Contrast Echocardiography: Introduction
- Sanjiv Kaul, MD
In 4 years, contrast echocardiography will be celebrating its Golden Jubilee. It was first described in 1968 by Gramiak and Shah and then found increasing use for cardiac shunt detection using hand agitated saline solutions. Assessment of myocardial perfusion became possible in the early 1980s using sonicated contrast agents, and precision microbubble development for transpulmonary transit started a decade later. Microbubbles are now successfully used for left ventricular cavity opacification during rest and stress, enhancement of Doppler signals, and myocardial perfusion. They have also been used for cardiac tumor characterization, skeletal muscle perfusion in patients with peripheral artery disease, quantification of plaque burden, assessment of plaque activity (the latter through vasa vasorum–derived perfusion ), and assessment of renal blood flow. These microbubbles have been used to evaluate skin graft viability and quantify cerebral perfusion in animal models. In addition, there is exciting research in the field of molecular imaging with ultrasound contrast agents.
Major developments in contrast echocardiography have occurred in the past two decades, with the advent of second- and third-generation ultrasound contrast agents, online signal processing routines for detecting microbubbles in tissue, creation of protocols and algorithms for quantifying tissue perfusion, and studies showing safety of contrast agents that have allowed their increasing use in the clinical setting. However, contrast echocardiography is still underused for various reasons.
In this section of the book, leaders in the field discuss important elements in state-of-the-art contrast echocardiography. Thomas Porter provides an update on ultrasound contrast agents. Jonathan Lindner reviews the physics of ultrasound-microbubble interactions. Kevin Wei discusses current applications and limitations of contrast echocardiography. Sharon Mulvaugh defines the role of contrast echocardiography in stress testing. And finally, Steven Feinstein elucidates the role of contrast agents in imaging the carotid arteries. The reader is directed to a white paper sponsored by the American Society of Echocardiography on future research directions and applications of ultrasound contrast agents.
Ultrasound Contrast Agents
- Joan Olson, BS, RDCS, RVT
- Feng Xie, MD
- Thomas Porter, MD
- Feng Xie, MD
The currently available ultrasound contrast agents in the United States are Optison (General Electric Healthcare, Princeton, NJ) and Definity (Lantheus Imaging, North Billerica, Mass.). In Europe and Brazil, Sonovue (Bracco Diagnostics, Colleretto Giacosa, Italy) is an approved contrast agent for left ventricular opacification ( Table 22.1 ). Agents that may be approved in the near future in Europe include Imagify (Acusphere, Inc, Lexington, Mass.). Sonozoid (General Electric Healthcare, Princeton, NJ) is approved in Japan for tumor imaging, but not for cardiac applications.
Agent | Manufacturer | Shell | Gas | Approved |
---|---|---|---|---|
Optison | GE Healthcare | Albumin | Perfluoropropane | LVO, USA |
Definity | Lantheus Medical Imaging | Lipid | Perfluoropropane | LVO, USA |
SonoVue | Bracco Diagnostics | Amphiphilic phospholipids | Sulfur hexafluoride | LVO and Doppler, Europe/Brazil |
Imagify | Acusphere | Polymer | Perfluorobutane | Seeking approval in Europe |
Sonozoid | GE Healthcare | Lipid | Perfluorobutane | Noncardiac, Japan |
Contrast agents in the United States are currently approved only for left ventricular opacification. In Europe, SonoVue is approved for improving the detection of coronary artery disease and Doppler enhancement. Imagify is seeking approval for both improved left ventricular opacification and myocardial perfusion. However, all of these agents can be used to examine myocardial blood flow with perfusion imaging techniques available for more than 10 years on Philips and Siemens ultrasound systems, and now available on Toshiba and General Electric ultrasound scanners.
Ultrasound contrast agent composition
The improvement in left ventricular opacification achieved with ultrasound contrast agents following venous injection or infusion has been related to the incorporation of high molecular weight gases inside the microbubble shell (see Table 22.1 ). High molecular weight gases (sulfur hexafluoride or perfluorocarbons) have both lower diffusivity and lower blood solubility, which prolongs their gas phase in blood.
Technical considerations and responsibilities
The success of contrast imaging depends on gain optimization, time gain compensation, and mechanical index (MI) for imaging. Additionally, the contrast infusion rate or bolus size must be controlled so that myocardial contrast enhancement can be obtained without shadowing in the LV cavity.
Role of the physician
The physician is responsible for the overall quality control of the procedure, which begins by ensuring all personnel (cardiology fellows, nurses, and sonographers) understand the concept of left ventricular opacification optimization that is necessary for both bolus injections and continuous infusions of microbubble contrast. The physician must work with the lead sonographer and nursing team to develop a standard operating procedure for using contrast. The physician assigned to the lab that uses contrast agents must have completed Level II or III training in echocardiography; training must also include performing and interpreting at least 50 contrast examinations with assistance before operating independently.
Role of the sonographer or nurse
Contrast is used to enhance images, improve border detection, and provide information on myocardial perfusion at rest and during functional stress echocardiographic studies. The contrast agent is administered by a registered nurse or other qualified medical personnel. A continuous infusion or bolus injection of Definity or Optison contrast is used. The nurse or sonographer prepares the contrast solution before acquiring images. Definity is activated by agitating the vial for 45 seconds in the Vialmix ( Fig. 22.1 ). Optison and SonoVue are distributed in glass vials that are ready for use. For bolus injections, it is recommended that initial doses be low (0.1 mL for Definity, 0.2 mL for Optison or SonoVue) and that the flush be 5 to 10 mL of saline for 10 seconds. This prevents excessive opacification and shadowing in the far field. For contrast infusions, dilute 0.8 mL of Definity into 29 mL normal saline for a total of approximately 30 mL; or half of a vial of Optison or SonoVue can be diluted into 20 mL of saline. The infusion rate is approximately 4 mL per minute by hand, watching the clock. The solution should be mixed back and forth periodically so that the contrast does not settle in the bottom of the syringe.

Safety of ultrasound contrast agents
In October 2007, the U.S. Food and Drug Administration (FDA) issued a Boxed Warning regarding the use of Definity and Optison, stating that deaths had occurred within 30 minutes of contrast administration ( http://www.fda.gov/Drugs/DrugSafety/PostmarketDrugSafetyInformationforPatientsandProviders/ucm110260.htm ). These findings seemed inconsistent with what was observed in routine practice. Several single-center and multicenter studies have evaluated the safety of ultrasound contrast ( Table 22.2 ). In all these studies, no such increase in mortality was observed when compared with an equivalent patient population not receiving contrast. As shown in Table 22.2 , there was no increased risk associated with contrast use in more than 100,000 patients, including patients in the intensive care setting, patients with potential acute coronary syndromes undergoing resting or stress echocardiography, or in patients following acute myocardial infarction. More recent publications focusing on the use of contrast within the first 24 hours of hospital admission have demonstrated that patients receiving contrast may have a reduced mortality rate compared with patients not receiving contrast, suggesting that the increased diagnostic accuracy afforded by the use of contrast may lead to earlier correct diagnoses and subsequent reduced mortality.
Authors | Year | Contrast Agent | Study Nature | Total Patient No. | Contrast Patient No. | Noncontrast Patient No. | Contrast | Noncontrast | ||
---|---|---|---|---|---|---|---|---|---|---|
Death | MI | Death | MI | |||||||
Wei et al | 2012 | Definity | PH | 32 | 32 | n/a | 0 (%) | 0 (0%) | n/a | n/a |
Wever-Pinzon et al | 2012 | Definity | PH | 1513 | 1513 | n/a | 1 (0.06%) | 0 (0%) | n/a | n/a |
Exuzides et al | 2010 | Optison | Critically iII | 14,500 | 2900 | 11,600 | 38 (1.3%) | n/a | 129 (1.1%) | n/a |
Abdelmoneim et al | 2010 | Definity Optison | Elevated RVSP | 16,434 | 6164 | 10,270 | 1 (0.016%) | 3 (0.03%) | 2 (0.03%) | 5 (0.05%) |
Abdelmoneim et al | 2009 | Definity Optison | 26,774 | 10,792 | 15,982 | 1 (0.009%) | 3 (0.028%) | 2 (0.013%) | 7 (0.044%) | |
Dolan et al | 2009 | Definity Optison | 48,308 | 42,408 | 5900 | 0 (0%) 30 min 2 (0.01%) 24 hr | 0 (0%) 30 min 8 (0.019%) 24 hr | 0 (0%) within 30 min 1 (0.017) within 24 hr only rest echo | 0 (0%) within 30 min 7 (0.01%) within 24 hr only rest echo | |
Gaibazzi et al | 2009 | SonoVue | 500 | 500 | n/a | 0 (0%) | 0 (0%) | 0 (0%) | 2 (0.1%) |
Authors | Year | Contrast Agent | Study Nature | Total Patient No. | Contrast Patient No. | Noncontrast Patient No. | Contrast | Noncontrast | ||
---|---|---|---|---|---|---|---|---|---|---|
Death | MI | Death | MI | |||||||
Anantharam et al | 2009 | SonoVue Luminity | 2775 | 689 | 2086 | 0 (0%) | 0 (0%) | 0 (0%) | 2 (0.1%) | |
Main et al | 2008 | Definity | Hospitalized patients | 4,300,966 | 58,254 | 4,242,712 | 616 (1.06%) | n/a | 45,789 (1.08%) | n/a |
Gabriel et al | 2008 | Definity Optison | 9798 | 4786 | 5012 | 2 (0.04%) | n/a | 3 (0.06%) | n/a | |
Wei et al | 2008 | Definity Optison | 78,383 | 78,383 | n/a | 0 (0%) | n/a | n/a | n/a | |
Kusnetzky et al | 2008 | Definity | Hospitalized patients | 18,671 | 6196 | 12,475 | 26 (0.42%) | n/a | 46 (0.37%) | n/a |
Shaikh et al | 2008 | Definity Optison | 5069 | 2914 | 2155 | 0 (0%) | 1 (0.03%) | 0 (0%) | 0 (0%) | |
Herzog | 2008 | Definity Optison | 28,999 | 28,999 | n/a | 0 (0%) | 0 (0%) | n/a | n/a |
A rare anaphylactoid reaction may occur in response to Definity; this is estimated to occur with a frequency of 0.006%. Therefore, all nurses and sonographers using Definity should have hospital policies that ensure recognition of such a rare reaction and its treatment (epinephrine, advanced cardiac life support measures for maintenance of airway and treatment of hypotension/shock).
The safety and efficacy of ultrasound contrast agents in patients with pulmonary hypertension and right-to-left cardiac shunts has also been demonstrated, although this issue continues to be a concern of the FDA. Ultrasound contrast agents have been shown to be safe even in high-risk patients with pulmonary hypertension and right-to-left cardiac shunts.
Physical Properties of Microbubble Ultrasound Contrast Agents
- Jonathan R. Lindner, MD
Microbubble contrast agents
Although a wide variety of acoustically active ultrasound (US) contrast agents have been developed, agents that have been approved by regulatory agencies for routine diagnostic use in humans are composed of encapsulated microbubbles. Key considerations that have guided the design of these agents are that they must be safe, able to transit the pulmonary and systemic microcirculation unimpeded, and be sufficiently stable after intravenous injection to reach the left ventricular cavity and the myocardium. To satisfy these requirements, microbubbles must be smaller than the effective diameter of the pulmonary and systemic capillary beds (less than 6 to 7 μm) and yet contain enough gas volume to be easily detected by US. The in vivo stability of microbubbles has been achieved by two strategies. Encapsulation of the microbubbles using lipid surfactant or albumin “shells” has been used to reduce outward diffusion and reduce microbubble surface tension. Microbubble stability has been enhanced further by using biocompatible high molecular weight gases such as perfluorocarbons (octafluoropropane [C 3 F 8 ], decafluorobutane [C 4 F 10 ]) or sulfur hexafluoride (SF 6 ). These gases have both low solubility (low Ostwald coefficient) and low diffusion coefficients, which, according to Epstein-Plesset modeling of the stability of free bubbles, markedly increase their life span. A partial list of microbubble agents approved for use in humans is provided in Figure 23.1 .

The behavior of commercially produced microbubbles in the microcirculation, or their rheology, has been well characterized because of both safety considerations and the importance of rheology when using microbubbles as a perfusion tracer. Rheologic studies have relied on either (1) comparing microbubble transit rates on imaging with those of labeled erythrocytes, or (2) intravital microscopy where microbubble transit can be directly visualized. These techniques have definitively demonstrated that microbubble behavior is similar to that of erythrocytes and they transit the microcirculation of normal tissues unimpeded, with the exception of reticuloendothelial organs (e.g., the liver) where uptake occurs for many microbubbles as part of their normal clearance pathway.
The acoustic detection of gas-filled microbubbles within the vascular compartment is based on their compressibility ( Fig. 23.2 , A ). The gases that have been used in microbubble contrast agents are several orders of magnitude more compressible than water or tissue. Because they are smaller than US wavelengths, they undergo volumetric oscillation, whereby they compress and expand during the pressure peaks and troughs of the US pulse. When imaging at low acoustic power, the degree of signal enhancement is governed by the magnitude of oscillation, also called stable cavitation . The mechanical index (MI), defined as the peak negative acoustic pressure divided by the square root of the transmit frequency, at which stable cavitation occurs is less than 0.25 for most agents. At higher acoustic pressures (MI generally greater than 0.5), exaggerated microbubble oscillation produces microbubble destruction, which is termed inertial cavitation . This activity produces very strong broadband US signals by a variety of mechanisms, the most important of which is probably the abrupt release of free gas microbubbles from the confines of their shell, which than can undergo nondamped exaggerated oscillation. The inertial cavitation phenomenon is also important for nullifying contrast signal when performing quantitative perfusion imaging, which is discussed elsewhere.

The schemes used clinically to augment microbubble signal relative to tissue rely on the detection of specific acoustic signatures produced by nonlinear oscillation. Nonlinear oscillation is defined by a variety of microbubble vibration behaviors in which the changes in microbubble volume are eccentric and not linearly related to the applied US pressure. The magnitude of oscillation and nonlinear behavior in turn depends on the compressibility and density of the gas, the viscosity and density of the surrounding medium, the frequency and power of US, and equilibrium radius of the microbubbles. Viscoelastic damping from the shell is a particularly important issue so that microbubbles will optimally have “flexible” shells. With regard to frequency, there is an ideal resonant frequency at which all forms of radial oscillation becomes efficient and exaggerated. This “resonant frequency” is inversely related to the square of the microbubble’s radius and is also influenced by the viscoelastic and compressive properties of the shell and gas. When performing contrast echocardiography in patients, selection of the ideal frequency for microbubble resonance is generally not a major consideration because the range of frequencies employed in clinical practice are generally close to the ideal resonant frequency for contrast agents approved for use in humans. This is not necessarily the case for high and ultrahigh frequency probes that are used for intravascular and preclinical imaging in small animals.
Contrast-specific imaging techniques
The general principle underlying 2D ultrasound imaging is to receive and filter US signals that are reflected from tissues to generate an image that defines anatomic boundaries and texture. Contrast echocardiography, whether for left ventricular opacification or for myocardial perfusion, is based on different principles. The aim is to minimize tissue signal and amplify microbubble signal to better define endocardial borders or measure the concentration of microbubbles in the myocardial microcirculation. To accomplish this, several pulse schemes have been developed and incorporated into contrast-specific features on most echocardiography imaging systems.
Perfusion imaging and certain research applications (molecular imaging, cavitation-related gene therapy, sonothrombolysis) have been performed with high-power US imaging to generate robust signals. When imaging microbubble agents at high power, inertial cavitation produces very strong but transient broadband signals that contain somewhat higher peaks at the harmonic frequencies, or multiples of the transmission frequency (see Fig. 23.2 , B ). The main limitation, other than the transient nature of the microbubble contrast effect, is that the tissue signal is strong, so it becomes difficult to discern microbubble from tissue signal. Although filtering to receive signals at twice the fundamental (transmission) frequency, called second harmonic imaging, improves microbubble signal-to-tissue ratio, tissue still continues to produce backscatter at the second harmonic frequency, primarily because the ultrasound beam is distorted as it travels through tissue. Tissue signal is more concentrated at the fundamental and harmonic peaks, whereas microbubble inertial cavitation produces a signal response with a much broader band, so off-harmonic imaging either between the fundamental and harmonic frequencies or just beyond the second harmonic (ultraharmonic imaging) are approaches that have been used to increase high-power contrast signal relative to tissue.
A second approach to augmenting microbubble signal-to-noise ratio during high-power imaging is to use online signal processing that further reduces tissue clutter by decorrelation imaging. This approach relies on either radiofrequency decorrelation or Doppler processing to measure the degree to which a packet of sequential ultrasound pulses along a single line differ from each other ( Fig. 23.3 ). Provided there is little tissue motion during the pulse sequences, signals from tissue alone are similar. Therefore the degree of noncorrelation, which determines pixel intensity, is low for tissue and below the wall filter settings. In contrast, microbubbles resident within tissue are destroyed rapidly so that the sequential returning signals are dissimilar and the degree of decorrelation is high, denoted by high pixel intensity.

When performing contrast imaging at low power, tissue does not produce much of a nonlinear signal to interfere with the microbubble signal. However, the amplitude of the nonlinear signal produced by microbubbles at low MI is rather small. Compensation by increasing the gain solves little because it also increases the existing tissue noise. Hence it is important again to eliminate tissue signal to maximize the microbubble signal-to-noise ratio. One approach, termed pulse inversion or phase inversion , relies on successive pulses of ultrasound that have inverted phases (are mirror images of each other) ( Fig. 23.4 , A ). At these low transmission powers, tissue produces mostly linear backscatter, so ultrasound is reflected at the fundamental frequency. By summing the two returning signals, tissue signal can be eliminated. However, microbubbles produce nonlinear signals that do not cancel and are displayed according to the amplitude. Tissue signal during low-power imaging may also be suppressed online by alternating acoustic power rather than phase, often called amplitude or power modulation (see Fig. 23.4 , B ). Several successive pulses are transmitted for each line alternating between low power and very low power (half-amplitude of the low-power pulse). Linear signals that return from tissue are similar in phase and frequency. Accordingly, this signal can be eliminated by doubling the half-amplitude (very low power) signal and subtracting from the low-power signal. Since microbubbles will produce a nonlinear signal at low but not very low power, doubling the low-power linear signal will not completely cancel the signal. Signals that are not cancelled are again displayed according to amplitude, and because noncancellation occurs even at the fundamental frequency, the received bandwidth can be broadened to include even the relatively stronger fundamental frequencies. A final approach has to use a “chirp” technique, in which frequency is gradually increased during a long power pulse, which permits detection of a wider range of the microbubble size distribution.

Clinical perspective
The physical properties of microbubbles with regard to rheology and behavior in an acoustic field are important for safety considerations and understanding and optimizing contrast-enhanced US. Although imaging systems have incorporated many of the microbubble signal detection schemes discussed in this chapter, there are many more user adjustments (power, dynamic range, acoustic focus, line density, etc.) that require knowledge of the interactions between ultrasound and microbubble contrast agents.
Applications of Ultrasound Contrast Agents
- Kevin Wei, MD
Echocardiography plays a critical role in the management of patients, especially in its ability to evaluate left ventricular (LV) global and regional function. Its clinical utility, however, may be affected by image quality. In approximately 10% to 15% of routine echocardiograms and up to 25% to 30% of studies performed in intensive care units, the endocardial border is not clearly defined. Ultrasound contrast agents have been shown in numerous studies to enhance delineation of the endocardial border, which improves both the qualitative and quantitative assessment of LV function, and decreases interobserver and intraobserver variability.
The currently available ultrasound contrast agents are listed in Table 24.1 . They are approved by regulatory agencies in multiple countries for LV opacification, which enhances definition of LV endocardial borders in patients with “technically suboptimal” echocardiograms at rest. The agents are also approved for enhancement of Doppler signals. Apart from helping to improve assessment of LV systolic function, ultrasound contrast agents have been shown to enhance the detection and evaluation of multiple other structural abnormalities. This chapter provides examples of common scenarios where ultrasound contrast agents can provide better delineation of not only cardiac function but also pathology. Not all current clinical applications can be reviewed within the scope of this chapter, and for more details, the reader is referred to the most recent consensus statement about contrast agents released by the American Society of Echocardiography.
Agent | Bubble Size, Mean (Range) (μm) | Gas | Shell Composition | Indication |
---|---|---|---|---|
Levovist * | 2-3 (2-8) | Air | Lipid (palmitic acid) | LVO and Doppler |
Optison † | 4.7 (1-10) | Perfluoropropane | Human albumin | LVO and Doppler |
Definity † | 1.5 (1-10) | Perfluoropropane | Phospholipid | LVO and Doppler |
Imagent * | 5 | Perfluorohexane nitrogen | Stabilized surfactant | LVO |
SonoVue ‡ | 2.5 (1-10) | Sulfur hexafluoride | Phospholipid | LVO and Doppler |
* Not currently commercially available.
† Approved by the U.S. Food and Drug Administration (FDA) in the United States (Optison and Definity are also approved in Canada, and Definity is approved in Europe under the name Luminity).
‡ Approved in Canada, Europe, and some Latin American and Asian countries.
Clinical applications
Assessment of Cardiac Function
The evaluation of right and left ventricular systolic function is a cornerstone for echocardiography, and it is one of the most common indications for requesting an echocardiographic study. For LV function, inadequate visualization of two or more endocardial borders has been used to define a study as “technically difficult” or “suboptimal.” However, ultrasound contrast agents could be used even in studies that are technically adequate, especially if quantification of LV volumes or ejection fraction is required, or if serial accurate assessments of cardiac function are sought. Ultrasound contrast agents improve feasibility, accuracy, and reproducibility of echocardiography for qualitative and quantitative assessment of LV structure and function, at rest and during exercise or pharmacologic stress. Kurt and colleagues evaluated 632 consecutive patients who were considered to have technically difficult or uninterpretable resting echocardiograms. The patients were from inpatient wards, medical and surgical intensive care units, and the outpatient lab, and the study showed that the number of uninterpretable studies declined from 11.7% to 0.3%, and the number of technically adequate studies increased from 1.6% to 89.9% after ultrasound contrast agents were used for LV opacification. Ultrasound contrast agent use significantly improved patient management as well; additional diagnostic procedures were unnecessary in 32.8% of patients, and drug management was changed in 10.4%.
Accurate determination of LV ejection fraction (LVEF) provides important prognostic information for patients who have had myocardial infarction and in those with congestive heart failure. Echocardiography can be used serially to assess cardiac function because it uses no ionizing radiation and is easily accessible, portable, and relatively cheap compared with other imaging techniques. It is therefore often used to monitor patients receiving cardiotoxic drugs, and to determine which patients may require device implantation. The use of ultrasound contrast agents improves the accuracy of LVEF quantification compared with harmonic imaging, and measurements of LV volumes and EF have been shown to correlate more closely with those obtained from radionuclide, magnetic resonance, and computed tomographic methods.
Both pharmacologic and exercise stress echocardiography have been shown to have high sensitivity and specificity for the diagnosis of coronary artery disease, but they mainly rely on the subjective assessment of wall thickening. As such, their accuracy is highly dependent on image quality. Complete visualization of every LV endocardial border is required to confidently document or exclude abnormalities of regional myocardial wall thickening. Studies may be nondiagnostic in up to 30% of patients because of challenges with image acquisition from tachycardia, tachypnea, body habitus, and other causes. With current agents and imaging methods, adequate endocardial border resolution can be obtained in up to 95% of patients at peak stress. LV opacification during stress echocardiography improves reader confidence and the accuracy of this technique for detecting coronary artery disease, resulting in less downstream resource use and greater cost-effectiveness.
The evaluation of regional cardiac function by echocardiography can be used to triage patients with acute chest pain. The presence of a new resting regional wall motion abnormality (RWMA) has a high sensitivity for detecting cardiac ischemia. Those with RWMA were 6.1 times more likely to have cardiac death, acute myocardial infarction, unstable angina, congestive heart failure, or revascularization within 48 hours of presentation ( P < .0001), and an abnormal echocardiogram was an independent and more useful prognostic indicator than clinical evaluation and elecrocardiogram (ECG) findings. Conversely, patients with normal wall motion have a primary event (nonfatal acute myocardial infarction or total mortality) rate of only 0.4%. In comparison, 2.3% of patients discharged from the emergency department after a routine evaluation may have an acute myocardial infarction.
Delineation of Intracardiac Pathology
Ultrasound contrast agents are particularly helpful for defining pathologic findings in the near field, such as at the LV apex. Apical abnormalities may be difficult to delineate clearly because of near-field noise and the lack of good harmonic signals in the near field. Contrast can facilitate the identification and assessment of intracardiac masses such as tumors and apical thrombi, apical hypertrophy, isolated LV noncompaction, and apical aneurysms or pseudoaneurysms, among others.
Figure 24.1 shows an example of a pedunculated thrombus at the apex (see Fig. 24.1 , A ), which appears as a “filling defect” protruding into the LV cavity. The typical clinical context is a patient with a cardiomyopathy or an associated apical wall thickening abnormality. Occasionally, a sessile or laminated thrombus may be difficult to differentiate from a mass or tumor. In these situations, changing imaging settings to evaluate myocardial perfusion could help differentiate a thrombus from a benign or malignant neoplasm. A thrombus would be completely avascular and demonstrate no contrast enhancement; masses that are brighter than the surrounding myocardium (or hyperenhanced) suggest a highly vascular or malignant tumor; and stromal tumors (such as myxomas, lipomas, or fibromas) have a poor blood supply and appear hypoenhanced. Figure 24.2 shows images from a patient with hypereosinophilia and endomyocardial fibroelastosis who has an unusually “thick” apex delineated on an LV opacification image (see Fig. 24.2 , A ). In Figure 24.2 , B, the myocardium and LV cavity are both enhanced with contrast, revealing an obliterative apical thrombus, which appears as a stark filling defect juxtaposed against the contrast-enhanced myocardium and LV cavity.


Figure 24.3 shows images from a patient with a nonischemic cardiomyopathy who was diagnosed with an apical thrombus (see Fig. 24.3 , A, arrowheads ) and treated with anticoagulants; but subsequent contrast-enhanced images revealed that the correct diagnosis (as well as the cause of the patient’s LV dysfunction) was isolated LV noncompaction (see Fig. 24.3 , B ). The prominent trabeculations and deep intertrabecular recesses are clearly demarcated by the ultrasound contrast agent.

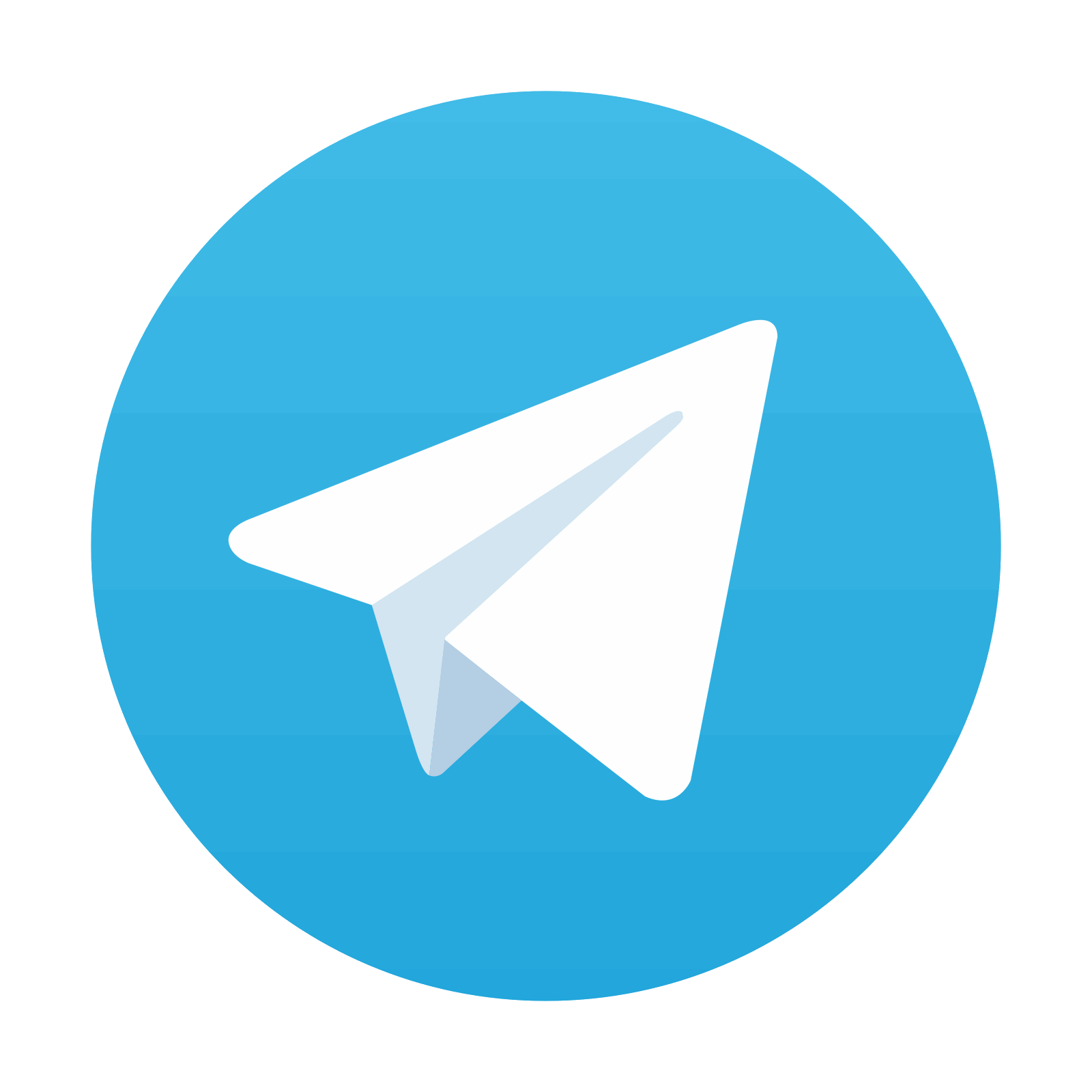
Stay updated, free articles. Join our Telegram channel

Full access? Get Clinical Tree
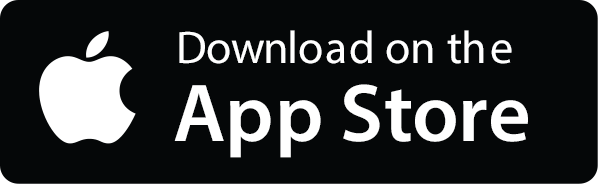
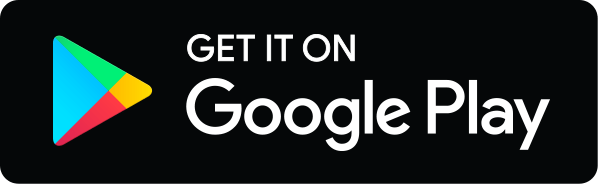
