Although swimming is one of the most popular, most practiced, and most recommended forms of physical activity, little information is available regarding the influence of regular swimming on vascular disease risks. Using a cross-sectional study design, key measurements of vascular function were performed in middle-aged and older swimmers, runners, and sedentary controls. There were no group differences in age, height, dietary intake, and fasting plasma concentrations of glucose, total cholesterol, and low-density lipoprotein cholesterol. Runners and swimmers were not different in their weekly training volume. Brachial systolic blood pressure and pulse pressure were higher (p <0.05) in swimmers than in sedentary controls and runners. Runners and swimmers had lower (p <0.05) carotid systolic blood pressure and carotid pulse pressure than sedentary controls. Carotid arterial compliance was higher (p <0.05) and β-stiffness index was lower (p <0.05) in runners and swimmers than in sedentary controls. There were no significant group differences between runners and swimmers. Cardiovagal baroreflex sensitivity was greater (p <0.05) in runners than in sedentary controls and swimmers and baroreflex sensitivity tended to be higher in swimmers than in sedentary controls (p = 0.07). Brachial artery flow-mediated dilation was significant greater (p <0.05) in runners compared with sedentary controls and swimmers. In conclusion, our present findings are consistent with the notion that habitual swimming exercise may be an effective endurance exercise for preventing loss in central arterial compliance.
Swimming can be an ideal mode of exercise for those at increased risk of vascular disease including elderly patients, obese patients, and patients with arthritis. Although swimming is widely promoted and recommended as a mode of aerobic exercise by national and international organizations, research focusing on influences of swimming on vascular disease risks is lacking. The aims of the present investigation were to determine (1) whether swimmers would demonstrate higher levels of key vascular function measurements (i.e., central artery compliance, arterial baroreflex sensitivity, and endothelial-dependent vasodilation) than sedentary controls and (2) if levels of such vascular measurements are different from runners who are matched for age and exercise training status.
Methods
We studied 75 apparently healthy middle-aged and older adults (37 to 75 years of age). They were swimmers, runners, or sedentary controls. All subjects were nonobese, nonsmoking, normotensive (<140/90 mm Hg), normolipidemic, and free of overt cardiovascular and other chronic diseases as assessed by medical history questionnaire, blood chemistry, and hematologic evaluation. None of the subjects were taking cardiovascular-acting medications including hormone replacement therapy. Physical activity status was verified by a modified physical activity questionnaire and maximal oxygen consumption. In average, runners and swimmers had been exercising 4.1 ± 2.2 times/week for 9 ± 2 years and 4.8 ± 1.1 times/week for 9 ± 2 years, respectively. Sedentary participants had been sedentary at least for the previous 12 months. All subjects gave their written informed consent to participate. The study was reviewed and approved by the institutional review board.
Before they were tested, subjects abstained from food, alcohol, and caffeine for ≥4 hours (overnight 12-hour fast for metabolic risk factors). Premenopausal women were tested during the early follicular phase of the menstrual cycle. All testing was performed 24 to 48 hours after the last exercise bout.
Body composition was assessed using dual-energy x-ray absorptiometry (Lunar DPX, GE Medical, Fairfield, Connecticut). A 3-day dietary intake record was obtained and analyzed by a registered dietitian using Nutritionist Pro software (Axxya Systems, Stafford, Texas). A blood sample was collected by venipuncture after an overnight fast. Fasting plasma concentrations of glucose, lipids, and lipoproteins were determined using a Vitros DT60 analyzer (Ortho-Clinical Diagnostics, Raritan, New Jersey). Graded exercise testing was undertaken using a metabolic cart during a modified Bruce protocol. After a 5-minute warm-up, subjects walked or ran while the treadmill slope was gradually increased 2% every 2 minutes until volitional exhaustion.
Bilateral brachial and ankle blood pressures, carotid and femoral pulse waves, and heart rate were measured by an automated vascular testing device (VP-2000, Omron Healthcare Bannockburn, Illinois) after a subject had been lying in a supine position for ≥15 minutes. Ankle–brachial pressure index was calculated as ankle systolic blood pressure divided by brachial systolic blood pressure. Carotid and femoral artery pulse waves were recorded by arterial applanation tonometry incorporating an array of 15 micropiezoresistive transducers placed on the carotid and femoral arteries. Time delay was measured automatically with the foot-to-foot method, and pulse wave velocity was subsequently calculated. Augmentation index, an index of arterial wave reflection, was obtained using an arterial tonometry placed on the carotid artery as previously described.
Arterial compliance and β-stiffness index were measured noninvasively by a combination of ultrasound imaging on the carotid artery and simultaneous applanation tonometry on the contralateral artery. A longitudinal image of the common carotid and femoral artery were acquired 1 to 2 cm proximal to the bifurcation using an ultrasound machine equipped with a high-resolution linear array transducer (Philips iE33 Ultrasound System, Philips, Bothel, Washington). All data were analyzed by the same investigator who was blinded to group assignment and used image analysis software (Vascular Research Tool Carotid Analyzer, Medical Imaging Applications, Coralville, Iowa). Pressure waveform and amplitude were obtained from the contralateral artery using arterial applanation tonometry (VP-2000, Omron Healthcare) and analyzed by waveform browsing software (WinDaq 2000, Dataq Instruments, Akron, Ohio).
Brachial artery flow-mediated dilation (FMD) was measured using a standard procedure as described previously. Briefly, a pneumatic blood pressure cuff was positioned 2 inches below the antecubital fossa. Brachial diameter and blood flow velocity were acquired from a Doppler ultrasound machine equipped with a high-resolution linear array transducer (Philips iE33 Ultrasound). After baseline images were obtained, the cuff was inflated to 100 mm Hg above a subject’s systolic blood pressure for 5 minutes. All ultrasound-derived blood flow and diameter data were analyzed by the same investigator using image analysis software (Brachial Analyzer, Medical Imaging Applications). FMD was calculated as (maximal artery diameter minus baseline artery diameter)/baseline artery diameter × 100.
Cardiovagal baroreflex sensitivity (BRS) was determined using the Valsalva maneuver as previously described. Subjects performed 3 Valsalva maneuvers by forcibly exhaling against a closed airway. Subjects were asked to maintain an expiratory mouth pressure of 40 mm Hg for 10 seconds. Data for cardiovagal BRS were recorded and analyzed by waveform browsing software (WinDaq 2000) during the phase IV overshoot. Systolic blood pressure values were linearly regressed against corresponding RR intervals from the point where the RR intervals began to lengthen to the point of maximal systolic blood pressure increase.
Analysis of covariance was used for statistical analysis to determine significant group differences. Correlation and regression analyses were performed to determine the relation between carotid arterial compliance and cardiovagal BRS. All variables were expressed as mean ± SEM.
Results
As presented in Table 1 , there were no group differences in age, height, and dietary intakes. Body mass and body mass index were lower (p <0.05) in runners than in sedentary controls and swimmers. Percent body fat of swimmers was lower (p <0.05) than of sedentary controls but higher (p <0.05) than of runners. Maximal oxygen consumption of swimmers was greater than of sedentary controls but lower than of runners. Fasting plasma concentrations of glucose, total cholesterol, and low-density lipoprotein cholesterol were not different among groups. Runners had significantly lower plasma triglyceride and higher high-density lipoprotein cholesterol concentrations than sedentary controls.
Variable | Sedentary | Runners | Swimmers |
---|---|---|---|
Men/women | 16/9 | 17/8 | 17/8 |
Age (years) | 54 ± 2 | 52 ± 2 | 56 ± 2 |
Height (cm) | 170 ± 2 | 173 ± 2 | 173 ± 2 |
Body mass (kg) | 74 ± 2 | 67 ± 2 ⁎ | 76 ± 2 † |
Body mass index (kg/m 2 ) | 26 ± 1 | 22 ± 1 ⁎ | 25 ± 1 † |
Body fat (%) | 30 ± 2 | 18 ± 2 ⁎ | 24 ± 2 ⁎ † |
Maximal oxygen consumption (ml/kg/min) | 31 ± 2 | 50 ± 2 ⁎ | 41 ± 2 ⁎ † |
Physical activity score (units) | 11 ± 4 | 58 ± 3 ⁎ | 57 ± 4 ⁎ |
Total caloric intake (kcal/day) | 2,370 ± 212 | 2,343 ± 169 | 2,160 ± 150 |
Carbohydrate intake (%) | 46 ± 3 | 49 ± 3 | 47 ± 2 |
Fat intake (%) | 36 ± 2 | 32 ± 2 | 33 ± 2 |
Protein intake (%) | 13 ± 1 | 14 ± 1 | 16 ± 1 |
Alcohol intake (%) | 5 ± 2 | 4 ± 1 | 3 ± 1 |
Sodium intake (mg/day) | 3,472 ± 348 | 2,968 ± 278 | 3,014 ± 246 |
Total cholesterol (mg/dl) | 193 ± 9 | 179 ± 9 | 194 ± 11 |
Low-density lipoprotein cholesterol (mg/dl) | 120 ± 8 | 105 ± 8 | 123 ± 9 |
High-density lipoprotein cholesterol (mg/dl) | 47 ± 3 | 61 ± 3 ⁎ | 52 ± 4 † |
Triglyceride (mg/dl) | 125 ± 14 | 70 ± 14 ⁎ | 97 ± 15 |
Plasma glucose (mg/dl) | 94 ± 3 | 95 ± 3 | 99 ± 3 |
Heart rate at rest was lower (p <0.05) in runners than in sedentary controls and swimmers ( Table 2 ). Brachial systolic blood pressure and pulse pressure were higher (p <0.05) in swimmers than in sedentary controls and runners. Runners and swimmers had lower (p <0.05) carotid systolic blood pressure and carotid pulse pressure than sedentary controls. Brachial ankle pulse wave velocity was significantly lower in runners than in swimmers and sedentary controls. There were no group differences in carotid artery augmentation index.
Variable | Sedentary | Runners | Swimmers |
---|---|---|---|
Heart rate (beats/min) | 60 ± 2 | 50 ± 2 ⁎ | 58 ± 2 † |
Systolic blood pressure (mm Hg) | 119 ± 3 | 119 ± 3 | 128 ± 3 ⁎ † |
Mean blood pressure (mm Hg) | 89 ± 2 | 88 ± 2 | 93 ± 2 |
Diastolic blood pressure (mm Hg) | 71 ± 1 | 72 ± 1 | 74 ± 2 |
Pulse pressure (mm Hg) | 48 ± 2 | 47 ± 2 | 54 ± 2 ⁎ † |
Carotid systolic pressure (mm Hg) | 116 ± 3 | 104 ± 3 ⁎ | 104 ± 3 ⁎ |
Carotid pulse pressure (mm Hg) | 47 ± 2 | 36 ± 2 ⁎ | 37 ± 2 ⁎ |
Brachial-ankle pulse wave velocity (cm/s) | 1,336 ± 36 | 1,230 ± 35 ⁎ | 1,334 ± 36 † |
Carotid augmentation index (%) | 15 ± 5 | 13 ± 4 | 13 ± 5 |
Carotid artery diameter at end-diastole (mm) | 6.77 ± 0.16 | 6.0 ± 0.15 ⁎ | 6.67 ± 0.15 † |
Brachial artery diameter at end-diastole (mm) | 4.2 ± 1.9 | 4.2 ± 1.8 | 4.6 ± 1.8 |
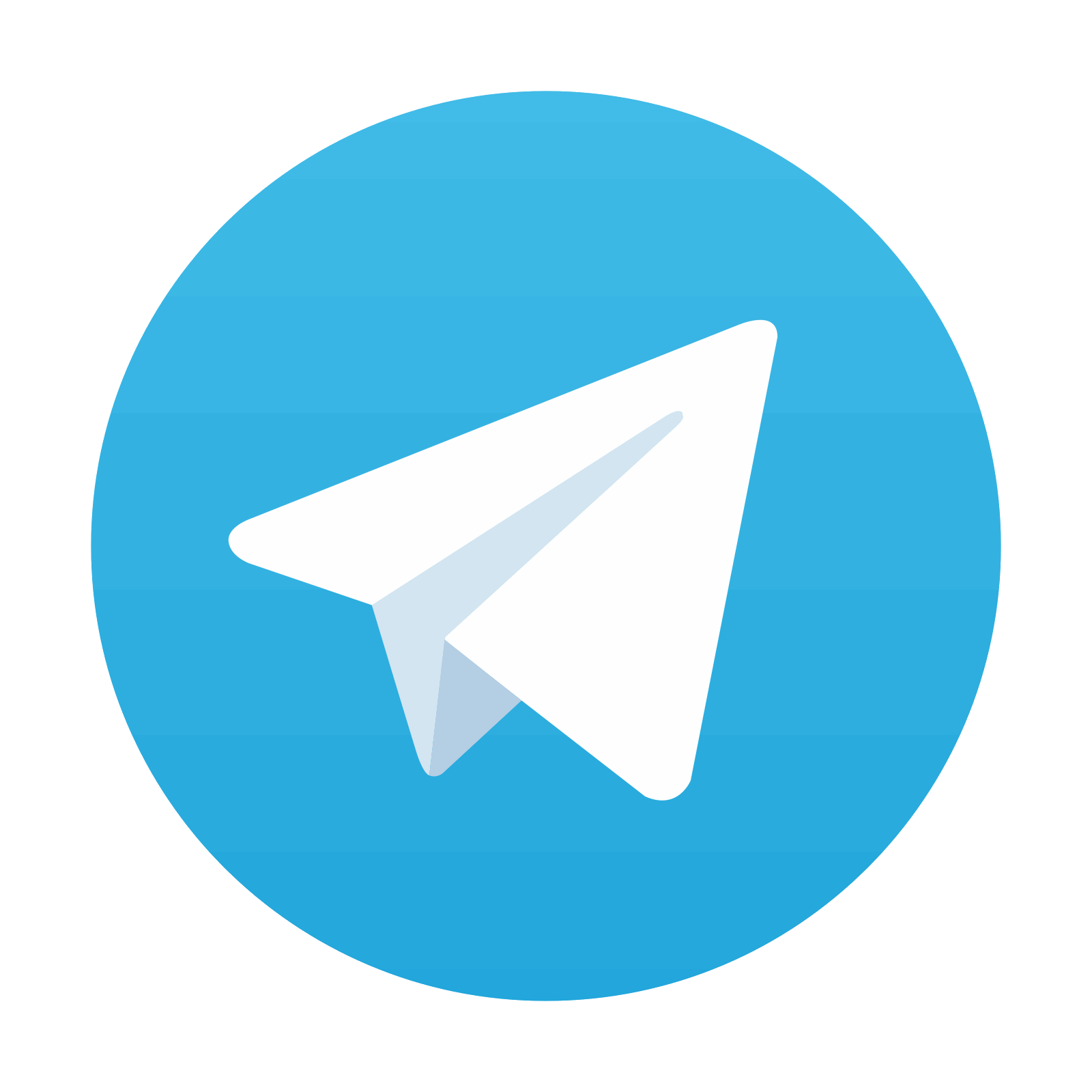
Stay updated, free articles. Join our Telegram channel

Full access? Get Clinical Tree
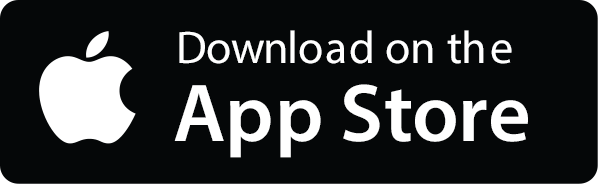
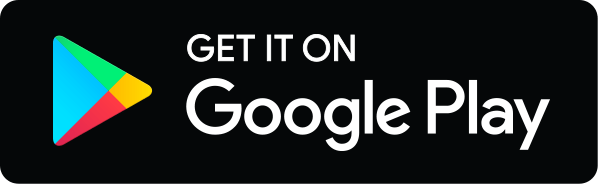
