Pro-hemorrhagic factors
Pro-thrombotic factors
Excessive heparin anticoagulation
Inadequate heparin anticoagulation
Consumption of coagulation factors
Acquired antithrombin deficiency
Low fibrinogen levels
Protein C-S complex consumption
Thrombocytopenia
Tissue factor pathway inhibitor consumption
Platelet dysfunction
Endothelial dysfunction
Hyperfibrinolysis
Heparin-induced thrombocytopenia
Acquired von Willebrand disease
Blood stagnation in the cardiac chambers
Surgical site bleeding
Endotoxins
Additionally to these factors, a major role is played by the nature of the ECMO support.
Basically, there is a great difference between venovenous ECMO, peripheral venoarterial ECMO, and postcardiotomy ECMO. Respiratory venovenous ECMO is usually applied through a peripheral cannulation of the femoral veins or jugular + femoral vein or single jugular vein cannulation with a double-stage cannula. Peripheral venoarterial ECMO is usually applied with groin vessels cannulation. Postcardiotomy ECMO is applied in cardiac surgery patients with difficult or impossible weaning from CPB. In this case, different kinds of cannulation may be used. Basically, a peripheral venoarterial ECMO can be placed, or, alternatively, a central cannulation of the right atrium and ascending aorta may be chosen. In this case, specific cannulas can be used, allowing closure of the chest.
However, even when the chest is closed, but particularly in case of open chest, postcardiotomy ECMO carries the greatest risk for severe bleeding. This is due to the fact that the coagulation system is already stressed by the long CPB run and by the great amount of TF and thrombin generated during the surgical procedure; finally, post-surgery hyperfibrinolysis, residual effects of large heparin doses, thrombocytopenia, and surgery itself are other determinants of bleeding. On the other side, intraoperative consumption of natural anticoagulants, like antithrombin (AT), tissue factor pathway inhibitor, and protein C-S complexes, determines a pro-thrombotic condition. As addressed in the following notes on anticoagulation, postcardiotomy ECMO requires a different approach to anticoagulation and heparin administration, at least during the first hours after implantation.
7.4 Hemostatic System Management During ECMO
7.4.1 Anticoagulation
Systemic anticoagulation during ECMO is intended to control thrombin generation and limit the risk for thrombotic and hemorrhagic complications. Unfractionated heparin (UFH) is the most commonly used anticoagulant [20]. UFH acts by binding and inactivating factor Xa and thrombin; however, heparin is not a direct thrombin inhibitor, and its efficacy is related to the presence of AT. UFH increases the kinetic of the natural thrombin-antithrombin binding by 2,000–4,000 times. Therefore, the efficacy of heparin as an anticoagulant is strongly dependent on the AT concentration: AT is a sort of “suicide” substrate, and once it is bound to thrombin and factor Xa, it needs to be reconstituted by the liver. As a consequence, chronic heparin administration consumes the endothelial and circulating pool of AT. Additionally, heparin may be bound and inactivated by plasma proteins, endothelial surface, and most of all by circulating platelets, which scavenge heparin by releasing PF4.
Due to this complex scenario, the exact dose required to correctly blunt thrombin generation is undefined and may greatly vary during the course of an ECMO. The classical dose range is reported between 20 and 70 IU/kg/h [20]. However, this dose may vary among individuals and within the same subject, depending on other conditions. Basically, chronic UFH infusion leads to AT consumption and consequently to a lower heparin sensitivity; once AT is corrected, the required dose of UFH decreases. Simultaneously, platelet consumption is inevitable during ECMO, and decreased levels of platelets lead to an increased sensitivity to UFH; again, once allogeneic platelet concentrates are administered and the platelet count recovers, the sensitivity to UFH decreases. Overall, this leads to the need for continuous adjustments of the UFH dose, even if in general there is a trend for larger doses the longer the ECMO system is in place [21].
Even given these limitations, heparin is still necessary while on ECMO, although some authors advocate the possibility of a heparin-free, no-anticoagulation-based ECMO [22–24]. This strategy may be considered in case of excessive bleeding risk, like for trauma patients.
To achieve peripheral vessels cannulation, a small (50–100 IU/kg) bolus dose of heparin is usually administered. In postcardiotomy ECMO, full heparinization is usually already achieved. In this case, a different strategy is suggested: after cannulation and onset of ECMO, heparin should be fully antagonized with protamine sulfate. Subsequently, given the residual effects of CPB and surgery, no heparinization is usually undertaken for the first 12–24 h, to avoid massive postoperative bleeding. Once bleeding is under control, heparin infusion should be started at a low dose (20 IU/kg/h) and subsequently adjusted to the desired level of anticoagulation.
7.4.2 Alternatives to Heparin
Theoretically, direct thrombin inhibitors could be used as an alternative to heparin. This is mandatory in case of heparin-induced thrombocytopenia (HIT).
Bivalirudin is a direct thrombin inhibitor with a short half-life of about 25 min and partial (20 %) kidney clearance [25, 26]. Its use during ECMO has been successfully reported in case of HIT [27–29]; recently, a relatively large series of patients without HIT and treated with bivalirudin as the sole anticoagulant for ECMO has been reported and compared with conventional UFH management [21], with a lower procedural bleeding and less need for allogeneic blood products transfusions.
The dose of bivalirudin is usually reported around 0.03–0.2 mg/kg/h, with [27–29] or without [21] an initial bolus of 0.5 mg/kg.
There are some caveats for the use of bivalirudin in ECMO. The first is that renal clearance may be strongly impaired in case of poor renal function, leading to drug accumulation; the second is that, given its nature, bivalirudin anticoagulation requires no blood stagnation in the circuit or inside the circulation. The ECMO circuit is closed and usually does not present stagnation areas; in venovenous ECMO, there is usually no blood stagnation inside the circulation. Conversely, in case of venoarterial ECMO for cardiac failure, some patients present large areas of blood stagnation inside the left heart chambers, easily detectable as a “smoke effect” at echocardiographic examination. In this case, the risk for cardiac thrombi formation is high, and bivalirudin should not be used [30].
Other direct thrombin inhibitors proposed for ECMO in case of HIT include argatroban [31] (0.1–0.4 μg/kg/min) while danaparoid and lepirudin have been used in the past but are presently abandoned.
7.4.3 Additional Drugs
Antiplatelet drugs have been proposed as additional agents during ECMO, in the attempt to preserve platelet function and prevent aggregation. Some authors propose the use of aspirin (1.5 mg/kg/day) for pumpless arteriovenous ECMO [32]. The use of dipyridamole, once quite popular [33], is presently rarely reported. Apart from anecdotical reports, no evidence exists with respect to the use of antiplatelet agents during ECMO.
Synthetic antifibrinolytics can be used when hyperfibrinolysis is suspected (excessive increase in FDP and d-dimers levels) [34].
The role of AT for the maintenance of a correct thrombin inhibition during UFH therapy has already been highlighted. Inevitably, AT is consumed during ECMO, and the majority of the authors suggest purified AT supplementation aimed to maintain AT activity at the lower normal range of 70 % [35, 36]. Of notice, when bivalirudin is used, AT consumption is strongly limited, albeit present [21].
7.5 Monitoring the Hemostatic System During ECMO
7.5.1 Activated Clotting Time (ACT)
ACT remains the standard of monitoring during heparin anticoagulation in ECMO. The ACT provides a bedside assessment of the intrinsic and common pathway integrity.
During ECMO, the ACT is usually maintained between 180 and 220 s [20]. However, it is well established that the correlation between heparin concentration and ACT is poor during CPB [37, 38]. However, direct measurement of heparin concentration is unpractical, and the optimal level of heparin concentration while on ECMO has not yet been established. Studies confronting heparin concentration with ACT values during ECMO reported variable heparin concentrations between 0.1 and 0.4 IU/mL, with correspondent ACT values ranging from 110 to 220 s [39–41].
7.5.2 Conventional Laboratory Tests
Activated partial thromboplastin time (APTT) explores the intrinsic and common pathways of coagulation and is the classical measure for heparin therapy [20]. APTT poorly correlates with ACT [42]; conversely, it has an acceptable degree of correlation with heparin concentration [43] and is therefore to be considered superior to the ACT for heparin treatment monitoring during ECMO. An APTT of 1.5 times the baseline APTT (50–80 s) is considered the target value during ECMO and corresponds to a heparin concentration of 0.2–0.3 IU/mL [20].
Prothrombin time (PT) is a marker of the extrinsic and common coagulation pathways and should be performed in order to detect the level of coagulation factors and to guide their supplementation with fresh frozen plasma (FFP), prothrombin complex concentrates (PCC), or cryoprecipitates.
Platelet count, fibrinogen levels, and d-dimers assays should be performed daily, since they determine the need for platelet concentrates, FFP, fibrinogen, and antifibrinolytics.
7.5.3 Thromboelastography and Thromboelastometry
Thromboelastography (TEG) and thromboelastometry (TEM) are dynamic tests based on the viscoelastic properties of blood during the coagulation process. In both tests, the time to change the physical nature of blood from liquid to gel (gel point) is represented by a straight line and defined as r time (TEG) or coagulation time (TEM). Once the gel point is reached, other parameters represent the kinetic of clot formation (alpha angle) and the retraction force of the clot (maximum amplitude in TEG and maximum clot firmness in TEM). Finally, the decrease over time of the clot strength is an index of fibrinolysis (clot lysis index in TEG and maximum lysis in TEM) (Fig. 7.1).
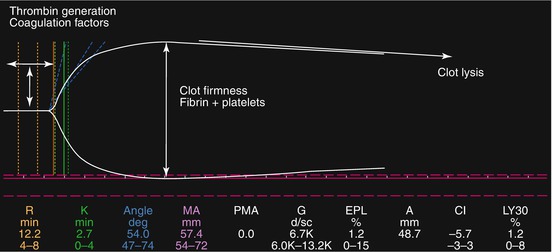
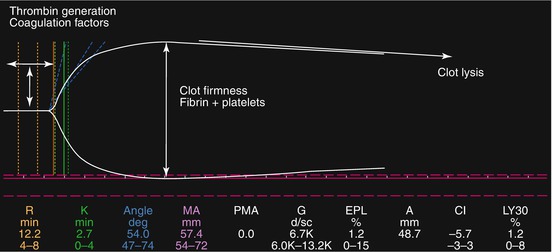
Fig. 7.1
Thromboelastographic tracing
TEG and TEM have a number of advantages over the routine coagulation tests. They provide a comprehensive and dynamic analysis of coagulation kinetic, can be done at point-of-care, provide data within about 30 min, and, finally, can be used for detecting hyperfibrinolysis. Therefore, their use for monitoring coagulation and anticoagulation gained wide popularity in recent years.
The r time and coagulation time are surrogates for thrombin generation and may guide the UFH infusion rate during ECMO. There is not a universally accepted value of r time for optimal UFH dose, but the majority of the authors report an optimal window between two and three times the upper normal limit (16–25 min) [20, 21] (Fig. 7.2).
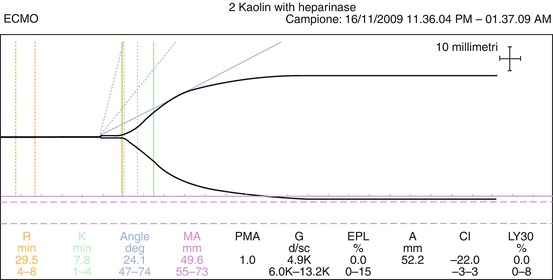
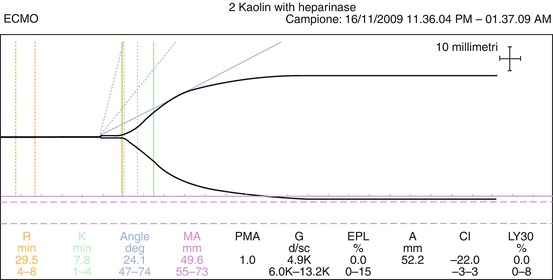
Fig. 7.2
An adequate TEG during ECMO
Both TEG and TEM offer an additional number of tests. Adding heparinase, it is possible to detect the “natural” underlying behavior of clot formation. This is particularly useful when the r time is excessively prolonged, to distinguish a heparin overload from a coagulation factor deficiency and for guiding the therapy with PCC, FFP, or cryoprecipitates. Specific tests (functional fibrinogen in TEG and Fibtem in TEM) provide a measurement of fibrinogen concentration (Fig. 7.3). These last values may guide the therapy with FFP and fibrinogen.
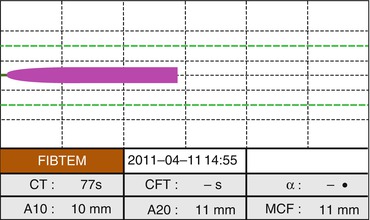
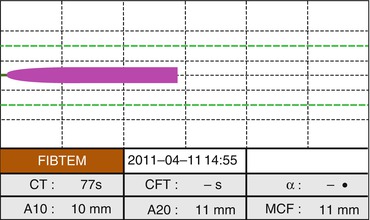
Fig. 7.3
ROTEM analysis for fibrinogen concentration (FIBTEM)
7.5.4 Other Hemostasis and Coagulation Tests
Given the limitations of ACT, and the fact that APTT values may change depending on the laboratory method used, some authors [44] suggested the use of more specific tests for the definition of the UFH infusion rate. The anti-Xa UFH assay measures the anti-Xa activity of heparin in plasma. An optimal value, corresponding to an APTT 1.5–2 times the baseline, is between 0.3 and 0.7 IU/mL [43].
Platelet function analysis with point-of-care tests during ECMO is suggested by some authors [35]. At present, there is a gap in knowledge about platelet function and antiplatelet drugs use during ECMO. There is not a clearly defined cutoff value suggesting platelet concentrate transfusion, and many of the available tests may be biased by the usually low platelet count during ECMO.
7.6 Adjusting the Coagulation Profile
Given the above reported data, it is possible to define an “optimal” coagulation pattern during ECMO. The main key point of this pattern is shown in Table 7.2.
Table 7.2
The optimal hemostatic pattern for the ECMO patient
Parameter | Suggested value |
---|---|
Activated clotting time (seconds) | 180–220 |
International normalized ratio | 1.3–1.5 |
R time at thromboelastography (seconds) | 16–25 |
Fibrinogen (mg/dL) | >100 |
Maximum clot firmness at FibTEM (mm) | >10 |
Antithrombin activity (%) | 70–80 |
Platelet count (cells/mmc) | >80,000 (bleeding patients/high risk) |
>45,000 (no bleeding/low risk) | |
d-dimers (μg/L) | <300 |
Guiding the patient into the framework of this optimal pattern is one of the most tricky steps during an ECMO management. UFH or bivalirudin dose should be adjusted based on ACT, APTT, and TEG/TEM. The other issues can be adjusted using allogeneic blood products or substitutes.
Purified AT is available for AT supplementation. AT may be administered using FFP, but very large doses are required.
A severe gap in plasma coagulation factors (INR >3) can be corrected with PCC or cryoprecipitates, whereas minor gaps (INR 2–3) could even be treated with FFP.
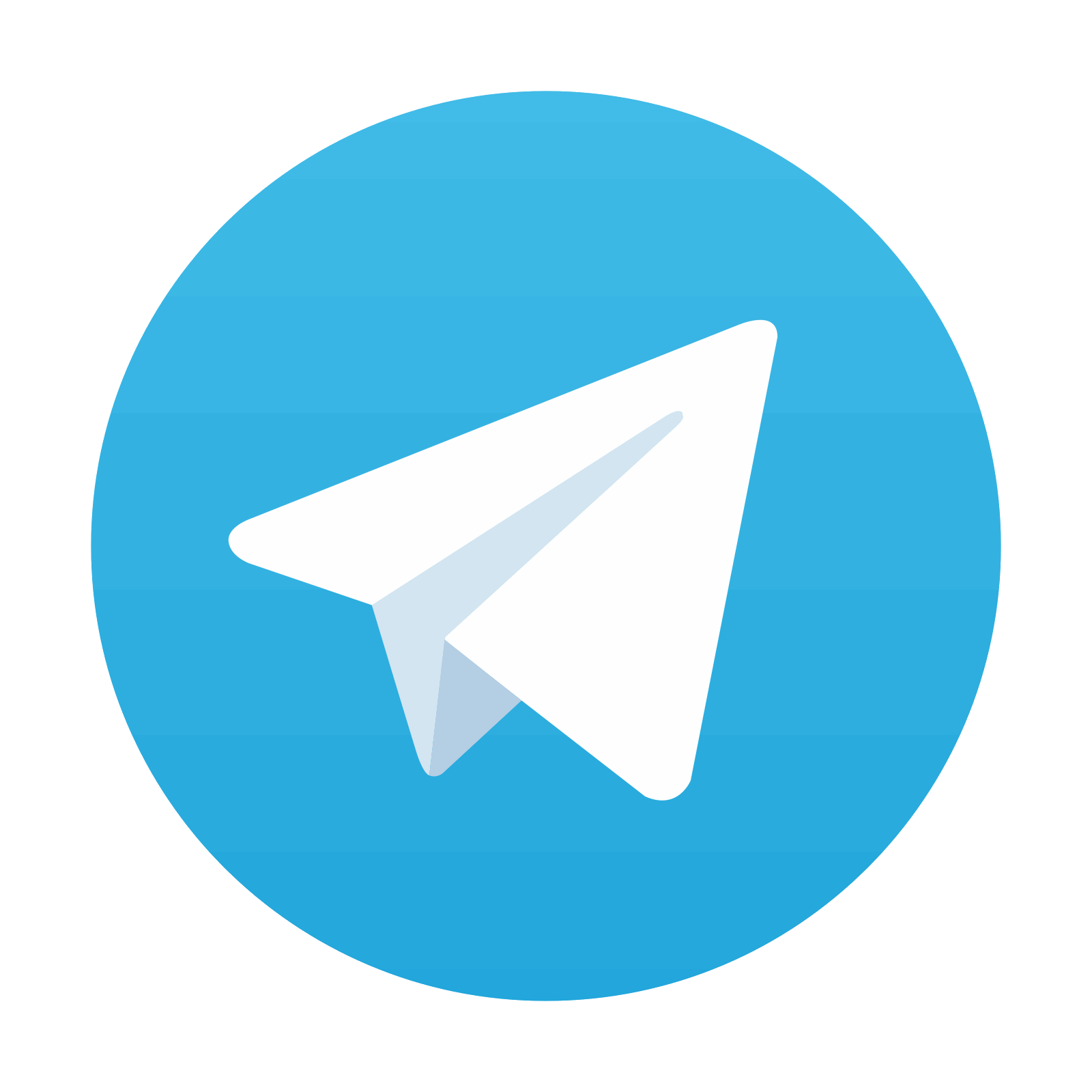
Stay updated, free articles. Join our Telegram channel

Full access? Get Clinical Tree
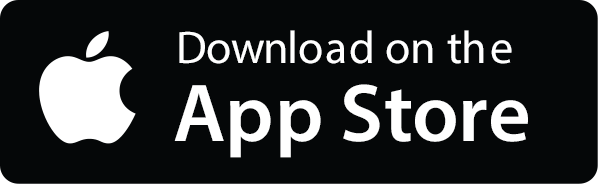
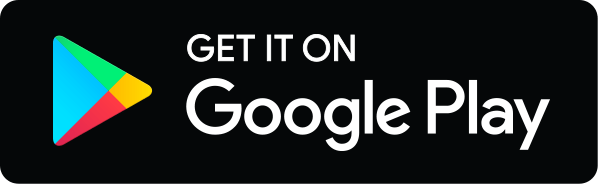