Real-time 3-dimensional (3D) transesophageal echocardiography (TEE) provides more accurate geometric information on the mitral valve (MV) than 2-dimensional (2D) TEE. The aim of this study was to quantify MV prolapse using real-time 3D TEE in patients with severe mitral regurgitation. In 102 patients with severe mitral regurgitation due to MV prolapse and/or flail, 2D TEE quantified MV prolapse, including prolapse gap and width in the commissural view. Three-dimensional TEE also determined prolapse gap and width with the use of the 3D en face view. On the basis of the locations of MV prolapse, all patients were classified into group 1 (pure middle leaflet prolapse, n = 50) or group 2 (involvement of medial and/or lateral prolapse, n = 52). Prolapse gap and prolapse width determined by 3D TEE were significantly greater than those by 2D TEE (all p values <0.001). The differences in prolapse gap and prolapse width between 2D TEE and 3D TEE were significantly greater in group 2 than group 1 (Δ gap 1.3 ± 1.4 vs 2.4 ± 1.8 mm, Δ width 2.5 ± 3.0 vs 4.4 ± 5.1 mm, all p values <0.01). The differences in prolapse gap and width between 2D TEE and 3D TEE were best correlated with 3D TEE–derived prolapse width (r = 0.41 and r = 0.74, respectively). Two-dimensional TEE underestimated the width of MV prolapse and leaflet gap compared to 3D TEE. Two-dimensional TEE could not detect the largest prolapse gap and width, because of the complicated anatomy of the MV. In conclusion, 3D TEE provided more precise quantification of MV prolapse than 2D TEE.
Mitral valve (MV) prolapse is a relatively common disease in clinical practice, and the estimated prevalence rates vary from 0.6% to 2.4%. Conventional 2-dimensional (2D) echocardiography with Doppler capability is a widely accepted diagnostic tool for the assessment of MV disease. However, the complex anatomy of the MV apparatus exposes the limitations of this routine technique. A high level of expertise is also required for the accurate interpretation of 2D images. As surgical and catheter techniques improve over time, more detailed quantification of MV prolapse is being required. Recently introduced real-time 3-dimensional transesophageal echocardiography (TEE) can provide more accurate geometric information on the MV than 2D TEE. Earlier studies have reported the feasibility and accuracy of 3D TEE for identifying the locations of MV prolapse. However, the quantification of MV prolapse using 3D TEE relative to 2D TEE has not been fully elucidated in these studies. Therefore, in this study, we aimed to (1) quantify MV prolapse using 3D TEE, and (2) compare the quantification with 2D TEE to that with 3D TEE.
Methods
This study consisted of 125 consecutive patients with severe mitral regurgitation (MR) caused by MV prolapse and/or flail who were clinically referred for TEE from January to November 2010. All patients underwent complete 2D TEE and 3D TEE on the same day. Patients with histories of MV replacement (n = 3 [2.4%]), atrial fibrillation (n = 11 [8.8%]), irregular heart rhythm (n = 1 [0.8%]), and significant mitral stenosis (n = 2 [1.6%]) were excluded from this study. Patients with poor-quality images (n = 3 [2.4%]) and those whose prolapse gap could not be measured (n = 3 [2.4%]) were also excluded. Finally, 102 patients were enrolled. This study was approved by the institutional review board of the Cedars-Sinai Medical Center.
TEE was performed using an iE33 ultrasound imaging system (Philips Medical Systems, Andover, Massachusetts) equipped with a fully sampled matrix-array TEE transducer that can display 2D and live 3D images. All patients underwent TEE in the left lateral decubitus position. Complete 2D, color, pulsed-wave, and continuous-wave Doppler images were obtained for assessing cardiac structures and function. Multiplane 2D transesophageal echocardiographic evaluation included the complete standard protocol for the evaluation of the MV, allowing complete description of all segments of the valve. The Carpentier nomenclature was applied to the locations of MV prolapse (anterior leaflet: A1, A2, and A3 for the lateral, middle, and medial scallops, respectively; posterior leaflet: P1, P2, and P3 for the lateral, middle, and medial scallops, respectively). Two-dimensional prolapse gaps were measured quantitatively from the 4- or 5-chamber view obtained at the midesophageal level with 0° and from the 3-chamber view obtained at the midesophageal level with 120° to 160° using 2D TEE ( Figure 1 ) in each patient. Of these, the largest 2D prolapse gap was adopted in this study. Two-dimensional prolapse width was determined quantitatively from the transcommissural view at the midesophageal level with 45° to 80° using 2D TEE ( Figure 1 ). Prolapse gap and width were quantified in the end-systolic frame. The severity of MR was determined quantitatively from the 4-chamber view obtained at the mid esophageal level with 0° using 2D TEE. Vena contracta width was measured as the narrowest portion of MR color Doppler jet. Effective regurgitant orifice area was also calculated using the following formula by the proximal isovelocity surface area method: effective regurgitant orifice area = 2π × R PISA 2 × V aliasing /V max , where R PISA is the maximal proximal isovelocity surface area radius (centimeters), V aliasing is the aliasing velocity of the proximal flow convergence (centimeters per second), and V max is the maximal velocity of the continuous-wave Doppler MR signal (centimeters per second). Vena contracta width ≥0.3 cm and effective regurgitant orifice area ≥0.2 cm 2 were considered significant (equal to or greater than moderate) according to current recommendations.
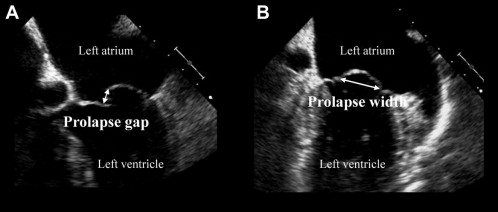
The probe was positioned at the midesophageal level for scanning 3D images. Three-dimensional TEE of the MV was performed, equipped with a fully sampled matrix-array transducer (X7-2t). Initially, gain settings were optimized using narrow-angle acquisition mode, which allows 3D TEE of a pyramidal volume of approximately 30° × 60°. Zoomed 3D transesophageal echocardiographic images of the entire MV or gated full-volume sets were then acquired in a single (31 patient [30.4%]) or 4 consecutive (71 patients [69.6%]) cardiac cycles (frame rate 15 ± 7 frames/s, range 6 to 31). All 3D transesophageal echocardiographic data were digitally stored for off-line analysis (QLAB Cardiac 3DQ; Philips Medical Systems). The end-systolic frame was defined as the second to last frame preceding MV opening. Then, using multiplanar reconstruction of the 3D transesophageal echocardiographic volume set, a cross-sectional plane through the MV leaflets was selected and the maximum MV prolapse gap, and width were searched with the movement of 2D planes in the 3D space. Then, prolapse gap and prolapse width were measured ( Figure 2 ).
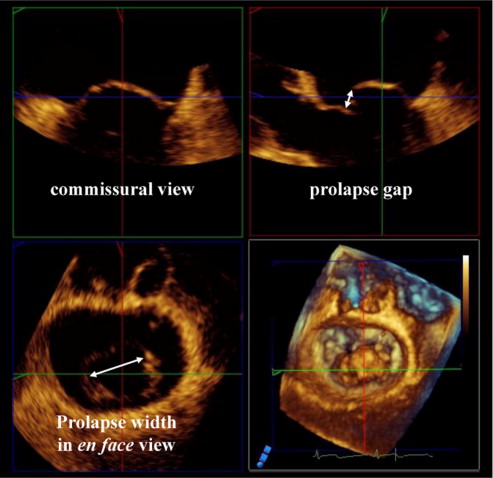
All patients underwent complete 2D echocardiographic and Doppler examinations. Left ventricular end-diastolic volume and end-systolic volume were measured according to the Simpson’s biplane method. The left ventricular ejection fraction was calculated as [(end-diastolic volume − end-systolic volume)/end-diastolic volume] × 100. From the transmitral inflow velocities, the following variables were measured: peak velocity of early diastolic filling (E velocity), late filling with atrial contraction (A velocity), E/A ratio, and the deceleration time of the E wave. With continuous-wave Doppler, the maximum peak tricuspid regurgitant velocity recorded from any view was used to determine pulmonary artery systolic pressure with the simplified Bernoulli’s equation (pulmonary artery systolic pressure = 4 × [peak velocity] 2 + mean right atrial pressure) ; mean right atrial pressure was estimated according to the most recent guidelines from the American Society of Echocardiography.
All values are expressed as mean ± SD. An unpaired Student’s t test was performed to compare group 1 and group 2. A paired Student’s t test was used to compare 2D and 3D MV parameters. Differences were considered significant at p <0.05. The linear regression analysis was used for correlation of variables of interest. Bland-Altman plots were used to evaluate differences in prolapse gap and width by 2D TEE and 3D TEE. Interobserver and intraobserver variability for MV prolapse gap and width measured using 2D TEE and 3D TEE was obtained by the analysis of 10 random images by 2 independent blinded observers and by the same observers at 2 different time points. The results were analyzed using intraclass correlation coefficients and the Bland-Altman method. Statistical analyses were performed using SPSS version 17.0 (SPSS, Inc., Chicago, Illinois).
Results
This study included 73 men and 29 women (mean age 65.8 ± 13.8 years). Table 1 lists anatomic characteristics diagnosed using 3D TEE. All patients were stratified into group 1 (pure middle scallop prolapse [P2 and/or A2], n = 50) or group 2 (involvement of medial and/or lateral scallop prolapse, n = 52) on the basis of the locations of MV prolapse. The mean left ventricular end-diastolic volume was 121 ± 43 ml, the mean left ventricular end-systolic volume was 44 ± 22 ml, and the mean left ventricular ejection fraction was 65 ± 10%. Two-dimensional prolapse gap was significantly correlated with 3D prolapse gap (r = 0.82, p <0.001; Figure 3 ), while 2D prolapse width was significantly correlated with 3D prolapse width (r = 0.86, p <0.001; Figure 3 ). Prolapse gap and width measured by 2D TEE were underestimated compared to those measured by 3D TEE (gap 4.4 ± 2.7 vs 6.1 ± 3.0 mm, width 13 ± 5 vs 16 ± 7 mm, all p values <0.001). The mean differences in prolapse gap and width between 2D TEE and 3D TEE were −1.9 ± 1.7 and −3.6 ± 4.1 mm, respectively ( Figure 4 ).
Variable | n (%) |
---|---|
Isolated P1 | 5 (5) |
Isolated P2 | 40 (39) |
Isolated P3 | 8 (8) |
Isolated A1 | 0 (0) |
Isolated A2 | 3 (3) |
Isolated A3 | 1 (1) |
Posterior leaflet (>1 scallop) | 19 (19) |
Anterior leaflet (>1 scallop) | 5 (5) |
Bileaflet | 21 (21) |
Table 2 lists the comparison of echocardiographic findings between group 1 and group 2. No differences in left ventricular end-diastolic volume, left ventricular end-systolic volume, the left ventricular ejection fraction, vena contracta width, pulmonary artery systolic pressure, or MV prolapse gap were found between the 2 groups. Two-dimensional and 3D prolapse widths were significantly greater in group 2 than those in group 1 (2D 11 ± 4 vs 14 ± 6 mm, 3D 14 ± 5 vs 19 ± 9 mm, all p values <0.001). The differences in MV prolapse gap and width between 3D TEE and 2D TEE (Δ gap and Δ width) were significantly greater in group 2 than group 1 (Δ gap 1.3 ± 1.4 vs 2.4 ± 1.8 mm, p = 0.002; Δ width 2.5 ± 3.0 vs 4.4 ± 5.1 mm, p = 0.008; Figure 5 ).
Variable | Total | Group 1 ∗ | Group 2 † | p Value ‡ |
---|---|---|---|---|
(n = 102) | (n = 50) | (n = 52) | ||
Left ventricular end-diastolic volume (ml) | 121 ± 43 | 118 ± 38 | 127 ± 52 | 0.501 |
Left ventricular end-systolic volume (ml) | 43 ± 22 | 41 ± 19 | 48 ± 26 | 0.308 |
Left ventricular ejection fraction (%) | 64 ± 10 | 66 ± 8 | 59 ± 12 | 0.116 |
Left atrial area (mm 2 ) | 31 ± 12 | 30 ± 10 | 32 ± 14 | 0.627 |
E velocity (cm/s) | 127 ± 20 | 130 ± 25 | 124 ± 20 | 0.449 |
A velocity (cm/s) | 74 ± 14 | 68 ± 15 | 82 ± 14 | 0.332 |
E/A ratio | 1.7 ± 0.7 | 1.9 ± 0.8 | 1.5 ± 0.5 | 0.268 |
Deceleration time (ms) | 206 ± 52 | 199 ± 60 | 218 ± 44 | 0.277 |
Pulmonary artery systolic pressure (mm Hg) | 42 ± 12 | 43 ± 12 | 41 ± 11 | 0.634 |
Vena contracta width (cm) | 0.50 ± 0.15 | 0.49 ± 0.28 | 0.51 ± 0.16 | 0.599 |
Effective regurgitant orifice area (cm 2 ) | 0.31 ± 0.11 | 0.30 ± 0.10 | 0.32 ± 0.13 | 0.612 |
2D prolapse gap (mm) | 4.4 ± 2.7 | 4.8 ± 1.8 | 4.0 ± 2.5 | 0.163 |
2D prolapse width (mm) | 13 ± 5 | 11 ± 4 | 14 ± 6 | 0.003 |
3D prolapse gap (mm) | 6.1 ± 3.0 | 6.1 ± 2.9 | 6.0 ± 3.1 | 0.839 |
3D prolapse width (mm) | 16 ± 7 | 14 ± 5 | 19 ± 9 | 0.001 |
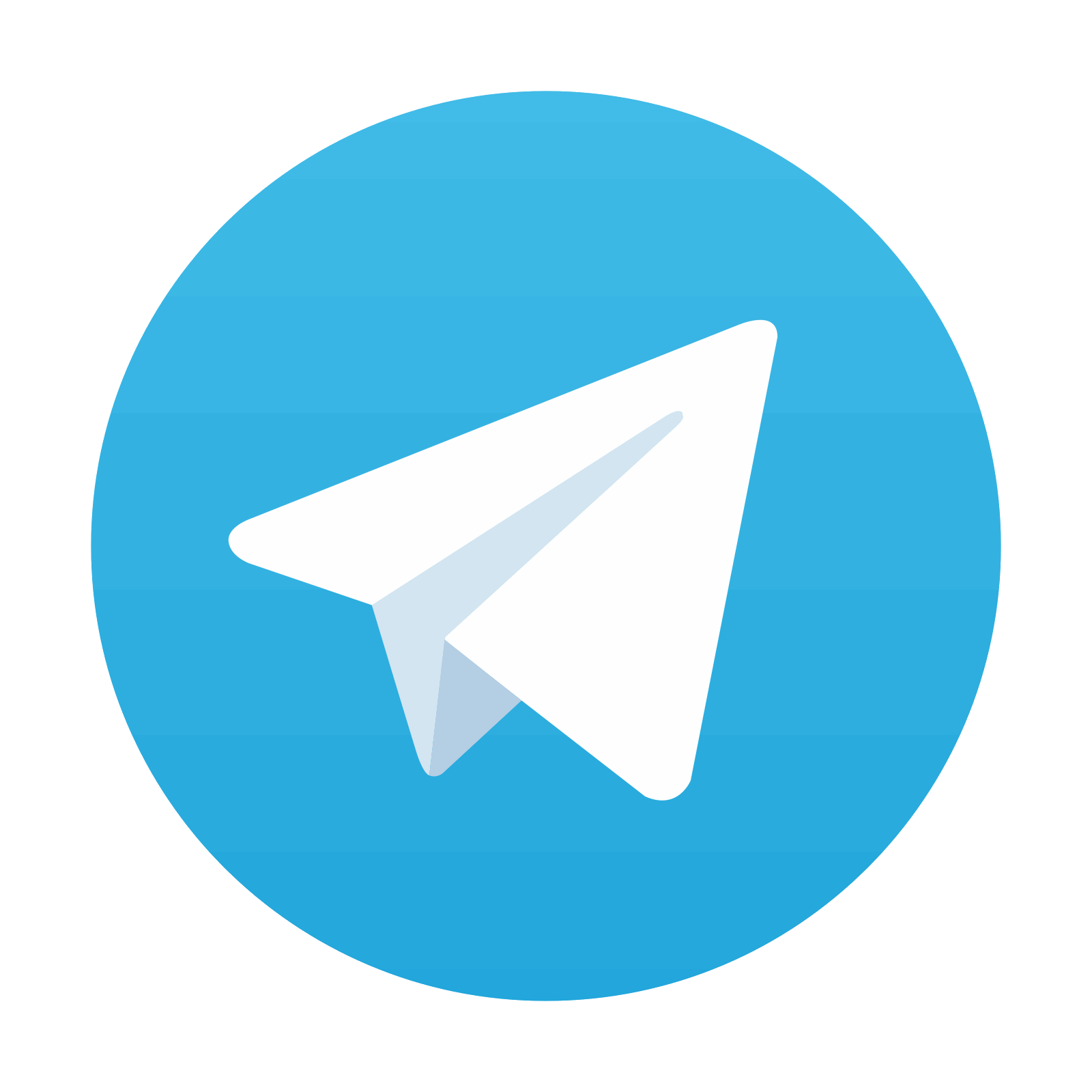
Stay updated, free articles. Join our Telegram channel

Full access? Get Clinical Tree
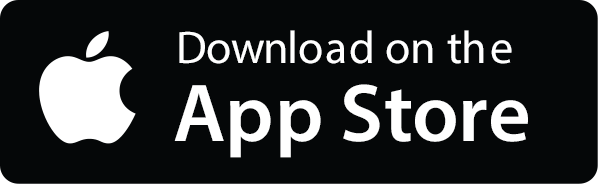
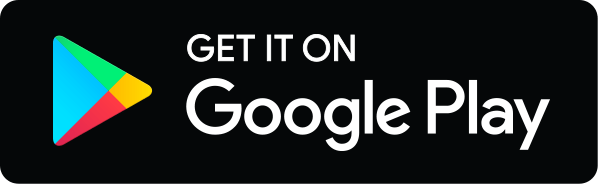
