM-CSF) and G-CSF released from macrophages. Once sequestered in the pulmonary microcirculation, it is possible that an imbalance between proinflammatory and anti-inflammatory cytokines may result in neutrophil migration into the airspaces. Expression of anti-inflammatory mediators, such as secretory component (SC), club cell (Clara) protein (CC16), and interleukin (IL)-10, are decreased in the airway lumen of smokers with COPD, whereas cytokines, such as IL-8 and monocyte chemotactic protein (MCP)-1, which promote the chemotaxis of neutrophils and monocytes, respectively, and TNF-α, which activates adhesion molecules, are increased. Up-regulation of E-selectin and intercellular adhesion molecule (ICAM)-1 on submucosal vessels and on bronchial epithelium of subjects with COPD suggests that these adhesion molecules may be involved in the recruitment of neutrophils from the circulation and their migration from bronchial subepithelial capillaries into and through the epithelium to enter the airway lumen. The airway epithelium is a rich source of the cytokines/chemokines that recruit both neutrophils and macrophages into the airspaces. Many of these cytokines/chemokines are overexpressed in COPD. IL-6, IL-1β, TNF-α, growth-related gene –α (GRO-α)/ keratinocyte-derived chemokine (KC, CXCL1), MCP-1, and IL-8 are increased in the sputum in COPD patients and the bronchiolar epithelium overexpresses MCP-1, its receptor CCR2, MIP1α, and IL-8.
The role of neutrophils in the pathogenesis of COPD is not entirely clear. Neutrophils can secrete serum proteinases, including neutrophil elastase, cathepsin G, and proteinase 3, as well as matrix metalloproteinase (MMP)-8 and MMP-9. These proteases may contribute to alveolar destruction and are also potent stimuli of mucus secretion. Relationships have been shown between circulating neutrophils and the decline in FEV 1 . Similarly, neutrophil numbers in bronchial biopsy specimens and induced sputum are related to disease severity and the rate of decline in lung function.
There is a 5- to 10-fold increase in the numbers of macrophages in the airways, lung parenchyma, and BAL in patients with COPD. Macrophage numbers in the airways correlate with the severity of the airflow limitation in COPD. Cigarette smoke activates macrophages to release inflammatory mediators, including TNF-α, IL-8, and other CXC chemokines, MCP-1, leukotriene B 4 , and reactive oxygen species (ROS). Macrophages also secrete proteases, including MMP-2, MMP-9, MMP-12, cathepsins K, L, and S, and neutrophil elastase taken up from neutrophils. Macrophages from COPD patients are more activated, secrete more inflammatory proteins, and have greater elastolytic activity when compared with macrophages from normal smokers; macrophage activation is further enhanced by exposure to cigarette smoke. Increased numbers of macrophages in the lungs of COPD patients and smokers may result from increased recruitment of monocytes from the circulation in response to monocyte chemotactic chemokines such as MCP-1, which is increased in sputum and BAL in patients with COPD. CXC chemokines also act as chemoattractants to monocytes. The concentration of CXCL1is markedly increased in sputum and BAL from patients with COPD. Furthermore, monocytes from patients with COPD show a greater chemotactic response to CXCL1 than the monocytes from normal smokers and nonsmokers.
Dendritic cells are present in increased numbers in the airways and alveolar walls of smokers. The role of dendritic cells in COPD is not yet defined, but the cells are likely to have an important role in the innate and adaptive immune responses in COPD.
Cigarette smoke activates airway epithelial cells to produce inflammatory mediators, including TNF-α, IL-1β, GM-CSF, and IL-8. The epithelium in the small airways may be an important source of transforming growth factor (TGF)-β, which may induce local fibrosis. Epithelial cells can also secrete antioxidants and antiproteases and transport immunoglobulin-α and thus may be involved in adaptive immunity. Cigarette smoke may impair these innate and adaptive immune responses of the airway epithelium and increase the likelihood of infection.
The expression of many of the inflammatory mediators implicated in the inflammatory response in the lungs in COPD is controlled by the transcription factor nuclear factor (NF)-κB. NF-κB is up-regulated in alveolar macrophages in patients with COPD and in airway cells in patients with mild/moderate COPD in comparison with control nonsmokers. Up-regulation of NF-κB in lung cells in COPD may be a key molecular mechanism in the ongoing inflammation in the airways. Epigenetic modifications may also contribute to the enhanced inflammation in the lungs in COPD. Histone deacetylase (HDAC) 2 has been shown to be reduced in lung cells of subjects with COPD; a reduction of HDAC would allow increased acetylation of histone residues on DNA, a greater unwinding of DNA, and a greater access of transcription factors such as NF-κB that up-regulate proinflammatory genes.
In general, with increasing severity of COPD there is a further increase in the inflammatory response. There is an increase in the number of neutrophils and macrophages in severe disease and a decrease in T lymphocytes (CD3 + cells) (see eTable 43-1 ). There appears to be a shift in the cellular type in severe disease toward cells with a phagocytic and proteolytic role in the bronchial tissues (see eTables 43-1 to 43-3 ).
CENTRAL AIRWAYS | PERIPHERAL AIRWAYS (<3 MM DIAMETER) | ||||||||||
---|---|---|---|---|---|---|---|---|---|---|---|
PMN | CD68 | CD4 | CD8 | IL-4 | Il-5 | PMN | CD68 | CD4 | CD8 | Total Inflammation | |
Mild/moderate COPD | → | → | → | → | ↓ | → | → | ↑ → | → | ↑ → | ↑ → |
Chronic bronchitis with normal FEV 1 | __ | __ | __ | __ | ↑ | → | __ | __ | __ | __ | ↑ |
Control smokers | → | → | → | → | → | → | → | → | → | → | → |
PMN | CD68 | CD3 | CD4 | CD8 | EOS | |
---|---|---|---|---|---|---|
Mild/moderate COPD | → | → 313 | ↑ | → | ↑ | → |
Smokers with normal FEV 1 | → | ↑ → | ↑ → | → | → | → |
Control Nonsmokers | ↑ → | → | → | → | → | → |
Cytokines and Chemokines
In patients with severe emphysema, lymphocytes in the lungs strongly express TH1 cytokines and secrete high levels of interferon (IFN)-γ, CCR5, and CXCR3. In addition, they show increased expression of CXCR3 ligands, monokine induced by interferon gamma (MIG), and IP-10. This polarization of alveolar lymphocytes toward the TH1 phenotype predominates in severe emphysema.
TNF-α has also been implicated in cigarette smoke–induced emphysema. Increased levels of TNF-α are present in the airways in cigarette smokers. Animals that overexpress TNF-α show evidence of emphysema and an exaggerated alveolar inflammatory response, while TNF receptor knockout mice demonstrate significant protection against cigarette smoke–induced emphysema. With respect to emphysema, TNF-α stimulates MMP synthesis by alveolar macrophages. Cultured macrophages exposed to cigarette smoke extract release TNF-α and, in addition, circulating TNF-α soluble receptors p55 and p75 are significantly increased in COPD patients compared with healthy controls. In animal studies, absence of TNF-α receptor type 2 was associated with reduced inflammatory responses in terms of neutrophil, macrophage, CD4 and CD8 cell influx, and protection against cigarette smoke–induced emphysema.
IL-1β may also play a role in the development of emphysema. In animal models, lung-specific induction of human IL-1β resulted in emphysema, whereas inhibition with IL-1β antibody reduced alveolar macrophage influx into the airspaces following cigarette smoke exposure. Furthermore, double IL-1 receptor and TNF-α receptor knockout mice are protected against elastase-induced emphysema.
Inflammation, Airway Remodeling, and Airflow Limitation
The peripheral airways (bronchioles < 2 mm in diameter) are the major site of increased resistance to airflow in COPD. The main pathologic lesions in the peripheral airways include increased number of inflammatory cells (see Table 43-1 and eTable 43-2 ) and structural changes, such as epithelial goblet cell metaplasia, airway wall fibrosis, and smooth muscle hypertrophy. The increase in the thickness of the airway wall, inflammation, fibrosis, and smooth muscle hypertrophy will encroach on the lumen and reduce airway diameter; increased wall thickness may also uncouple the airways and the surrounding lung parenchyma, thereby reducing the elastic force that opposes bronchiolar smooth muscle contraction and promoting airway closure. Airway wall inflammation can also contribute to the destruction of alveolar-bronchiolar attachments, producing deformation and narrowing of the airway lumen. This is supported by the observation that, in smokers, the destruction of alveolar attachments correlates with the degree of inflammation in peripheral airways.
Increased goblet cell metaplasia and subsequent hyperplasia in the small airways of smokers may also limit airflow. The goblet cell may contribute to the increased peripheral airway resistance by producing mucus at a site where it is not normally produced, leading to a marked increase in the surface tension of the airway lining fluid. This would lead to instability of the peripheral airways, facilitating their early closure during expiration. The increase in goblet cells in the peripheral airway epithelium of smokers is associated with an increased number of neutrophils. Because neutrophil elastase is a potent secretagogue, the colocalization of neutrophils and goblet cells within the epithelium in COPD may result in increased secretion of mucus by goblet cells.
In smokers with COPD, CD8 + T lymphocytes are increased not only in central airways but also in peripheral airways and in the lung parenchyma (see eTable 43-2 ). CD8 + cytotoxic T cells are thought to play a role in the rapid resolution of acute viral infections that are frequent in patients with COPD. The observation that people with frequent childhood respiratory infections are more prone to develop COPD supports the role of current and latent viral infections in this disease. In response to repeated or persistent viral infection, it is possible that an excessive number of CD8 + T lymphocytes may be recruited and damage the lung in susceptible smokers, possibly through the release of TNF-α and perforins. Conversely, it is also possible that CD8 + T lymphocytes can damage the lung directly, even in the absence of viral infection. It has been hypothesized that the CD8 + cytotoxic T cell and other inflammatory cells accumulate in response to an autoantigen.
Inflammatory changes appear to target the peripheral airways and alveoli. A comparison of the central and peripheral airways shows that the total number of inflammatory cells is increased in the peripheral airways (<3 mm diameter) in patients with chronic bronchitis with normal lung function, compared with control smokers (see eTable 43-2 ). Some studies have shown an increase in total leukocytes and in CD8 + cells in the peripheral airways of patients with mild/moderate COPD in comparison with control smokers. Studies of tissue obtained from lung volume reduction surgery in patients with severe COPD have shown an increase in total leukocytes and in CD4 + and CD8 + lymphocytes in both the peripheral airways and the lung parenchyma. In contrast, smokers with normal lung function show an increased number of macrophages and T lymphocytes in lung parenchyma compared with control nonsmokers, with no changes in CD4 + and CD8 + cells. In patients with mild to moderate COPD, there is an increase in CD8 + cells in the alveolar septa compared with control nonsmokers, with no change in the numbers of neutrophils, macrophages, or CD4 + cells.
The inflammatory response in the peripheral airways may play a role in the fibrosis that characterizes the small airways in patients with moderate/severe COPD. As the disease progresses, small airways develop increased thickness and enhanced inflammatory infiltrate by neutrophils, macrophages, and T lymphocytes (CD4 + and CD8 + T cells), and B lymphocytes. Lymphoid follicles also accumulate within the walls of the bronchioles, and the lumen of these airways are often obliterated by mucus. A number of mechanisms have been proposed to link inflammation and small airway remodeling. Fibroblast growth factor (FGF) and FGF receptor (FGFR) signaling appear to be associated with airway vascular remodeling in chronic bronchitis. In studies of lung tissue from COPD, FGF1 and its receptor FGFR1 are detected by immunohistochemistry in vascular and airway smooth muscle and in airway epithelial cells. FGF1 and/or FGF2 increase the mRNA levels of FGFR1 and induce cellular proliferation of cultured human airway smooth muscle cells. Smokers with COPD show increased expression of FGF in central airways, predominantly due to enhanced expression in the bronchial glands, suggesting that FGF may also have a role in promoting mucus hypersecretion in smokers.
The pattern of cytokine profile and chemokine receptor expression has been investigated in the peripheral airways in COPD. CD8 + T cells in the peripheral airways in COPD are associated with IFN-γ and express CXCR3, a chemokine receptor that is thought to be preferentially expressed on TH1 cells. Moreover, CXCR3 expression is associated with the expression in the epithelium of its ligand CXCL10. This suggests that the CXCR3/CXCL10 axis may be involved in the recruitment of TH1 cells into the peripheral airways of smokers with COPD. These studies also showed that the interaction of CXCL10 with CXCR3 drives the release of macrophage MMP12 by macrophages. MMP12 is a potent elastolytic enzyme, which can cause lung tissue destruction. These data suggest a possible mechanism by which TH1 lymphocytes can drive the progression of small airway and emphysematous destruction, thus relating the inflammation in peripheral airways to surrounding alveoli. Studies also indicate that differences in gene expression in the small airways and surrounding lung parenchyma may result preferentially in fibrosis of small airways but in loss of alveolar cells in the lung parenchyma, resulting in emphysematous destruction.
Mucus Hypersecretion
Mucus forms a film that coats the airway epithelium and is propelled from the periphery of the lung to the upper airways by the coordinated movement of cilia. The main constituents of the mucus layer are mucus glycoproteins (mucins), water, and peptides. Mucus plays a key role in the clearance of foreign material and infectious agents and has important antioxidant properties. In chronic bronchitis, there is an increase in mucus in the airways, resulting from increased production of mucins and increased secretion from goblet cells.
Mucus production and secretion in COPD is regulated by multiple cellular and molecular mechanisms. Goblet cells express MUC5AC and MUC2 while glandular mucosal cells express MUC5B, MUC8, and MUC9. Enhanced expression of MUC5B has been shown in the bronchiolar epithelium of COPD patients. A number of stimuli such as neutrophil elastase, lipopolysaccharides (LPSs), IL-1β, TNF-α, cigarette smoke, and oxidative stress cause goblet cell metaplasia and mucus hypersecretion. Neutrophil elastase increases MUC5AC mRNA levels by enhancing mRNA stability. LPS also induces MUC5AC expression in animal models, associated with neutrophil infiltration and increased expression of MMP9.
Signaling pathways initiated by epidermal growth factor (EGF) receptor phosphorylation, which can be induced by cigarette smoke-derived oxidative stress, EGF, or TGF-α, also play an important role in mucin production in airway epithelial cells. ROS can activate the serine protease tissue kallikrein, which can then cleave the transmembrane precursor of EGF. Cigarette smoke produces ROS, also resulting in vascular endothelial growth factor receptor (VEGFR) activation and mucus production by activation of the TNFα converting enzyme (TACE), resulting in loss of TGF-α in airway epithelial cells. Acrolein, a component of cigarette smoke, can also induce MUC5AC expression created by ligand/dependent activation of EGFR and mediated by TACE and MMP9. In addition, ROS derived from cigarette smoke can activate an Src-dependent signaling cascade and trigger transcriptional regulation of MUC5AC mediated by binding of the activator protein-1 response element by JunD and Fra-2. Moreover, cigarette smoke extract has been shown to synergize with LPS or TNF-α in the induction of MUC5AC expression, suggesting a potential amplification by cigarette smoke and inflammatory stimuli relevant to the pathogenesis of COPD. A number of transgenic mice experiments have begun to unravel the complex interplay between inflammation, oxidative stress, and growth factors in the development of mucus hyperplasia. These studies suggest that CD4 + Th2 cells and the cytokine network including IL-4, IL-10, and IL-13 play a crucial role in the development of goblet cell hyperplasia/metaplasia.
Proteases/Antiproteases
A protease/antiprotease imbalance, leading to the breakdown of connective tissue components, particularly elastin, has been considered to be the critical mechanism in the pathogenesis of emphysema in smokers. This concept developed from studies showing the development of early-onset emphysema in patients deficient in the major anti-elastase α 1 -antitrypsin (A1AT) and from animal studies showing the development of emphysema in response to the instillation of proteolytic enzymes. Pallid mice, which have decreased A1AT levels, develop emphysema earlier on exposure to cigarette smoke than mice with normal A1AT levels. Furthermore, mice lacking neutrophil elastase are protected from chronic cigarette smoke–induced emphysema.
Elastin is the principal component of elastic fibers and is secreted from several cell types as a precursor, tropoelastin. These tropoelastin molecules become aligned in the extracellular space on microfibrils. Under the action of lysyl oxidase, the lysine residues in tropoelastin are modified, causing the tropoelastin monomers to cross-link and form larger, insoluble elastin polymers. Because the cross-links, known as desmosines, are unique to elastin, they have been used as a marker of elastin degradation. Elastin turnover is minimal in normal subjects; thus, breakdown products should not be detectable. However, desmosine and elastin peptides are elevated in smokers and patients with COPD. In addition, studies have shown that the annual rate of decline in FEV 1 in a group of smokers correlated positively with urine levels of desmosine.
Elastin is an important target for proteolytic enzymes, and its destruction results in loss of elasticity in the lung parenchyma. Together with the destruction of elastin, inactivation of antiproteases is central to the protease/antiprotease imbalance hypothesis. Early studies showed that the function of A1AT was reduced by about 40% in smokers, compared with nonsmokers. This “functional A1AT deficiency” was thought to result from inactivation of A1AT by oxidants in cigarette smoke. However, most of the A1AT in cigarette smokers remains active and is therefore still capable of protecting against the increased protease burden. Studies assessing the function of A1AT in either chronic or acute cigarette smoking have not been definitive. Only a transient and nonsignificant fall in A1AT activity has been measured in BAL 1 hour after smoking. Thus, the hypothesis that the major event is an imbalance between an increased elastase burden in the lungs and a “functional deficiency” of A1AT, due to its inactivation, is an oversimplification.
There are increased numbers of neutrophils and macrophages in the airspaces in chronic smokers, which may increase the elastase burden by the release of elastase from activated neutrophils. In support of this, neutrophils isolated from patients with emphysema show greater elastase-induced fibronectin degradation in vitro than cells from control subjects matched for age and smoking history. Other studies have invoked a contributory role for other antiproteases, such as antileukoprotease, or more subtle changes (e.g., a decrease in the association rate constant of A1AT for neutrophil elastase, which may contribute to elastin degradation).
There is also evidence that an abnormality in elastin synthesis and repair may be involved in the pathogenesis of emphysema. In an animal model of elastase-induced emphysema, treatment with retinoic acid restored normal alveolar architecture. These studies in adult rats (which have continued lung growth throughout their adult life, in contrast with humans) provide some evidence that the destructive process in emphysema, which was always considered irreversible, may be capable of repair. However, human studies of retinoic acid have not shown any evidence of repair of emphysema.
In addition to serine proteases, cysteine proteases (cathepsins) may have a role in the pathogenesis of COPD. Cathepsin-L has been detected in BAL from patients with emphysema, and alveolar macrophages in patients with COPD secrete more cysteine proteases than macrophages from normal smokers or nonsmokers.
Matrix metalloproteases (MMPs) are a group of at least 20 proteolytic enzymes that have a role in tissue remodeling and repair associated with normal development and inflammation, by degrading collagen, laminin, and elastin. They are characterized in distinct subclasses depending on their substrate specificity, amino acid similarity, and identifiable sequence molecules. The subclasses are collagenases (MMP1, 8, 13), gelatinases (MMP2, 9), stromelysin S (MMP3, 10, 11), membrane-type MMP14–MMP25), matrilysin (MMP7), and macrophage metalloelastase (MMP12). The major inhibitors of MMPs are alpha-2 macrogrobulin and the tissue inhibitor of metalloproteases (TIMP) family.
There is substantial evidence for a role of MMPs in the pathogenesis of COPD. Several studies have shown increased expression of several MMPs in the lungs of COPD patients. MMP12 protein has been observed in sputum, BAL, bronchial biopsies, and peripheral lung tissue in patients with severe emphysema. Increased concentrations of MMP1 (collagenase) and MMP9 (gelatinase B) are present in BAL from patients with COPD, and there is increased activity of MMP9 in the lung parenchyma of patients with emphysema. MMP12 mRNA can be induced by exposure of human bronchial epithelial cells to cigarette smoke extract or cytokine mix (TNF-α and IFN-γ). Alveolar macrophages from smokers express more MMP9 than those from normal subjects, and there is an even greater increase in patients with COPD.
There is considerable evidence from experimental models to link MMP12 and the development of emphysema. Increased MMP12 expression was present in alveolar macrophages after smoke exposure in C57BL/6 mice, and animal models have shown that cigarette smoke does not induce emphysema in mice lacking MMP12. MMPs are also known to activate the latent form of TGF-β. In mice lacking the integrin αVβ6, there is a failure to activate TGF-β, and these animals do not develop age-related emphysema, which can be overcome by overexpression of TGF-β1. These data suggest that TGF-β may down-regulate MMP12 under normal conditions and that the absence of TGF-β results in excessive MMP12 production and emphysema. Although MMP9 and MMP2 both degrade elastin and are both expressed in COPD lungs, the role of these MMPs in the pathogenesis of COPD remains unclear.
Oxidants/Antioxidants in COPD
Cigarette smoke is a complex mixture of more than 4700 chemical compounds, including high concentrations of free radicals and other oxidants. The oxidant burden in lungs may be further enhanced in smokers by the increased numbers of neutrophils and macrophages in the alveolar space that release increased amounts of oxidants, such as oxygen and H 2 O 2 . Other sources of ROS are those generated through normal cellular processes in the lungs, such as those produced by normal cellular respiration, or by inhalation of air pollutants, such as particulate pollution.
A delicate balance exists between the toxicity of oxidants and the protective effects of intracellular and extracellular antioxidant defense systems, which are critically important for the maintenance of normal pulmonary cellular functions. A shift of the oxidant/antioxidant balance in favor of oxidants is known as oxidative stress. There is now considerable evidence of increased oxidative stress in smokers and patients with COPD.
All tissues are vulnerable to oxidant damage but, by virtue of its direct contact with the environment, the airspace epithelial surface of the lung is particularly vulnerable. Injury to the epithelium, manifested as an increase in airspace epithelial permeability, may be an important early event following exposure to cigarette smoke. Extracellular and intracellular glutathione, an antioxidant, appears to be critical to the maintenance of epithelial integrity following exposure to cigarette smoke. This was shown in studies in which the increased permeability of epithelial cell monolayers in vitro, and in rat lungs in vivo, following exposure to cigarette smoke condensate, was associated with profound changes in the homeostasis of glutathione. These in vitro and animal studies are paralleled by human studies demonstrating increased epithelial permeability in chronic smokers compared with nonsmokers, with a further increase in epithelial permeability following acute smoking. Thus, cigarette smoke has a detrimental effect on alveolar epithelial cell function that is, in part, oxidant mediated.
A major site of free radical attack is on polyunsaturated fatty acids in cell membranes producing lipid peroxidation, a process that may continue as a chain reaction to generate hydroperoxides and long-lived aldehydes. Levels of products of lipid peroxidation in plasma and BAL are significantly increased in healthy smokers and in patients with acute exacerbations of COPD, compared with healthy nonsmokers.
Several studies demonstrate increased levels of oxidants in exhaled air or breath condensates in COPD patients. Furthermore, there is evidence that oxidative stress can cause increased lipid peroxidation in lung tissue in COPD patients compared with smokers who have a similar smoking history but have not developed the disease, with the level of lipid peroxidation correlating with the degree of airflow limitation.
Free iron is a critical element in many oxidative processes. Macrophages from smokers have been shown to contain more iron than those from nonsmokers, and they release more iron, thus potentially increasing the oxidant burden in smokers.
The major antioxidants in respiratory tract lining fluid include mucin, reduced glutathione, uric acid, protein (largely albumin), and ascorbic acid. There is limited information on respiratory epithelial antioxidant defenses in smokers, and even less on those in COPD patients. Studies have shown that glutathione is elevated in the BAL from chronic smokers. Even so, glutathione may not be present in sufficient quantities to deal with the excessive oxidant burden during acute smoking because cigarette smoke exposure depletes glutathione in a dose- and time-dependent manner.
Other antioxidants such as vitamin C and E have shown variable changes in COPD patients. Reduced levels of vitamin E are present in the BAL of smokers compared with nonsmokers. By contrast, other studies found a marginal increase in vitamin C in the BAL of smokers, compared with nonsmokers. The apparent discrepancy may be due to different smoking histories in chronic smokers, particularly the time of the last cigarette in relation to the sampling of BAL.
There is an imbalance between oxidants and antioxidants in COPD resulting in oxidative stress, which underlies many of the pathogenic mechanisms in COPD. Numerous studies documented increased expression of markers of oxidative stress in the lungs of COPD patients, compared with smokers who have not developed COPD. 4-hydroxy-2-nonenal (4HNE), a highly reactive lipid peroxidation end product, reacts quickly with extracellular proteins to form adducts. 4HNE adducts are present in greater quantities in airway epithelial and endothelial cells in the lungs of COPD patients, compared with smokers with a similar smoking history who have not developed the disease. 4HNE can act as a chemoattractant for neutrophils and is also involved in numerous cellular functions such as cell proliferation, growth inhibition, T-cell apoptosis, and activation of various signaling pathways. 4HNE has also been shown to activate the synthesis of the antioxidant glutathione, by induction of the glutamate cysteine ligase gene as an antioxidant response to increased oxidative stress, and also of a variety of proinflammatory genes coding for IL-8, MCP-1, EGF, and MUC5AC. The presence of increased oxidative stress in COPD lungs is confirmed by the finding of increased expression of 8-hydroxy-2 deoxy-guanosine, formed by the reaction of hydroperoxides with the DNA base guanosine.
Many actions of oxidative stress can potentially play a role in the pathogenesis of COPD. These include the inactivation of anti-proteases (such as A1AT or secretory leukoprotease inhibitor) or activation of metalloproteases by oxidants, resulting in a protease/antiprotease imbalance in the lungs. Oxidants can directly damage components of the lung matrix (e.g., elastin and collagen) and also interfere with elastin synthesis and repair.
Oxidative stress also influences the molecular mechanisms involved in proinflammatory gene expression. Gene activation by transcription factors is dependent on a number of factors, among which is the remodeling of DNA dependent on the nuclear histone acetylation/deacetylation balance, controlled by the activities of histone acetyl-transferases (HATs) and histone deacetylases (HDACs). DNA in the resting cell is coiled around a nucleosome core of histone residues. This configuration suppresses the accessibility of transcription factors such as NF-κB to their cognate DNA sequences. Acetylation of the lysine residues in the core histones results in uncoiling of the DNA, increasing the accessibility of transcription factors and RNA polymerase 2 and hence increased gene transcription. Deacetylation, under the influence of HDACs, results in rewinding of the DNA around the histone proteins, decreasing gene transcription. Macrophages from cigarette smokers show a decrease in histone deacetylase activity, as has also been shown in the lungs of smoke-exposed animals associated with nitrotyrosine, 4HNE, and aldehyde-modified HDAC proteins, resulting in their enhanced degradation in the proteosome system and thus decreased HDAC activity.
Studies of resected lung indicate that HDAC2 activity is reduced in lung tissue in COPD, associated with lower HDAC2 RNA levels and expression of HDAC2 protein as a result of modification of HDACs by oxidants. This decrease in HDAC increased with disease severity and was associated with an increase in histone-4 acetylation at the IL-8 promoter and increased in IL-8 mRNA expression. Thus, molecular mechanisms such as transcription factor activation and chromatin remodeling, as a result of increased oxidative stress, may be responsible for perpetuating inflammation in COPD.
The most compelling data in support of the causal role of oxidative stress in the pathogenesis of emphysema have been provided by studies outlining the role of the master antioxidant transcription factor nuclear erythroid–related factor 2 (NRF2) in the disease. NRF2 controls the expression of more than 100 gene products, including several of the most important antioxidant enzymes. Human lungs and alveolar macrophages from COPD patients have decreased expression of NRF2 transcriptional activity. The protective role of NRF2 in emphysema is underscored by the increased susceptibility of NRF2 null mice to cigarette smoke. Furthermore, enhancement of NRF2 expression using a small molecule activator protected wild type mice against cigarette smoke–induced emphysema.
Oxidative stress is linked to cell death, including alveolar cell apoptosis in the setting of human and experimental emphysema. Moreover, oxidative stress underlies several of the mechanisms thought to participate in aging, which may lower the threshold for lung injury to cigarette smoke.
Apoptosis and Emphysema
Alveolar Cell Death in Emphysema
Studies have documented that lung cells undergo apoptosis in emphysematous lungs, predominantly involving endothelial cells in the alveolar walls, compared with normal smokers’ lungs. Experimental emphysema in animals can be produced by decreased VEGF or VEGF signaling, and studies in human lungs demonstrated decreased expression of VEGF and VEGF-receptor 2 expression in association with emphysema. The aggregate of these data led to the concept of an alveolar maintenance program that was required for structural preservation of the lungs. Cigarette smoke is thought to cause destruction of this maintenance program, thus causing emphysema.
Lung tissue is destroyed due to the mutual interaction of alveolar cell apoptosis, oxidative stress, and protease/antiprotease imbalance. This concept is supported by observations that antioxidant treatment prevented both apoptosis and emphysema induced by down-regulation of VEGF.
Inflammation can be triggered and amplified by alveolar injury, including either enhanced alveolar cell apoptosis or defective apoptotic cell clearance. Targeted alveolar cell apoptosis using a chimeric peptide containing a lung homing sequence linked to a proapoptotic peptide led to emphysematous enlargement of murine lungs, associated with alveolar cell apoptosis, oxidative stress, macrophage influx, and increases in ceramide levels. Moreover, viral infections (such as influenza virus) can synergize with cigarette smoke to cause experimental emphysematous tissue destruction involving alveolar cell death and secondary lung inflammation.
Role of Aging in the Pathogenesis of Emphysema
The evidence of shared features between pulmonary emphysema and lung aging has led to the hypothesis that both conditions share underlying mechanisms, including oxidative stress, inflammation, and apoptosis.
The cellular equivalent of aging is senescence, which is characterized by a nonproliferative state in which cells are metabolically active and apoptosis resistant. A number of molecular and cellular mechanisms are associated with cellular senescence, including accumulation of DNA damage, impairment of DNA repair, epigenetic modifications in nuclear DNA, protein damage from oxidative stress, and telomere attrition. Central to “end replication senescence” is the erosion of telomeres, with the ensuing activation of DNA repair enzymes, and the cell cycle control kinase inhibitors p53, p21, and p16. These signaling processes converge on de-phosphorylated retinoblastoma protein, which potently inhibits cell cycle progression. Cellular senescence also takes place in vivo, particularly in conditions associated with aging. Several markers of cellular senescence are present in vivo, particularly senescence-associated β-galactosidase (SA-β-gal), and the increased expression of cyclin-kinase inhibitors p16 and p21.
Cigarette smoke extract leads to increased SA-β-gal expression in cultured type II cells or lung fibroblasts, which have also been shown in lung fibroblasts from emphysematous lungs. Oxidative stress enhances telomere shortening. Furthermore, alveolar epithelial and endothelial cells in emphysematous lungs also have increased expression of p21 associated with decreased telomere length. The decreased telomere length parallels similar findings in circulating peripheral blood leukocytes. An association has been shown between blood leukocyte telomere length and pack-years of smoking, and telomeres are shorter in blood leukocytes from COPD patients compared with control subjects.
Sirtuins are type III histone deacetylases that have a role in a number of processes including stress resistance, apoptosis, cell senescence, differentiation, and aging. Sirtuin-1 (SIRT1) is essential for maintaining silent chromatin via the deacetylation of chromatin. Environmental stress, such as cigarette smoke exposure, decreases SIRT1 levels in both macrophages in vitro and in rat lungs in vivo, associated with increased expression of inflammatory cytokines. SIRT1 has been shown to be decreased in lung cells from COPD patients, compared with smokers who have not developed the disease, as a result of post-translational oxidative modification. This would accelerate the process of aging and also enhance inflammation.
No single mechanism can account for the complex pathology in COPD. It is likely that interactions transpire between different mechanisms. For example, there are probably interrelationships between protease/antiprotease, oxidative stress, and apoptosis as destructive processes in emphysema. Better understanding of the relative importance of these different pathogenic mechanisms will come from proof-of-concept therapeutic intervention studies.
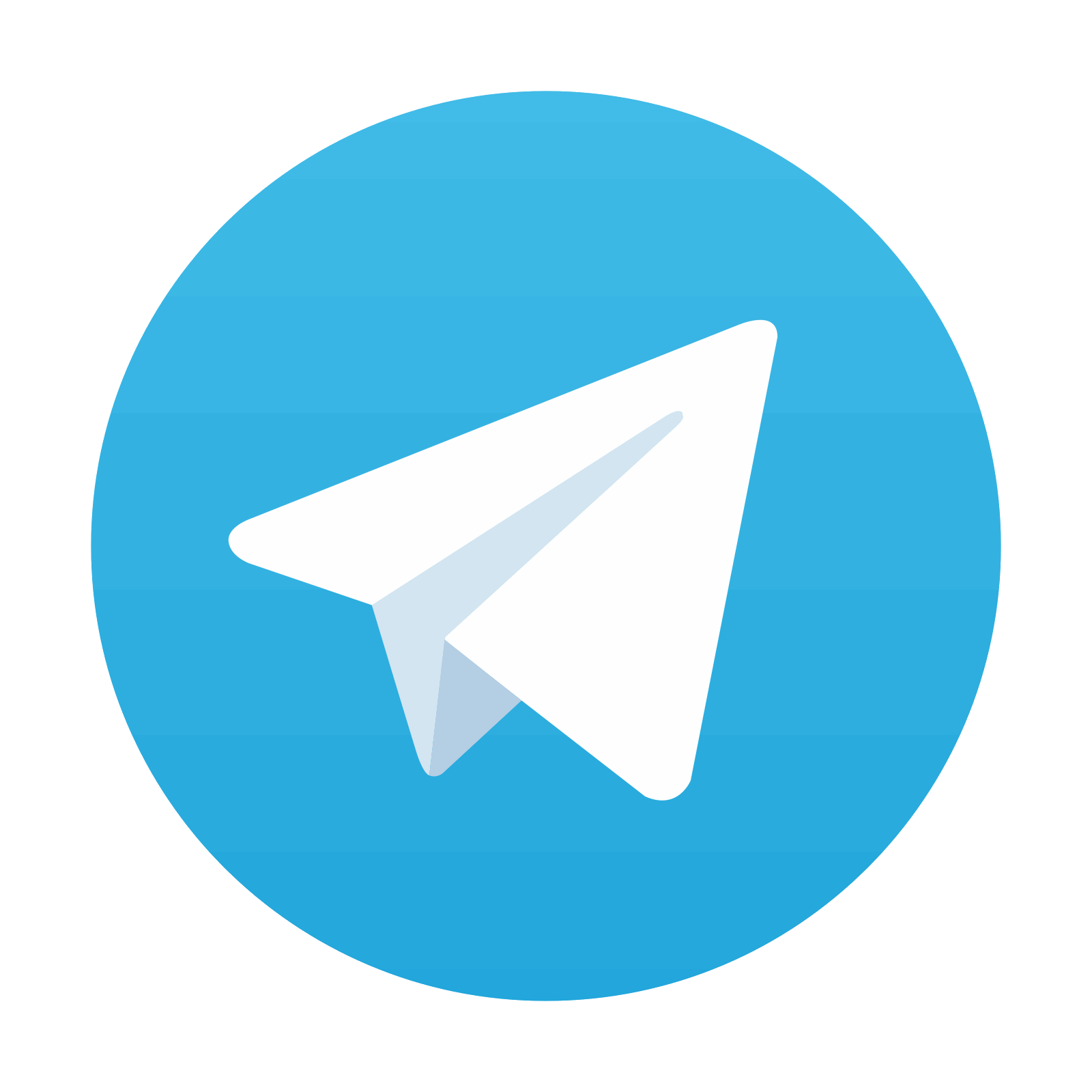
Stay updated, free articles. Join our Telegram channel

Full access? Get Clinical Tree
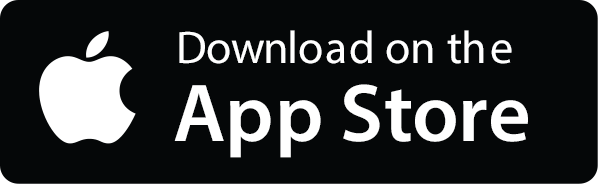
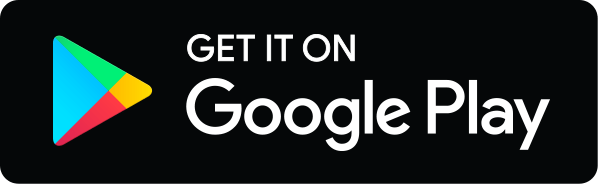