Charles D. Fraser III1, Trevor A. Ellison2, Duke E. Cameron3, and Luca A. Vricella4 1Dell Children’s Hospital, Austin, TX, USA 2Mount Carmel Health System, Columbus, OH, USA 3The Massachusetts General Hospital, Boston, MA, USA 4University of Chicago, Chicago, IL, USA The genetic basis for heart and vascular conditions is heterogeneous and includes both inheritable and de novo mutations. More than 100 genes associated with congenital or progressive cardiovascular abnormalities have thus far been identified. In recent years, there has been increased awareness and focus on connective tissue diseases (CTDs) and associated cardiovascular pathology, particularly in children. These diseases are hereditary disorders of the connective tissues of the body [1]. Connective tissues are biologic tissues with an extracellular matrix (ECM) that serves to support and bind structures and organs across numerous systems. The ECM is a highly organized multimolecular structure that is essential for the normal functioning of organ systems [2]. Mutations affecting vascular ECM structure commonly manifest as arterial tortuosity and aneurysm formation, with a risk of subsequent vascular (i.e., aorta and carotid artery, among others) dissection and/or rupture. Furthermore, many CTDs are also associated with structural heart defects, including patent arterial duct (PAD), bicuspid aortic valve (BAV), coarctation of the aorta (CoA), and atrioventricular valve disease, among others. Children with connective tissue disorders are prone to aortic dissection, aneurysm, and aortic rupture [3–6]. In fact, aortic catastrophic events are the primary cause of morbidity and mortality in the most common CTDs. While the incidence of cardiovascular anomalies varies by disease type, mortality following aortic catastrophe in all these patients is exceptionally high, with up to 40% of pediatric patients dying immediately, and 1–3% expiring every hour in the first 24 hours following the initial event. Should the child reach the operating room alive, operative mortality as high as 25% has been reported [3]. In response to these devastating statistics, various medical and surgical strategies have been developed to mitigate the risk of cardiovascular catastrophe by means of prophylactic intervention. Advances in genetic analysis have had a significant impact on the identification and management of children with connective tissue disorders, providing a better understanding of their etiology and phenotypes, improving management strategies, and offering insights into long‐term prognosis [1]. In this chapter, we provide a description of the most common CTDs that affect the pediatric population and their associated cardiovascular manifestations. We will focus on the most common forms of cardiovascular phenotype (namely proximal aortic pathology and mitral valve disease) to define surgical indications and operative management. The most common CTDs affecting the cardiovascular system include Marfan syndrome (MFS), Loeys–Dietz syndrome (LDS), Ehlers–Danlos syndrome (EDS), osteogenesis imperfecta (OI), and other nonsyndromic conditions, such as familial thoracic aortic aneurysmal disease. All CTDs carry varying degrees of risk for aortic dissection and other cardiovascular pathology and, as such, require close surveillance and management to avert the risk of cardiovascular catastrophe. Patients with CTDs and proximal aortic aneurysms will often require aortic root replacement to prevent aortic dissection or rupture, often warranting surgical intervention at an early age. Although children and young adults with bicuspid aortic valves, conotruncal abnormalities, Turner syndrome, and other anomalies may necessitate surgical replacement of the proximal aorta, they do not fall into the CTD syndromes described herein. First described in 1896 [7], MFS is an inherited disorder resulting from mutations in the fibrillin 1 gene (FBN1) and most commonly affects the ocular, skeletal, and cardiovascular systems [8, 9]. Although the syndrome is most commonly inherited in an autosomal dominant pattern, approximately 25% of cases result from de novo mutations [9]. MFS has a prevalence of 1 in 5000–10,000 [9]. The syndrome is characterized by a high degree of clinical variability, although ocular, cardiovascular, and skeletal manifestation are the true hallmarks of this disorder. Up to 90% of patients with a clinical diagnosis of MFS have mutations in FBN1, a gene that codes for fibrillin‐1 [1]. Fibrillin‐1 is a structural component of ECM microfibrils that provides mechanical stability and critical elastic properties to connective tissues [10]. Furthermore, more recent studies suggest that the fibrillin‐deficient state of MFS leads to upregulation of the effects of the cytokine transforming growth factor beta (TGFβ), which in turn results in dysregulation of this signaling cascade. This derangement in signaling is thought to be responsible for the diverse and variable phenotypic expression of the disease [2]. Several hundred FBN1 mutations responsible for altered fibrillin‐1 structure have been reported, although no major correlation between the specific mutation and subsequent phenotypic manifestations have been identified. Furthermore, clinical variability exists even in patients with identical genotypic mutations, suggesting a potential role of modifier genes in the phenotypic expression of MFS [2]. The clinical diagnosis of MFS is based on the revised Ghent criteria (Table 38.1) [11]. These diagnostic criteria place a greater emphasis on cardiovascular manifestations than was previously described. The cardinal features of MFS based on the Ghent nosology are aortic root aneurysms and ectopia lentis. In the absence of family history, the presence of these two clinical manifestations is per se sufficient for the diagnosis of MFS. In the absence of either aortic root aneurysm or ectopia lentis, the presence of an FBN1 mutation or in combination with a systemic score ≥7 is sufficient for diagnosis [10, 11]. With a positive family history, an isolated finding of ectopia lentis, aortic root enlargement, or a systemic score ≥7 suffices for diagnosis [11]. Cardiovascular pathology is the leading cause of morbidity and early mortality in MFS [1, 12]. The most prominent cardiovascular manifestations of MFS are mitral valve prolapse and aortic dilatation. Mitral valve prolapse in MFS is thought to develop as a result of fibromyxomatous changes in the leaflets and chordae tendineae, calcification of the annulus, abnormal annular compliance, and distensibility [13]. These abnormalities result in important leaflet prolapse associated with mitral regurgitation (MR) and, in severe cases, rupture of chordae tendineae [14]. Mitral valve pathology is the most common structural heart pathology encountered in MFS, particularly in young children; tricuspid valve prolapse, dilatation of the proximal pulmonary artery, supraventricular arrhythmias, and impaired systolic and diastolic left ventricular function are however not uncommon [1, 2]. Table 38.1 Criteria for Marfan syndrome diagnosis from revised Ghent criteria. Ao, aortic diameter at the sinuses of Valsalva above the indicated z‐score or aortic dissection; MFS, Marfan syndrome. Source: Adapted from Loeys BL et al. J Med Genet. 2010;47:476–485. FBN1 mutations lead to arterial aneurysm formation, particularly of the ascending aorta. Although the walls of the entire arterial tree are weakened, dilatation most commonly occurs at the sinuses of Valsalva [1, 2, 13]. As the weakened proximal aorta dilates, aortic valve leaflet coaptation diminishes and central regurgitation ensues. Eccentric regurgitation is seen when asymmetric enlargement of the root or cusp prolapse develops. These changes in the proximal aorta make aortic pathology the leading cause of mortality in MFS [13]. While most patients with MFS present in late childhood or adolescence, a more severe form of MFS (infantile Marfan syndrome) has been described [15]. Infantile MFS typically presents with early onset of cardiovascular disease as well as severe skeletal manifestations, chest wall deformity, arachnodactyly, hyperextensible joints, micrognathia, and various forms of ocular pathology [15]. The most salient and life‐threatening feature of infantile MFS is its aggressive vascular phenotype. As many as 61% of infants with this syndrome will have substantial cardiac abnormalities, with the most common being mitral valve prolapse and annular enlargement. These can in turn result in severe congestive heart failure, with failure to thrive and ventilator dependency [15]. In early series investigating infantile MFS, mitral valve prolapse was identified on echocardiography in the majority of these children, with almost all cases progressing to various degrees of MR. Substantial proximal aortic root dilatation will also be manifest in 30% of these children [15]. Nonsurgical cardiovascular management of MFS typically focuses on close follow‐up of the vascular tree aimed at monitoring aortic diameters and prevention of dissection or rupture. In addition, the routine, long‐term, nonsurgical management strategy of MFS typically includes angiotensin‐receptor antagonists (such as Losartan) and beta blockers. Longitudinal imaging, afterload reduction, and congestive heart failure management are the cornerstones of medical therapy of mitral valve regurgitation, which remains the most common structural abnormality in these patients [6, 13]. Guidelines for cardiovascular surveillance, indications for surgical intervention, and clinical follow‐up strategies will be discussed below. Over the past five decades, the lifespan of patients with MFS has markedly improved, largely due to advances in both medical and surgical management of the cardiovascular manifestations of the disease [16]. With appropriate medical and, when necessary, surgical intervention, the life expectancy of patients with MFS approaches that of the general population. However, this improvement in outcome is maximized when the patient receives comprehensive cardiovascular care by a multidisciplinary team with extensive knowledge and experience in the care of patients with CTDs [1]. LDS is an autosomal dominant CTD characterized by aortic aneurysms and generalized arterial tortuosity, hypertelorism, and bifid/broad uvula or cleft palate [17–19]. Although the clinical features of this disorder share some similarities with MFS, LDS is caused by mutations in the genes encoding the TGFβ receptor 1 (TGFBR1) or 2 (TGFBR2) [16, 20]. Additional syndromic features may include craniosynostosis, Chiari malformation, club feet, PAD, and, most importantly, the potential for aneurysmal enlargement or dissection throughout the arterial tree [2]. In contrast to MFS, LDS less commonly presents with long bone overgrowth or lens dislocation. Additionally, aortic aneurysms in LDS tend to have accelerated growth when compared to those observed in MFS. As a consequence of this highly malignant vascular phenotype, children with LDS tend to present for surgical intervention at a younger age. LDS results from mutations in the mother against the decapentaplegic homolog 3 (SMAD3) gene and the TGFβ 2 ligand gene (TGFB2), which lead in turn to anomalies of TGFBR1 and/or TGFBR2. Chromosome deletions are responsible for these malformations, and the size of the microdeletion is thought to correlate with the broad spectrum of clinical presentation in LDS [17]. Although guidelines for management and treatment are similar across all types, significant clinical variability exists between the four types of LDS. The main organ systems affected in LDS include skeletal, craniofacial, cutaneous, and cardiovascular. Indications for surgical intervention will be detailed in subsequent sections but, when compared to MFS, arterial dissection can occur at diameters implying the need for earlier surgical intervention in LDS [1, 2]. Arterial tortuosity is observed through the entire arterial tree, and resulting complications may occur at any location. As a result, frequent and comprehensive surveillance as well as early surgical intervention are warranted. As mentioned above, LDS patients were originally categorized into two types, depending on the prevalence and severity of craniofacial (type 1) or cutaneous features (type 2). However, more recently, four types of LDS have been described based on genotype. LDS types 1 and 2 have significant craniofacial anomalies and also the most severe cardiovascular manifestations of disease. Type 4 is the least severe form of LDS in terms of risk of cardiovascular catastrophe. The various subtypes of LDS, associated genetic mutations, and phenotype characteristics are summarized in Table 38.2. Rapidly progressive aortic aneurysmal disease is a distinctive feature of LDS [17]. Aortic dissection has been reported in children as young as 3 months [18]. In fact, the initial reports of LDS types 1 and 2 described a mean age of death of 26.1 years, with aortic dissection and cerebral hemorrhage as the primary causes of death [20]. Although improvements in diagnosis, surveillance, and early interventions have substantially improved the life span of affected individuals, the severity of the cardiovascular manifestations of LDS cannot be overstated. Congenital heart defects such as BAV, atrial septal defects, and PAD are more commonly seen in children with LDS types 1 and 2 when compared to the general population. In addition to mitral valve prolapse and dysfunction, pulmonary root dilation and tricuspid valve regurgitation have been observed. Of note is that children with LDS may require aortic or mitral valve interventions even without the presence of aortic root dilatation because of the severity of structural valve disease [17]. Atrial fibrillation, ventricular hypertrophy, ventricular arrhythmias, and heart failure have also been described. Table 38.2 Loeys–Dietz syndrome (LDS) subgroup classification system. In view of the vast cardiovascular expressions of disease, individuals diagnosed with LDS require echocardiography at frequent intervals (every 6–12 months) to monitor the status of heart valves, aortic root, and ascending aorta [17]. Additionally, frequent imaging with magnetic resonance angiography (MRA) or computed tomographic angiography (CTA) with three‐dimensional reconstruction is recommended to monitor the entire arterial tree for aneurysmal enlargement and dissection. Although MRA and CTA may expose the patient to ionizing radiation and anesthesia, serial imaging is critical to prevention of cardiovascular catastrophe. Many advocate for surveillance CTA or MRA every 1–2 years depending on the severity of the disease. Cameron and colleagues reported that 7/21 (33%) of their originally reported surgical cohort of LDS 1 and 2 required multiple vascular surgical interventions, highlighting the need for judicious, life‐long surveillance [18]. Management of children with LDS mirrors that of MFS and is summarized in Table 38.3. However, given the aggressive nature of the disease, thresholds for intervention tend to be lower than with MFS. Well‐defined guidelines for surgical intervention are limited, and vary slightly based on the type of LDS and the severity of disease. Aortic dissections have been reported in individuals with maximal aortic diameters of less than 4.0 cm in LDS types 1, 2, or 3. Therefore, given the aggressive nature of the disease and relatively low rate of complications with proximal aortic surgery in experienced centers, aortic root replacement is indicated when the root diameter reaches a threshold of 4.0 cm for LDS types 1 and 2. In children with LDS type 3, which is caused by mutations in SMAD3 and is moderately aggressive, aortic root replacement is recommended for aneurysms between 4.0 and 4.5 cm in size. LDS type 4 is the mildest phenotype and therefore the threshold for intervention is slightly higher at 4.5 cm. Additionally, aneurysmal growth of greater than 0.5 cm/year warrants surgical intervention. However, regardless of type in these children, those with a concerning family history of aortic catastrophe and severe craniofacial features may warrant earlier intervention [21]. Table 38.3 Guidelines for routine clinical care in children with Loeys–Dietz syndrome. EDS is most commonly inherited in an autosomal dominant pattern, with an estimated prevalence of 1 in 5000 [22]. Historically, the Villefranche nosology has been widely used as the standard clinical classification system for EDS and described the six major subtypes of the syndrome [11, 23]. In 2017, given the extensive research in CTDs as well as the advances in gene sequencing, a new classification system was developed to include newly defined subtypes of EDS [23]. In the 2017 classification system, 13 subtypes have been recognized, maintaining a clinical classification system to define subtypes by phenotype. All subtypes include the basic clinical hallmarks of EDS, which include joint hypermobility, skin hyperextensibility, and tissue fragility. Vascular EDS, also known as type IV EDS, result from mutations in the COL3A1 gene, which affects type III collagen synthesis and is inherited in an autosomal dominant pattern. Characteristic features of vascular EDS include thin, translucent skin, characteristic facial appearance (large eyes, small chin, sunken cheeks, thin nose and lips, lobeless ears), vascular fragility demonstrated by extensive bruising, easy bleeding, and spontaneous arterial, intestinal, or uterine rupture [1, 3, 22, 24, 25]. Cardiovascular manifestations of EDS include arterial aneurysms at any location throughout the arterial tree, mitral valve dysfunction, and venous malformations. The diagnosis of type IV EDS is based on clinical findings and confirmed by genetic analysis for the causative mutation or by identification of abnormal type III collagen synthesis. Major criteria for diagnosis include a family history of vascular EDS with a documented causative variant in COL3A1, arterial rupture or dissection at a young age (<40 years), spontaneous colonic perforation, uterine rupture during the third trimester in the absence of previous Cesarean section, and carotid‐cavernous sinus fistula formation in the absence of trauma [23].
CHAPTER 38
Connective Tissue Disorders
Diagnostic Syndromes and Associated Heart Disease
Marfan Syndrome
In the absence of family history:
(1) Ao Z‐Score ≥2 AND Ectopia Lentis = MFS
(2) Ao Z‐Score ≥2 AND Fibrillin‐1 Mutation = MFS
(3) Ao Z‐Score ≥2 AND Systemic Score ≥7 = MFS
(4) Ectopia Lentis AND Fibrillin‐1 Mutation = MFS
In the presence of family history:
(5) Ectopia Lentis and family history of MFS = MFS
(6) Systemic Score ≥7 and family history of MFS = MFS
(7) Ao Z‐Score ≥2 (above 20 years old), ≥3 (below 20 years old) and family history of MFS = MFS
Loeys–Dietz Syndrome
LDS subtype
Gene
Other disorders reported
1
TGFBR1
Thoracic aortic aneurysm and dissection
2
TGFBR2
Thoracic aortic aneurysm and dissection, Marfan syndrome type 2
3
SMAD3
Aneurysms‐osteoarthritis syndrome
4
TGFB2
Aortic and cerebral aneurysm, arterial tortuosity, and skeletal manifestations
Yearly echocardiography; shorter intervals depending on the extent of aortic disease
Angiotensin‐receptor blockade, beta blocker, or angiotensin‐converting enzyme inhibitor and strict blood pressure control
Avoidance of contact/competitive sports, isometric or strenuous exercise, and trauma to head/chest
Avoidance of stimulants and vasoconstrictors
Subacute bacterial endocarditis prophylaxis in those with artificial valves
Cardiac surgery consultation when surgical thresholds for intervention are approaching
Ehlers–Danlos Syndrome
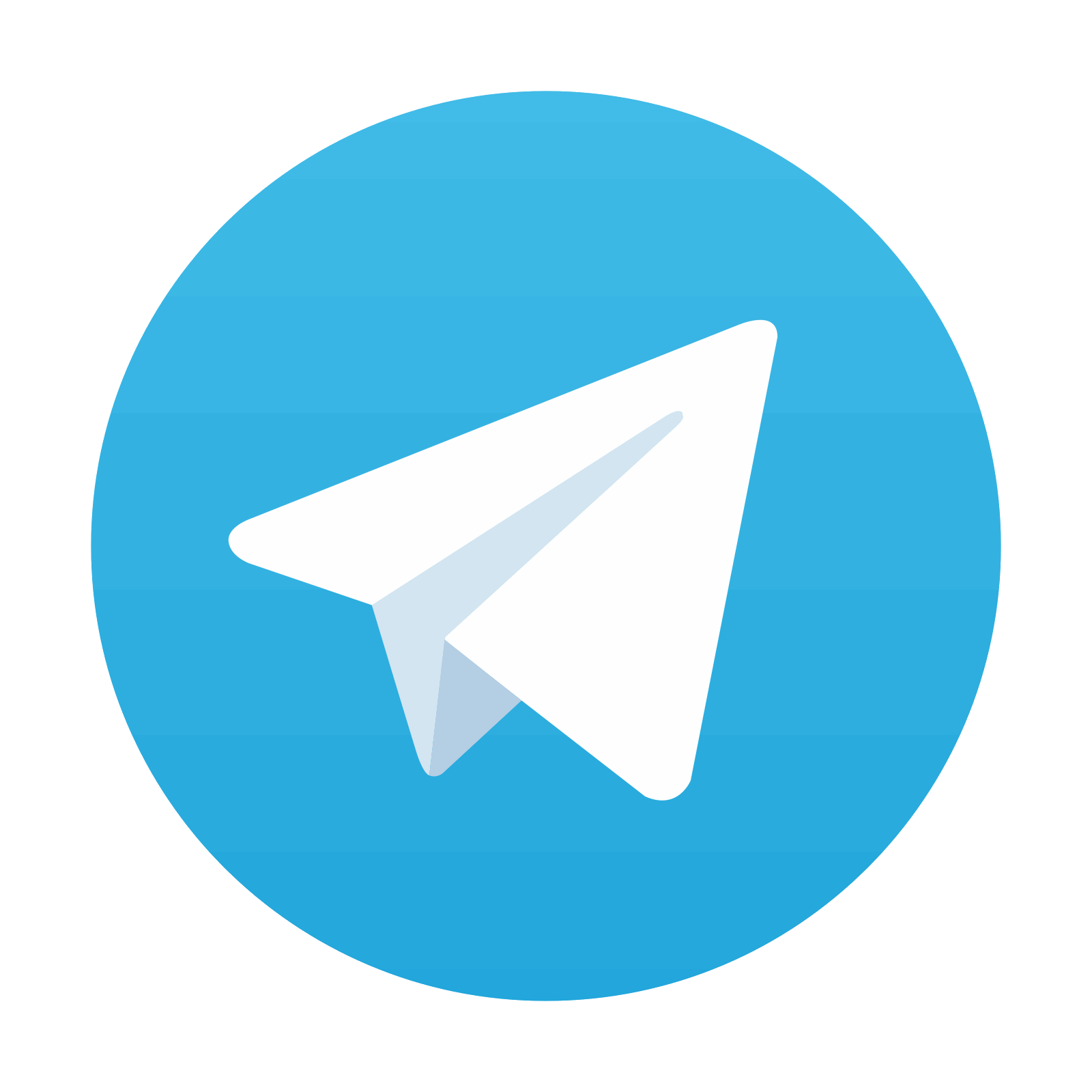
Stay updated, free articles. Join our Telegram channel

Full access? Get Clinical Tree
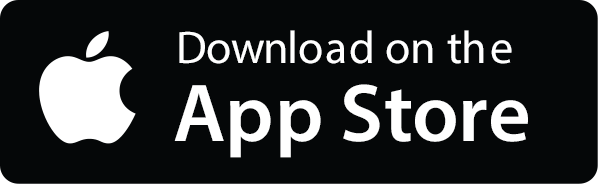
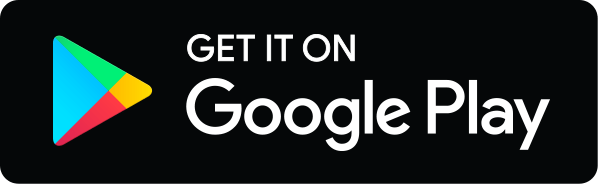