Peak cardiac power output (CPO), as a direct measurement of overall cardiac function, has been shown to be a most powerful predictor of prognosis for patients with chronic heart failure. The present study assessed CPO and exercise performance in patients implanted with a left ventricular assist device (LVAD), those explanted due to myocardial recovery, and those with moderate to severe heart failure. Hemodynamic and respiratory gas exchange measurements were undertaken at rest and at peak graded exercise. These were performed in 54 patients—20 with moderate to severe heart failure, 18 with implanted LVADs, and 16 with explanted LVADs. At rest there was a nonsignificant difference in CPO among groups (p >0.05). Peak CPO was significantly higher in the explanted LVAD than in the heart failure and implanted LVAD groups (heart failure 1.90 ± 0.45 W, implanted LVAD 2.37 ± 0.55 W, explanted LVAD 3.39 ± 0.61 W, p <0.01) as was peak cardiac output (heart failure 9.1 ± 2.1 L/min, implanted LVAD 12.4 ± 2.2 L/min, explanted LVD 14.6 ± 2.9 L/min, p <0.01). Peak oxygen consumption was higher in the explanted LVAD than in the heart failure and implanted LVAD groups (heart failure 15.8 ± 4.1 ml/kg/min, implanted LVAD 19.8 ± 5.8 ml/kg/min, explanted LVAD 28.2 ± 5.0 ml/kg/min, p <0.05) as was anaerobic threshold (heart failure 11.2 ± 1.9 ml/kg/min, implanted LVAD 14.7 ± 4.9 ml/kg/min, explanted LVAD 21.4 ± 5.0 ml/kg/min, p <0.05). In conclusion, peak CPO differentiates well during cardiac restoration using LVADs and emphasizes the benefits of this therapy. CPO has the potential to be a key physiologic marker of heart failure severity and can guide management of patients with LVAD.
Cardiac power output (CPO) is a novel central hemodynamic measurement that demonstrates overall cardiac function and is the product of simultaneously measured cardiac output and mean arterial pressure. By incorporating pressure and flow domains of the cardiovascular system, CPO is an integrative measurement of cardiac hydraulic pumping ability. CPO has been shown to be a most powerful predictor of prognosis and mortality in patients with chronic heart failure and those with cardiogenic shock, whereas cardiac reserve, the difference between peak and CPO at rest, has been shown to be a major determinant of exercise capacity in heart failure. Interestingly, it seems that CPO has not been evaluated in patients supported with left ventricular assist devices (LVADs) and those with explanted LVADs due to myocardial recovery. Therefore, the first purpose of the present study was to assess and compare CPO at rest and peak exercise in patients implanted with LVADs, those explanted due to sufficient myocardial recovery, and those with moderate to severe heart failure. Second, the study evaluated and compared exercise performance among these 3 groups of patients.
Methods
The study population consisted of 54 men who attended cardiopulmonary exercise testing at Harefield Hospital (Harefield, United Kingdom). Data were collected from August 2006 to May 2008. Exclusion criteria included inability to perform treadmill exercise tests, inability to exercise beyond anaerobic threshold, symptomatic angina limiting exercise, and unwillingness to provide a consent form. Of the 54 patients recruited, 20 had moderate to severe heart failure, 18 have implanted LVADs, and 16 had explanted LVADs due to myocardial recovery. Patients’ clinical characteristics and medication are presented in Table 1 .
Variable | Patients With Heart Failure (n = 20) | Patients With Implanted LVAD (n = 18) | Patients With Explanted LVAD (n = 16) |
---|---|---|---|
Age (years) | 45 ± 10 | 39 ± 14 | 41 ± 14 |
Age range (years) | 22–64 | 19–58 | 18–63 |
Left ventricular ejection fraction (%) | 32 ± 14 | 50 ± 08 | 58 ± 14 |
Cause of heart failure | |||
Idiopathic dilated cardiomyopathy | 17 | 18 | 16 |
Coronary heart disease | 3 | — | — |
Medical therapy | |||
Angiotensin-converting enzyme inhibitors | 15 (75%) | 13 (72%) | 12 (75%) |
β blockers | 17 (85%) | 15 (83%) | 13 (81%) |
Diuretics | 18 (90%) | 16 (89%) | 12 (75%) |
Angiotensin II antagonists | 7 (35%) | 3 (17%) | 6 (38%) |
Aldosterone antagonists | 17 (85%) | 15 (83%) | 9 (56%) |
Digoxin | 15 (75%) | 11 (61%) | 13 (81%) |
Antiarrhythmics | 5 (25%) | 4 (22%) | 6 (38%) |
Clenbuterol | — | 4 (22%) | — |
Fifty-five percent of patients with heart failure had New York Heart Association (NYHA) class IV, and 45% had NYHA class III heart failure symptomatology. Pathogeneses of heart failure were ischemic heart disease in 15% and dilated cardiomyopathy in 85%. LV ejection fraction was 15% to 55%.
All patients with implanted LVADs received continuous blood flow LVADs. Fourteen patients had a HeartMate II LVAD (Thoratec Corp., Pleasanton, California) and 4 patients had a HeartWare LVAD (HeartWare Limited, Sydney, Australia). All patients with LVADs had dilated cardiomyopathy. LV ejection fraction (measured on pump) was 45% to 68%. Ten patients were in the first phase and 8 patients were in the second phase of the specific Harefield Recovery Protocol in which the β 2 -adrenergic receptor agonist clenbuterol also was administered. The first stage of the Harefield Recovery Protocol was intended to increase reverse remodeling, whereas the second stage aimed to induce “physiologic hypertrophy” using clenbuterol. Time since device implantation averaged 219 ± 87 days (range 62 to 388). The indication for insertion of the LVAD was development of severe heart failure that was not responsive to intensive medical treatment, including inotropic support, with evidence of impeding or actually multiorgan failure due to low cardiac output. At the time of data collection in all patients with LVADs, the device was set up at the optimal speed rate, from 9,000 to 9,600 revolutions/min for the HeartMate II LVAD and 2,600 to 3,500 revolutions/min for the HeartWare LVAD.
Most patients with explanted LVADs (87%) were in NYHA class I and 13% were in NYHA class II. All patients with explanted LVADs had dilated cardiomyopathy. LV ejection fraction was 50% to 72%. The mean period of LVAD support had been 396 days (range 22 to 638). Explantation was considered if the following criteria were met while the LVAD was off for 15 minutes: LV end-diastolic diameter <60 mm, LV end-systolic diameter <50 mm and LV ejection fraction >45%, LV end-diastolic pressure (or pulmonary-capillary wedge pressure) <12 mm Hg, cardiac index at rest >2.8 L/min per square meter of body surface area, and maximal oxygen consumption with exercise >16 ml/kg/min and an increase in minute ventilation compared to carbon dioxide production (ventilatory response) <34. Patients in the present study were tested at an average of 3.3 ± 1.1 years (range 0.3 to 5.8) after device explantation. All patients with explanted LVADs completed the 2 stages of pharmacologic regimen suggested by Birks et al. The study was approved by the ethics committee of the Royal Brompton and Harefield Foundation Trust (London, United Kingdom). All patients who participated in the study provided written informed consent.
This study took the form of a cross-sectional study in which cardiac and respiratory measurements were compared among the 3 groups of patients: (1) patients with moderate to severe heart failure, (2) patients with implanted LVADs, and (3) patients with explanted LVADs due to myocardial recovery.
Upon arrival in the transplantation exercise laboratory, patients’ weight and height were measured. Electrocardiographic electrodes were attached according to the standard lead configuration for exercise testing and electrocardiographic cables were connected. The patient then sat on a chair and, after a 5-minute resting period, arterial blood pressure was assessed from the brachial artery by cuff sphygmomanometry. This was followed by measurement of cardiac output in a seated position using the inert gas rebreathing method (Innocor A/S, Innovision, Odense, Denmark) as previously described. Briefly, the Innocor device employs a rebreathing system that uses an oxygen-enriched mixture of an inert soluble gas (0.5% nitrous oxide) and an inert insoluble gas (0.1% sulfur hexafluoride) from a 4-L prefilled anesthesia bag. Photoacoustic analyzers measure gas concentrations over a 5-breath interval. Nitrous oxide concentration decreases during the rebreathing maneuver at a rate proportional to pulmonary blood flow, allowing estimation of cardiac output. Three to 4 respiratory cycles are needed to obtain a value for nitrous oxide washout.
After cardiac output measurement at rest, a patient was connected to the Oxycon Pro (Jaeger, Wurzburg, Germany) metabolic cart with a disposable pneumotach attached to the face mask. Oxygen consumption, carbon dioxide production, and minute ventilation were measured. After 3 minutes of metabolic measurements at rest, patients with mild to severe heart failure and those with implanted LVADs performed a modified Bruce protocol, and those patients with explanted LVADs performed the Bruce protocol. Continuous breath-by-breath sampling of respiratory gases and heart rate measurements were undertaken; during the last 30 seconds of each exercise stage arterial blood pressure was measured and Borg scale recordings for dyspnea and fatigue were performed. Patients were instructed to give an approximately 1-minute warning before they felt they would end the exercise so that a final cardiac output rebreathing measurement was obtained at the peak of exercise; this is because the Innocor device takes approximately 45 seconds to boot up followed by 15 seconds to make the measurement. The patient continued to exercise through the measurement and cool down was started after it was complete. At the same time during the rebreathing maneuver, peak arterial blood pressure was measured.
Peak oxygen consumption was defined as average oxygen uptake during the last minute of exercise, expressed as milliliters per kilogram of body weight per minute. Oxygen consumption at the ventilatory (anaerobic) threshold was identified as the oxygen uptake before the systematic increase in the ventilatory equivalent for oxygen, without a concomitant increase in the ventilatory equivalent for carbon dioxide, together with the V-slope method. Echocardiographic measurement of LV ejection fraction was performed at rest in all studied patients on the same day that the cardiopulmonary exercise test was conducted.
The cardiac power output was calculated from the product of cardiac output and mean arterial pressure using the following equation: CPO = (QT × MAP) × K, where CPO is measured in watts, Q T is cardiac output in liters per minute, MAP is mean arterial pressure in millimeters of mercury, and K is the conversion factor (2.22 × 10 −3 ). The physiologic cardiac reserve is equal to the difference between peak CPO and baseline CPO at rest. Mean arterial pressure was calculated as SBP + 0.412 × (SBP − DBP), where SBP is systolic blood pressure and DBP is diastolic blood pressure. Ventilatory response to exercise was calculated by linear regression analysis using values of minute ventilation and carbon dioxide output as previously described. Age- and gender-adjusted maximal predicted oxygen consumption was determined using the treadmill equation previously described. Systematic vascular resistance to blood flow at peak exercise was estimated as the ratio between mean arterial pressure and cardiac output and, according to convention, was multiplied by a factor of 80 to convert units to dynes per second per centimeter to the fifth power.
All statistical analysis was carried out using SPSS 13.0 (SPSS, Inc., Chicago, Illinois). Before statistical analysis, data were checked for univariate and multivariate outliers using standard z-distribution cutoffs and Mahalanobis distance tests, respectively. Normality of distribution was assessed using a Kolmogorov-Smirnov test. To test differences in measured variables in patients with moderate to severe heart failure, those with implanted LVADs, and those with explanted LVADs, 1-way analysis of variance was used. To identify groups that differed significantly from 1 another, a post hoc Tukey test was performed. Statistical significance was indicated if the p value was <0.05. All data are presented as mean ± SD unless otherwise indicated.
Results
There were nonsignificant differences in age, body weight, and height in patients with moderate to severe heart failure, those with implanted LVADs, and those with explanted LVADs (p >0.05).
CPO at rest was not significantly different among groups with heart failure, implanted LVAD, and explanted LVAD (p >0.05). At peak exercise, CPO in patients with implanted LVADs and those with explanted LVADs was significantly higher compared to those with heart failure (p <0.01; Figure 1 ). Mean physiologic cardiac reserve of patients with explanted LVADs (2.43 ± 0.54 W) was significantly higher than in those with implanted LVADs (1.48 ± 0.55 W) and heart failure (1.07 ± 0.40 W, p <0.01). The mean difference in cardiac reserve of 0.36 W between the implanted LVAD and heart failure groups was not significantly different (p >0.05).
Cardiac output at rest was significantly greater (by 1.4 L/ min or 25%) in patients with implanted LVADs than in patients with heart failure (p <0.05) as were stroke volume and cardiac index, by 25% and 30%, respectively ( Table 2 ). At peak exercise, cardiac output was significantly greater in patients with implanted and explanted LVADs compared to patients with heart failure, as were heart rate, stroke volume, and cardiac index ( Table 2 ). Systematic vascular resistance at peak exercise was significantly higher (p <0.05) in patients with heart failure (845 ± 281 dyne/s/cm 5 ) than in patients with implanted LVADs (572 ± 155 dyne/s/cm 5 ) and those with explanted LVADs (556 ± 192 dyne/s/cm 5 ).
Variable | Cardiac Output (L/min) | Heart Rate (beats/min) | Stroke Volume (ml/beat) | Cardiac Index (L/min/m 2 ) | Systolic Blood Pressure (mm Hg) | Diastolic Blood Pressure (mm Hg) | Mean Arterial Pressure (mm Hg) |
---|---|---|---|---|---|---|---|
Sitting at rest | |||||||
Heart failure | 4.1 ± 1.3 | 74.6 ± 18.3 | 56.8 ± 20.8 | 1.95 ± 0.61 | 103.4 ± 13.8 | 68.9 ± 9.7 | 84.2 ± 10.8 |
Implanted left ventricular assist device | 5.5 ± 2.1 ⁎ | 72.6 ± 14.5 | 76.0 ± 21.2 ⁎ | 2.79 ± 0.86 † | 94.2 ± 20.1 | 59.9 ± 16.2 | 75.2 ± 16.6 |
Explanted left ventricular assist device | 5.2 ± 0.8 | 73.2 ± 6.6 | 70.8 ± 11.9 | 2.53 ± 0.46 ‡ | 104.6 ± 16.8 | 70.9 ± 13.6 | 86.2 ± 14.1 |
Peak exercise | |||||||
Heart failure | 9.1 ± 2.1 | 126.3 ± 27.3 | 71.1 ± 14.1 | 4.30 ± 0.99 | 123.2 ± 22.1 | 75.0 ± 12.6 | 95.9 ± 16.2 |
Implanted left ventricular assist device | 12.4 ± 2.2 ⁎ | 142.2 ± 27 ⁎ | 87.2 ± 16.4 † | 6.19 ± 1.33 ⁎ | 110.7 ± 24.1 | 67.7 ± 11.5 | 87.4 ± 14.5 |
Explanted left ventricular assist device | 14.6 ± 2.9 § | 163 ± 18.1 § | 89.4 ± 15.1 § | 6.95 ± 1.41 § | 137.8 ± 22.4 ¶ | 76.6 ± 12.7 | 102.8 ± 15.0 ∥ |
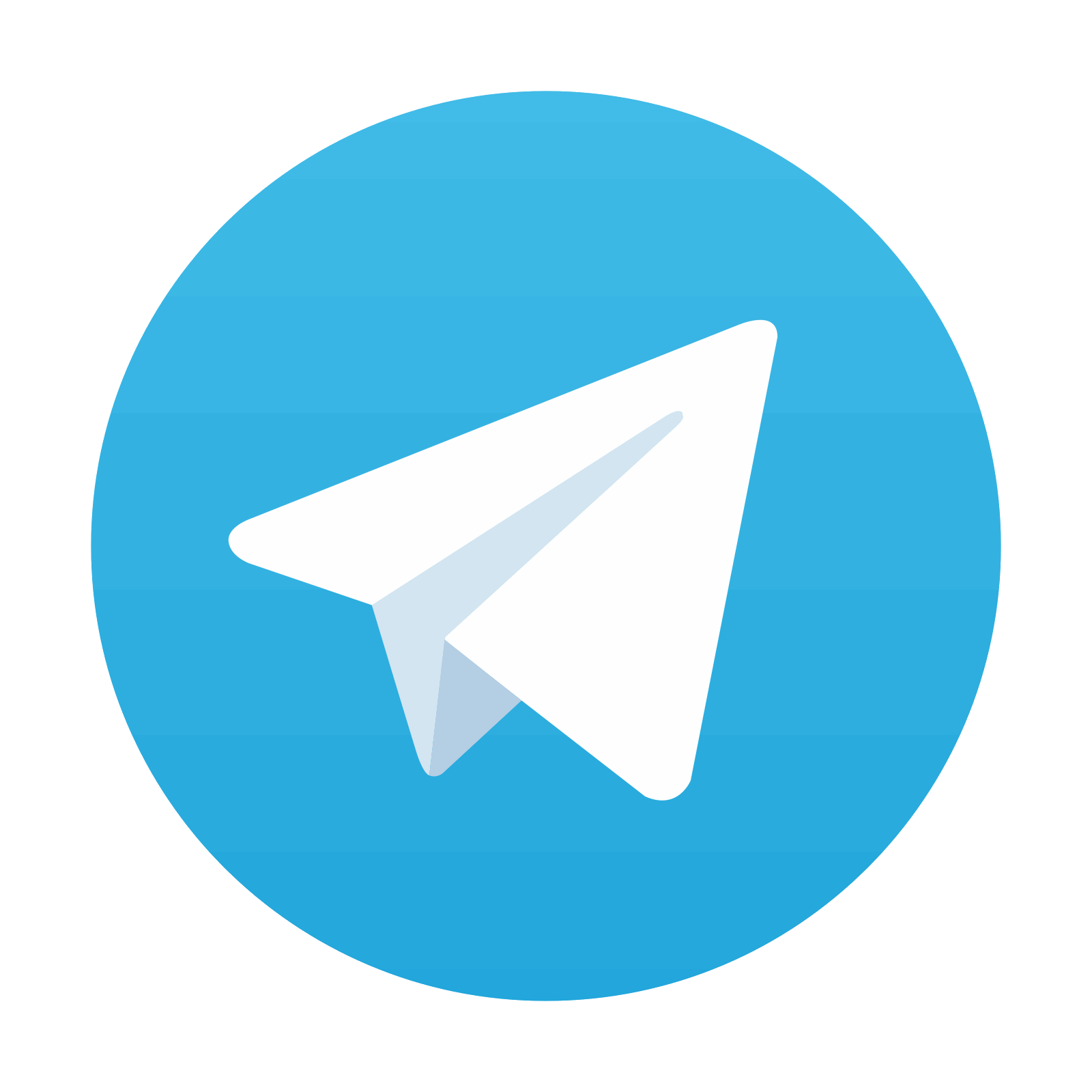
Stay updated, free articles. Join our Telegram channel

Full access? Get Clinical Tree
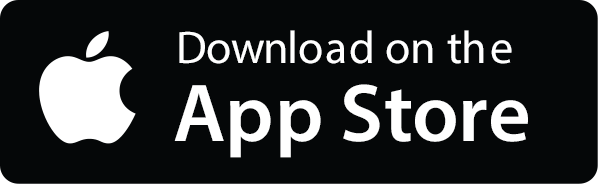
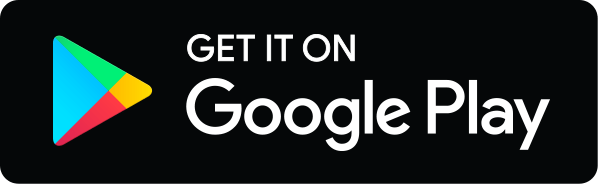
