Fig. 2.1
The initiation and progression of atherosclerosis involves a complex set of inflammatory cytokines, which are derived from different cellular components such as macrophages, smooth muscle cells, platelets, endothelial cells, dendritic cells, T lymphocytes, and mast cells
Current risk prediction models for coronary heart disease (CHD) based on traditional risk factors are used to predict the long-term risk of adverse cardiovascular events in many individuals [5, 6]. However, these models have many shortcomings. Therefore, extensive research has been conducted to identify novel risk factors that can improve our ability to accurately predict cardiovascular risk, identify new targets for therapy, and improve current prognostic algorithms [7, 8]. Myocardial ischemia, necrosis, and subsequent remodeling are accompanied by the release of specific markers into the serum (Fig. 2.2) [9]. Currently, specific serum biomarkers of cardiovascular risk are being sought. As a general rule, new biomarkers for risk prediction need to be biologically plausible and measurable [1]. In addition, measurements for these biomarkers should be reproducible and should show a strong and graded relationship to the disease [1]. In the clinical setting, the laboratory tests for these biomarkers should be accurate and precise and should show low or modest variability [10]. Ideally, such biomarkers should predict the short-, intermediate-, and long-term risk of cardiovascular events, aid in monitoring therapy, help assess the patient’s prognosis after interventions, and improve risk stratification for the purpose of clinical decision making [1, 2]. Eventually, some of these biomarkers could become specific targets for therapy [1, 2].
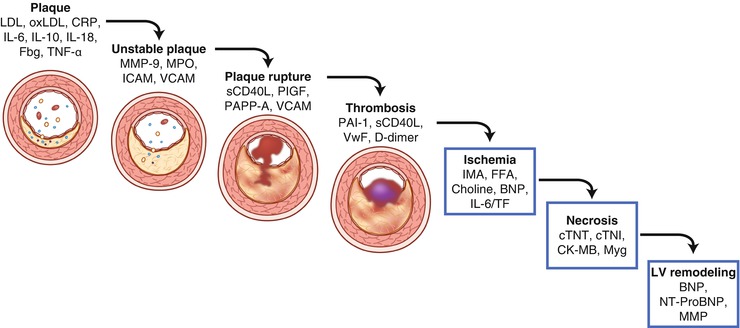
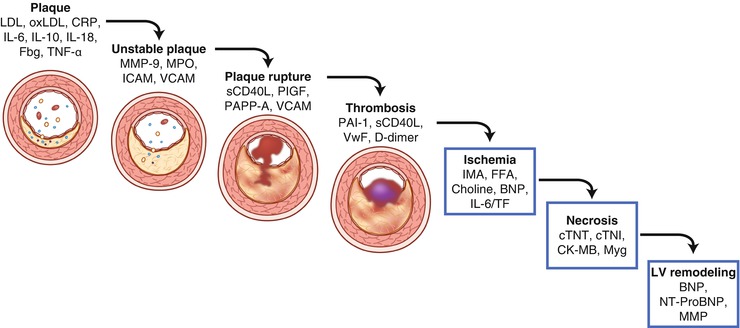
Fig. 2.2
Serum biomarkers increased in acute coronary syndrome. Diagram depicts the steps in disease progression for acute coronary syndrome and the associated increases in serum biomarker levels. BNP B-type natriuretic peptide, CK-MB creatine kinase MB, CRP c-reactive protein, cTNI cardiac troponin I, cTNT cardiac troponin T, Fbg fibrinogen, FFA free fatty acid, ICAM intercellular adhesion molecule, IL interleukin, IMA ischemia modified albumin, LDL low-density lipoprotein, MMP matrix metalloproteinase, MPO myeloperoxidase, Myg myoglobin, NT-proBNP N-terminal proBNP, oxLDL oxidized low-density lipoprotein, PAI-1 plasminogen activator inhibitor, PAPP-A pregnancy-associated plasma protein-A, PlGF placental growth factor, sCD40L soluble CD40 ligand, TF tissue factor, TNF tumor necrosis factor, VCAM vascular cell adhesion molecule, and VWF von Willebrand factor (Adapted from the following: Vasan [9])
As our understanding of the molecular basis of atherosclerosis has advanced, we have begun to identify many biomarkers that could potentially be used to assess CHD risk in clinical practice. In this chapter, we will discuss the use of serum biomarkers of inflammation, wall stress, and myocardial necrosis for predicting CHD risk. Furthermore, we will examine approaches and concepts related to the use of such biomarkers in clinical practice. These concepts can also be applied to the use of future biomarkers once further evidence establishes their use in clinical practice.
Biomarkers of Inflammation
C-reactive Protein
C-reactive protein is an acute-phase reactant. In the 1980s, de Beer, Maseri, and colleagues [11] found that C-reactive protein (CRP) levels are increased in patients admitted for acute myocardial infarction (MI). Subsequently, large epidemiological studies led by Ridker and colleagues have established a role for CRP in the prediction of CHD events [12–15]. However, increases in CRP levels are non-specific and may occur after many types of injury, inflammation, or infection (sometimes several fold). C-reactive protein activates the classical complement pathway and has pro-atherogenic and pro-inflammatory properties [16]. In addition, CRP activates endothelial cells, causing them to express the chemokine monocyte chemotactic protein-1 and adhesion molecules, such as intercellular adhesion molecule-1, vascular cell adhesion molecule-1, and selectins [16]. Furthermore, CRP decreases the expression and bioavailability of endothelial nitric oxide synthase in human endothelial cells [16, 17]. Although CRP is primarily produced by the liver in response to a rise in the levels of interleukin–6 (IL-6) and tissue necrosis factor-alpha (TNF-α) [1, 18], Calabro and colleagues [18] have shown that CRP is also produced by the smooth muscle cells and adipocytes of arterial blood vessels when exposed to interleukins and TNF-α and that it contributes to vascular inflammation.
As mentioned above, CRP is a non-specific marker of inflammation. In the absence of inflammation, CRP levels are usually less than 1 μg/mL, but in the presence of infections, autoimmune diseases, or malignancy, CRP levels can increase above 100 μg/mL [1, 19]. When assessing the risk of CHD, the predictive power of CRP resides primarily in the range between 1 and 5 μg/mL. High-sensitivity CRP (hs-CRP) levels of less than 1 μg/mL indicate a low risk for CHD, whereas levels above 3 μg/mL are associated with an increased risk for CHD [1]. Serum CRP levels higher than 10 μg/mL suggest the presence of an infection or other systemic inflammatory processes. When CRP levels are this high, the measurement must be repeated weeks to months later. The assay for CRP is well-standardized and widely available at this time. In addition, CRP is stable during long-term storage and has a long half-life. Moreover, CRP levels lack diurnal variations and are not dependent on age or sex. Because of these benefits and the strong predictive power of CRP, CRP measurements have become a part of routine clinical practice in many settings [2, 20].
Multiple prospective cohort studies conducted in different populations have shown that hs-CRP levels are a reliable marker of systemic inflammation and a strong predictor of future MI and stroke [2]. In addition, hs-CRP levels can predict both short-term (a few years) and long-term (up to 20 years) risk and are of value in primary and secondary prevention in men and women, in the young and the elderly, and in different ethnic groups [1, 12, 15, 21]. Furthermore, the predictive ability of CRP has been consistent across different populations with diverse clinical characteristics. C-reactive protein levels can also be used to predict the risk of a variety of cardiovascular outcomes, including the incidence of acute MI, stroke, and sudden cardiac death and the onset of peripheral artery disease, diabetes, and hypertension [7, 14, 15, 22]. Also, hs-CRP levels can predict recurrent MI in patients with acute coronary syndrome (ACS) [23, 24] and the prognosis and recurrence of events in patients with stroke [25] and peripheral arterial disease [26]. Moreover, they can be used to estimate the risk of restenosis after percutaneous coronary intervention [27].
A well-designed meta-analysis of 54 long-term, prospective studies that included individual records of 160,309 people who had no known history of vascular disease showed that serum CRP concentration has consistently been associated with the risk of CHD, ischemic stroke, and vascular mortality (Fig. 2.3) [28]. After adjusting for all conventional risk factors, the investigators found that each increase of 1 standard deviation in log(e) CRP concentration (i.e., about a threefold increase in concentration) was associated with a risk ratio of 1.37 for CHD [28]. As seen with previous studies, the predictive power of hs-CRP levels was partially accounted for by conventional risk factors and obesity and decreased after multivariate adjustments.
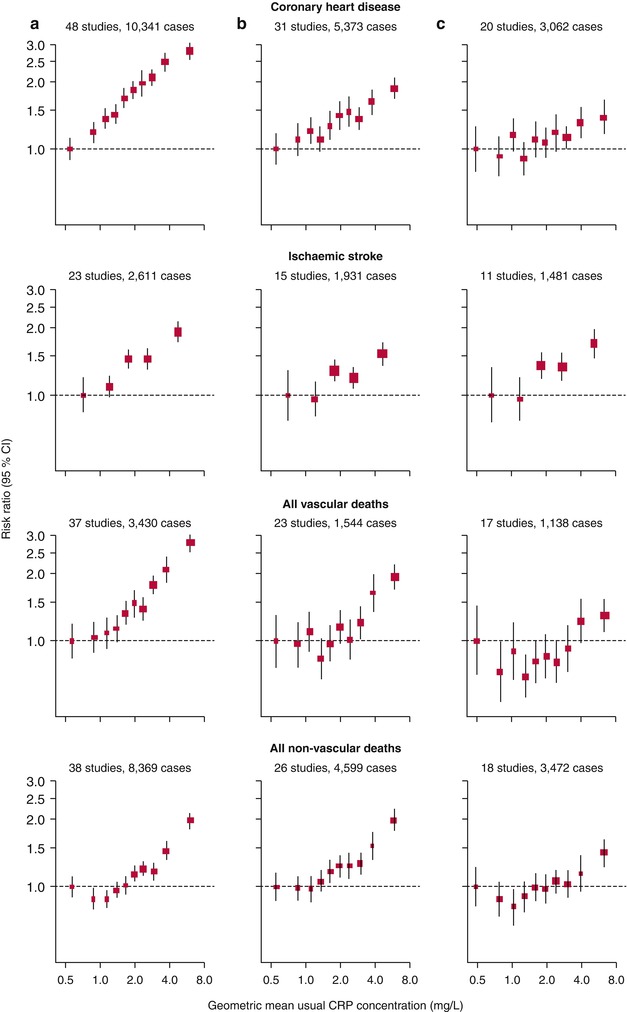
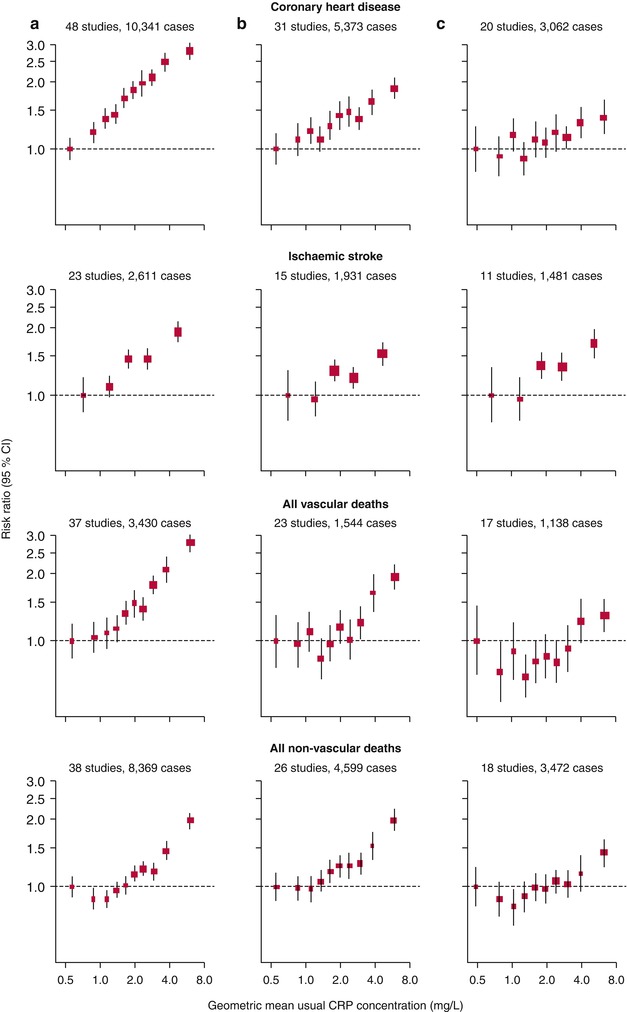
Fig. 2.3
Risk ratios for major vascular and non-vascular outcomes by quantiles of C-reactive protein (CRP) concentration, with different degree of adjustment for potential confounders. Adjusted study-specific loge risk ratios were combined by use of multivariate random-effects meta-analysis. The adjustments were age, sex, and study only (a); age, sex, study, systolic blood pressure, smoking, history of diabetes, body-mass index, concentrations of loge triglycerides, non-HDL cholesterol, and HDL cholesterol, and alcohol consumption (b); and (a) plus (b) plus fibrinogen (c). Studies with fewer than ten cases of any outcome were excluded from the analysis of that outcome. Error bars represent the 95 % CIs, calculated using floating absolute risk technique. The sizes of the boxes are proportional to the inverse of the variance of the risk ratios (From Kaptoge et al. [28]. Copyright © 2010 Elsevier Ltd. All rights reserved. Open Access: http://dx.doi.org/10.1016/S0140-6736(09)61717-7)
Although CRP has been proven to have multiple pro-inflammatory and pro-atherogenic properties in experimental settings [19], findings from a recent study using a Mendelian randomization approach have questioned whether CRP actually has a causal role in atherogenesis [29]. Nevertheless, because increased levels of CRP have consistently been shown to be associated with an increased CHD risk, CRP remains an established “marker of risk,” and it may indeed contribute to CHD. Multiple activities that are known to reduce CHD risk (i.e., smoking cessation, weight loss, and exercise) can also decrease serum CRP levels. In addition, CRP levels can be reduced by several medications, especially statins, aspirin, and clopidogrel [30–33].
Lipoprotein-associated Phospholipase A2
Lipoprotein-associated phospholipase A2 (Lp-PLA2), also known as platelet-activating factor acetylhydrolase (PAF-AH), belongs to a family of A2 phospholipases [34]. This enzyme hydrolyzes oxidized phospholipids, resulting in the production of lysophosphatidylcholine (lysoPC) and oxidized nonesterified fatty acids (NEFA) (Fig. 2.4) [35]. Plasma lysoPC has strong pro-inflammatory and pro-atherogenic effects. In contrast, hydrolysis of platelet-activating factor and other phospholipids by Lp-PLA2 has an anti-inflammatory effect. Therefore, Lp-PLA2 can have both pro-inflammatory and anti-inflammatory effects, although the balance tips toward the pro-inflammatory effects. Lipoprotein-associated phospholipase A2 is produced by hematopoietic cells (primarily by macrophages). Most Lp-PLA2 (80–85 %) is co-transported with circulating low-density lipoprotein (LDL), but it can be transported with high-density lipoproteins (HDL) and lipoprotein (a) in lesser amounts [34]. The enzyme activity of Lp-PLA2 is higher in small, dense LDL [36]. In human atherosclerotic plaques, Lp-PLA2 is produced locally by inflammatory cells (macrophages, T cells, and mast cells) and is possibly internalized from the circulation with LDL. Autopsy findings have shown that in coronary plaques, Lp-PLA2 immunostaining is particularly prominent in macrophage-rich areas within thin-cap fibroatheromas (Fig. 2.5) [37]. Lipoprotein-associated phospholipase A2 can be assayed based on its mass or enzymatic activity. Both Lp-PLA2 mass and Lp-PLA2 activity have been found to be correlated with age, male gender, smoking, and LDL cholesterol levels [38]. In addition, both Lp-PLA2 mass and Lp-PLA2 activity are significantly reduced by the use of statin drugs [39].
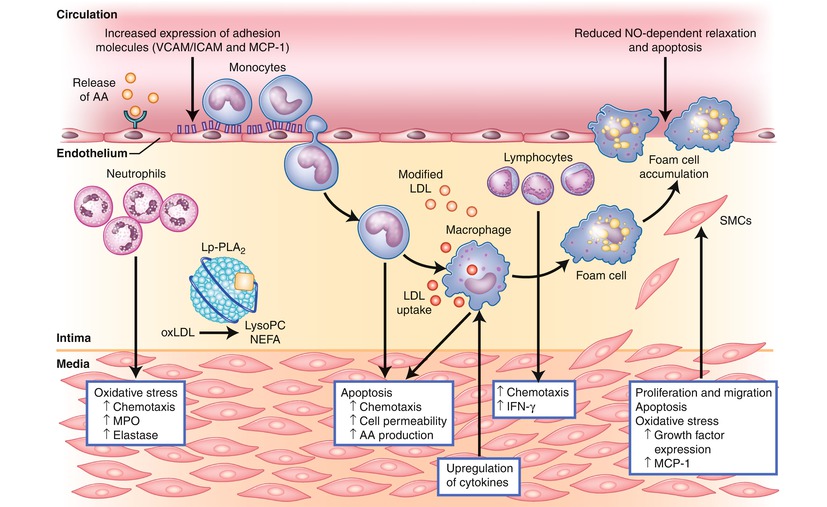
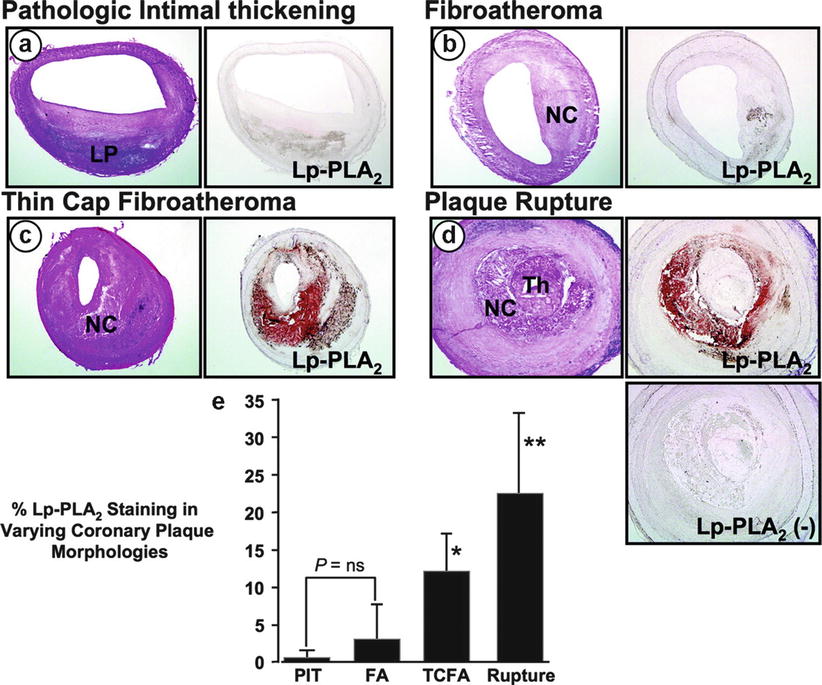
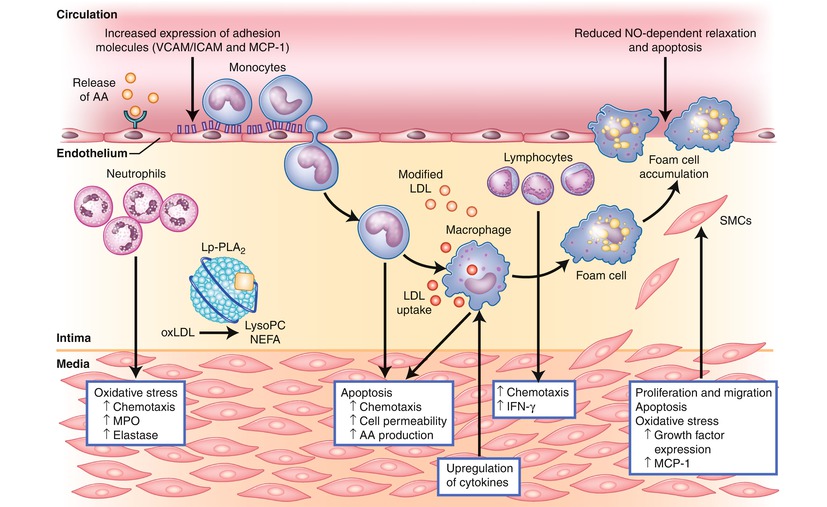
Fig. 2.4
Changes induced by the enzymatic products of lipoprotein-associated phospholipase A2 (Lp-PLA2). Lp-PLA2 hydrolyzes oxidized phospholipids to produce lysophosphatidylcholine (LysoPC) and nonestrified fatty acids (NEFA), which alter the function of multiple cell types, including endothelial cells, smooth muscle cells, monocytes, macrophages, and lymphocytes. AA arachidonic acid, ICAM intercellular adhesion molecule, IFN-γ interferon-gamma, LDL low-density lipoprotein, Lp-PLA 2 lipoprotein-associated phospholipase A2, LysoPC lysophosphatidylcholine, MCP-1 monocyte chemoattractant protein-1, MPO myeloperoxidase, NEFA nonestrified fatty acids, NO nitric oxide, oxLDL oxidized low-density lipoprotein, SMCs smooth muscle cells, VCAM vascular cell adhesion molecule (Adapted from the following: Ali and Madjid [34])
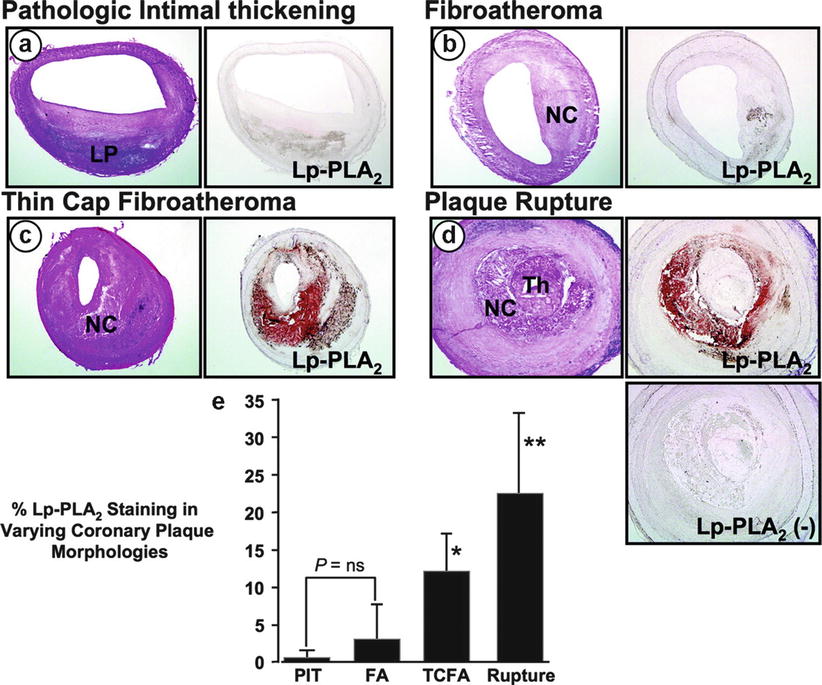
Fig. 2.5
Serial cryostat sections showing staining for lipoprotein-associated phospholipase A2 (Lp-PLA2) protein (right panels) in human coronary plaques with varying morphologies including (a) pathologic intimal thickening, (b) fibroatheroma, (c) thin-cap fibroatheroma, and (d) plaque rupture. The respective hematoxylin & eosin stained sections are also shown (left panels). The most intense Lp-PLA2 staining was found within the necrotic core and the adjoining macrophage-rich areas of the plaque, and Lp-PLA2 was highly expressed in the thin-cap fibroatheroma and the ruptured lesion. The panel labeled Lp-PLA2 (−) is a negative control for Lp-PLA2 staining, in which primary antibody was omitted. (e) Bar graph showing the % Lp-PLA2 staining for each plaque morphology; note that the highest intensity was observed in the ruptured plaque. *P < 0.05 vs FA or PIT; **P < 0.05 vs TCFA, FA, or PIT. FA fibroatheroma, LP lipid pool, NC necrotic core, ns not significant, PIT pathologic intimal thickening, TCFA thin-cap fibroatheroma, Th thrombus (From Kolodgie et al. [37]. Reprinted with permission from Wolters Kluwer Health)
In a case–cohort analysis of data from the West of Scotland Coronary Prevention Study (WOSCOPS), high baseline Lp-PLA2 levels were found to be strongly associated with an increased risk for CHD events, even after adjusting for traditional risk factors and hs-CRP levels [40]. Multiple large prospective cohort studies have since shown that a high Lp-PLA2 level (measured by mass or activity) is a strong, independent predictor of multiple cardiovascular outcomes [38, 40]. In most studies, the Lp-PLA2 attributable risk for occurrence of coronary events, stroke, or death remained statistically significant even after full adjustment for traditional risk factors [40–44].
A large and robust meta-analysis of individual data from 79,036 participants in 32 prospective studies showed that both Lp-PLA2 mass and activity predicted risk of CHD and vascular death (Fig. 2.6) [45]. After full adjustment for conventional risk factors, relative risk for CHD was 1.10 (95 % confidence interval [CI], 1.05–1.16) with 1 standard deviation (SD) higher Lp-PLA2 activity and 1.11 (1.07–1.16) with 1 SD higher Lp-PLA2 mass. Both Lp-PLA2 mass and activity predicted the risk of ischemic stroke, vascular mortality, and non-vascular mortality in patients with and without known vascular disease at baseline. In this study, after adjusting for other conventional risk factors, the magnitude of risk for CHD calculated for a 1 SD increase in Lp-PLA2 mass or activity was comparable to that for a 1 SD increase in systolic blood pressure or non-HDL cholesterol [45].
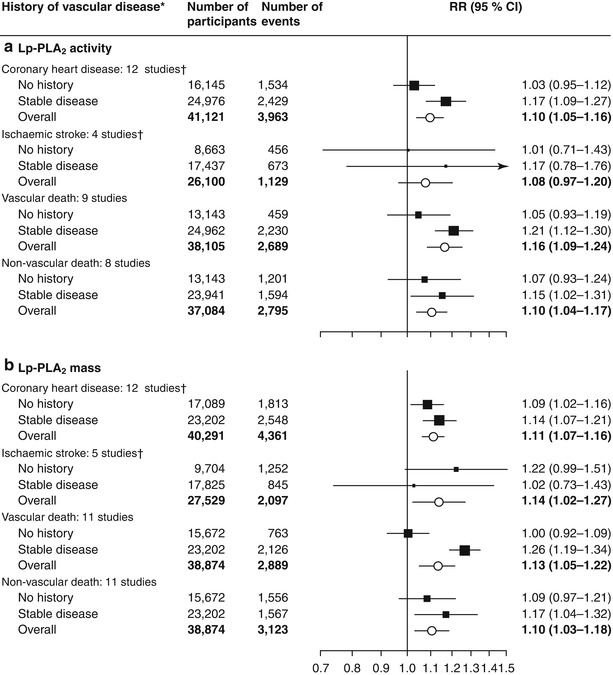
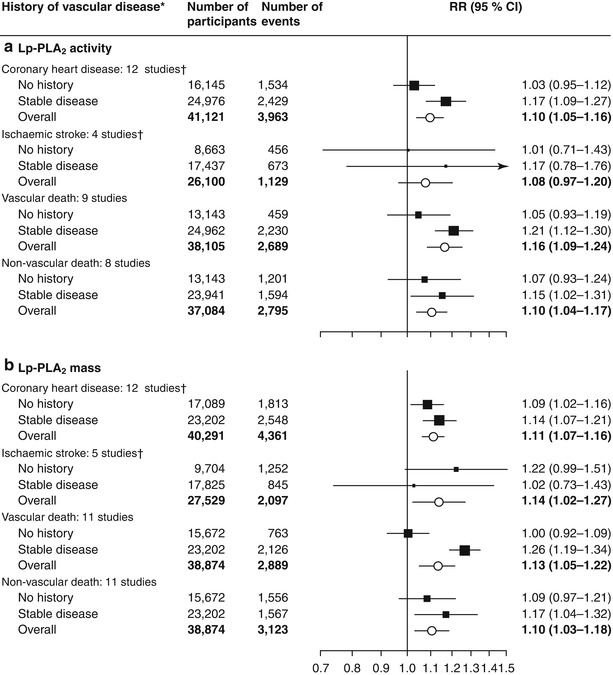
Fig. 2.6
Risk ratios for coronary heart disease, ischemic stroke, and vascular and non-vascular mortality per 1 SD higher Lp-PLA2 activity or mass at baseline, adjusted for several risk factors. Risk ratios were adjusted for multiple non-lipid and lipid risk factors. We noted no significant differences in risk ratios between people with and without a history of stable vascular disease at baseline, apart from for vascular death with Lp-PLA2 mass (P = 0.007). Error bars represent 95 % CIs. The sizes of the boxes are proportional to the inverse of the variance of the RRs. Lp-PLA 2 lipoprotein-associated phospholipase A2, RR risk ratio. *Diagnosis more than 30 days before baseline of myocardial infarction, angina, other coronary heart disease, stroke (including transient ischemic attack), peripheral vascular disease, or coronary surgery (including revascularizations). †Fatal and non-fatal events (From Thompson et al. [45]. Open Access Copyright © 2010 Elsevier Ltd. All rights reserved. http://dx.doi.org/10.1016/S0140-6736(10)60319-4)
The effect of Lp-PLA2 levels on CHD risk remains significant after adjustment for CRP levels. The Atherosclerosis Risk in Communities (ARIC) study and the Rancho Bernardo study also showed statistically significant, though modest, improvements in CHD risk discrimination of multivariate models when Lp-PLA2 was added, as determined by using area under the curve (AUC) analysis [46, 47]. According to the 2010 American College of Cardiology Foundation (ACCF)/American Heart Association (AHA) guideline for assessment of cardiovascular risk in asymptomatic adults, Lp-PLA2 measurement may be reasonable for cardiovascular risk assessment in intermediate-risk, asymptomatic adults (Level of Evidence: B, Class IIb) [7].
Biomarkers of Myocardial Stress/Stretch
B-type natriuretic peptide (BNP) is a hormone produced primarily in the cardiac ventricles in response to excessive wall stretch [48–50]. The functions of BNP include vasodilation, natriuresis, and inhibition of the renin-angiotensin and sympathetic nervous systems. The overall effect of BNP is a reduction in blood volume, which lowers blood pressure. The secretion of BNP can be induced by cytokines, such as interleukin-1β and tumor necrosis factor-α [51, 52], and by vasoconstrictors, such as angiotensin II [53]. BNP is derived from the enzymatic cleavage of prohormone proBNP, which also produces the biologically inactive N-terminal proBNP (NT-proBNP).
Both BNP and NT-proBNP have become established markers for use in the diagnosis of heart failure [54]. In addition, they have both been shown to provide prognostic information in patients with ACS [55–60] or stable CHD [61–64]. In these studies, increased levels were found to be associated with a higher risk of heart failure or death. Di Angelantonio et al. [65] performed a meta-analysis of 40 long-term, prospective studies comprising 87,474 participants. This study population included individuals from the general population (i.e., no pre-existing disease), individuals with elevated cardiovascular disease (CVD) risk factors, and individuals with stable CVD. When they compared individuals with baseline BNP levels in the top third of the study cohort to those in the bottom third, they found that those in the top third had a relative risk for CVD of 2.82 (95 % CI, 2.40–3.33). In 14 of these studies, receiver operating characteristic (ROC) curve analysis was used to assess the change in risk discrimination when either BNP or NT-proBNP was added to a risk prediction model of traditional factors. The improvements in risk discrimination ranged from 0.01 to 0.1 (Fig. 2.7). In the 2010 guidelines for the assessment of cardiovascular risk in asymptomatic adults (i.e., in apparently healthy adults), measurement of natriuretic peptides was not recommended for CHD risk assessment in this population because of a lack of definitive data [7]. Currently, there is no consensus as to the benefits of using BNP or NT-proBNP levels to guide treatment decisions [66, 67]; however, they have established utility in the diagnosis and management of patients with congestive heart failure [54].
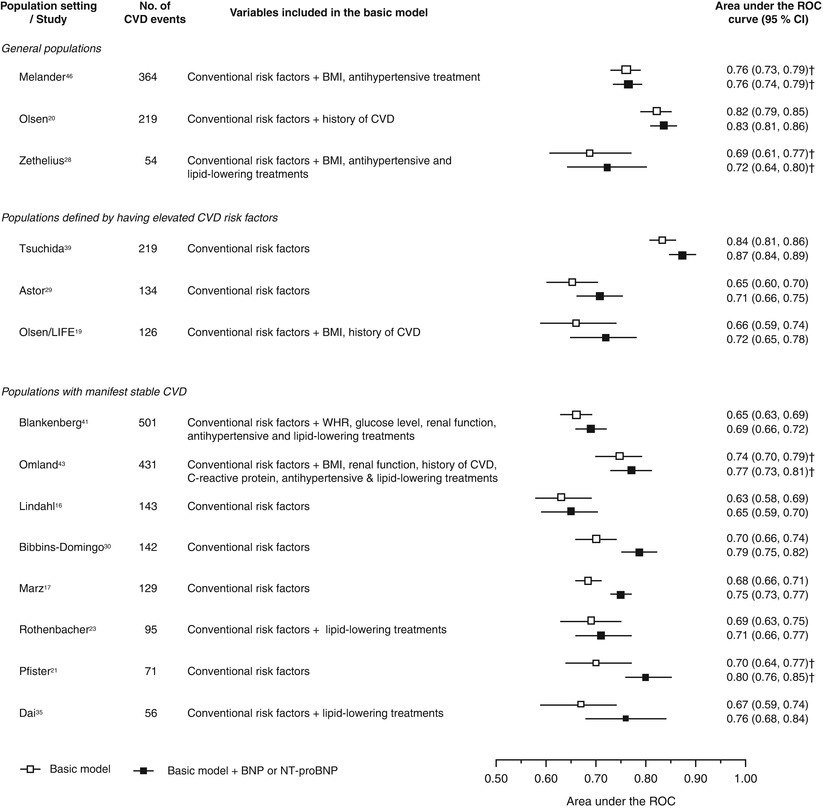
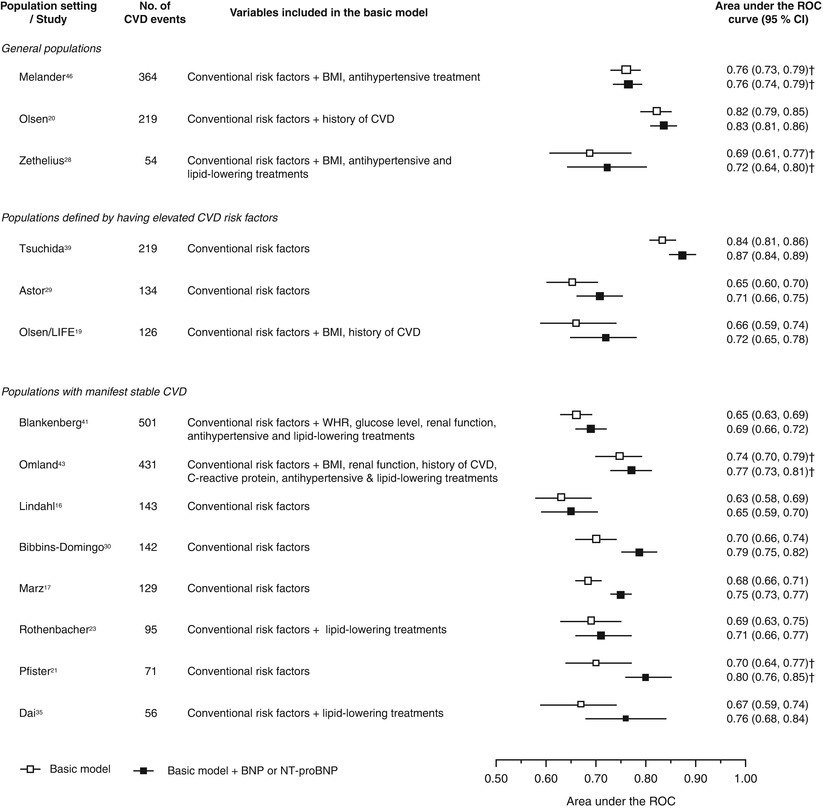
Fig. 2.7
Increment in risk prediction ability by adding BNP or NT-proBNP for cardiovascular disease. Conventional risk factors include age, sex (when appropriate), smoking, history of diabetes mellitus, blood pressure (and/or history of hypertension), total cholesterol (or non–high-density lipoprotein cholesterol), and high-density lipoprotein cholesterol. BMI indicates body mass index, WHR waist-to-hip ratio, and ROC receiver-operating characteristic curve. †C index (95 % CI) (From Di Angelantonio et al. [65]. Reprinted with permission from Wolters Kluwer Health)
Biomarkers of Necrosis
Cardiac troponin T (cTnT) and cardiac troponin I (cTnI) are part of a heterotrimeric protein complex that regulates Ca2+-mediated contractions in cardiac muscle by controlling the interactions between actin and myosin [68]. cTnI is found exclusively in the myocardium [69]. Although cTnT can also be found in small amounts in skeletal muscle, current cTnT assays are specific for the myocardial form [70]. These biomarkers are released into the circulation as a result of myocardial necrosis and are indicators of damage to the heart muscle. Both provide similar information and are the preferred biomarkers for the diagnosis of MI [71]. Because these 2 troponins indicate myocardial necrosis, they are not specific for MI and may also be increased in other conditions that lead to heart muscle damage, such as heart failure, renal failure, cardiac surgery, and several forms of cardiomyopathy [71, 72]. Therefore, according to recent guidelines, clinicians are advised to use serial cardiac troponin testing (i.e., at admission and 3–6 h later) to differentiate between MI and chronic myocardial disease [71]. Myocardial infarction should be suspected when the patient’s cardiac troponin levels show a change over time (rise and/or fall) and at least one of the measurements is elevated (i.e., a value exceeding the 99th percentile of a normal reference population). Furthermore, the patient should show at least one clinical indicator of ischemia.
Increased troponin levels have consistently been shown to be associated with a higher risk of adverse events in patients with stable CHD [73–76]. Recently, high-sensitivity assays for cTnT (hs-cTnT) and cTnI (hs-cTnI) have been developed; the limit of detection of these assays can be 10-fold lower than that of conventional assays [76]. In a study of patients with stable CHD, 3,630 of the 3,679 study participants had cTnT levels that would be undetectable by the conventional assay [76]. Nonetheless, increased hs-cTnT levels in these patients were associated with risk of heart failure, indicating the usefulness of these high-sensitivity assays when determining the prognosis of individuals with cTnT levels below the limit of detection in conventional assays. In a prospective study of 984 patients with stable CHD, increased hs-cTnT levels were associated with a greater risk of secondary cardiovascular events (including MI, heart failure, or cardiovascular death) [73]. In this study, patients in the highest tertile of hs-cTnT levels had 5 times as many cardiovascular events during follow-up (median, 8.2 years) as patients in the lowest tertile (hazard ratio [HR], 5.19; 95 % CI, 3.80–7.09; P < 0.001). Using a Cox proportional hazards regression model, the researchers also found that after adjusting for clinical risk factors, echocardiogram measurements, and other cardiovascular biomarkers (NT-proBNP and CRP), each doubling of hs-cTnT level was independently associated with a 37 % increase in the risk of cardiovascular events (HR, 1.37; 95 % CI, 1.14–1.65; P = 0.001) (Table 2.1).
Table 2.1
Association of hs-cTnT level (per doubling) with cardiovascular events after adjusting for clinical risk factors and measures of disease severity
Myocardial infarction | Heart failure | Cardiovascular death | Any event | |||||
---|---|---|---|---|---|---|---|---|
HR (95 % CI)a | P value | HR (95 % CI)a | P value | HR (95 % CI)a | P value | HR (95 % CI)a | P value | |
Unadjusted | 1.74 (1.50–2.03) | <.001 | 2.30 (2.04–2.59) | <.001 | 1.95 (1.70–2.23) | <.001 | 1.98 (1.80–2.17) | <.001 |
Model 1b | 1.55 (1.26–1.91) | <.001 | 1.92 (1.62–2.28) | <.001 | 1.78 (1.46–2.16) | <.001 | 1.68 (1.47–1.92) | <.001 |
Model 2c | 1.32 (1.02–1.71) | .03 | 1.55 (1.19–2.01) | .001 | 1.39 (1.06–1.83) | .02 | 1.42 (1.19–1.70) | <.001 |
Model 3d | 1.28 (0.99–1.67) | .06 | 1.44 (1.10–1.89) | .007 | 1.36 (1.03–1.81) | .03 | 1.37 (1.14–1.65) | .001 |
Studies have also assessed the use of cardiac troponin levels in patients with ACS for risk stratification and guiding clinical management. Reichlin et al. [77] have shown that both hs-cTnT and hs-cTnI levels may be useful for risk stratification in patients with unstable angina. In this study, only 26–31 % (depending on the specific test used) of patients with unstable angina showed a change in cardiac troponin levels ≥2 ng/L within the first hour after presentation, but the patients exhibiting this change showed a significantly higher risk of death or MI at 30-day and 2-year follow-up. These findings suggest that this group of patients may benefit from more aggressive treatment. Patients with ACS who have increased levels of cardiac troponins have been shown to benefit more from treatment with low molecular weight heparin [78, 79] or glycoprotein IIb/IIIa receptor inhibitors [80, 81] than do patients with low levels. Likewise, the reduction in risk of death or MI after undergoing an invasive strategy has been found to be greater in patients with increased levels of cardiac troponins than in patients with low levels [82, 83].
Use of Serum Biomarkers to Improve Cardiovascular Risk Prediction: The Case of CRP
C-reactive protein was the first biomarker used to improve CHD risk prediction models, and, therefore, it is currently the most well-studied. Most studies have shown that adding hs-CRP measurements to a CHD risk prediction model only modestly improves the predictive power [84]. However, after adjustments for conventional risk factors have been made, the significance of these improvements, as assessed by changes in the area under the ROC curve, has been questioned [21, 85]. In fact, the statistical and clinical utility of adding biomarkers to a CHD risk model differs based on a patient’s baseline CHD risk assessment [2]. Adding hs-CRP measurements may not substantially improve risk prediction models in low- or high-risk groups; however, this addition is helpful in reclassifying patients who fall into the intermediate-risk category [21, 86]. Based on this concept, Ridker and colleagues [6, 86] have developed and validated a new prediction model (the Reynolds Risk Score), which incorporates hs-CRP measurements to help reclassify intermediate-risk women and men into higher or lower risk groups. According to the latest scientific statement from ADDF/AHA, measurement of CRP may be reasonable for cardiovascular risk assessment in asymptomatic men 50 years of age or younger or women 60 years of age or younger who are at intermediate-risk (Level of Evidence: B) (Table 2.2) [7]. However, in low- or high-risk patients, CRP measurement might not be helpful [7].
Table 2.2
American College of Cardiology/American Heart Association recommendations for the measurement of C-reactive protein
Class IIa |
In men 50 years of age or older or women 60 years of age or older with LDL cholesterol less than 130 mg/dL; not on lipid-lowering, hormone replacement, or immunosuppressant therapy; without clinical CHD, diabetes, chronic kidney disease, severe inflammatory conditions, or contraindications to statins, measurement of CRP can be useful in the selection of patients for statin therapy. (Level of Evidence: B) |
Class IIb |
In asymptomatic intermediate-risk men 50 years of age or younger or women 60 years of age or younger, measurement of CRP may be reasonable for cardiovascular risk assessment. (Level of Evidence: B) |
Class III: No benefit |
In asymptomatic high-risk adults, measurement of CRP is not recommended for cardiovascular risk assessment. (Level of Evidence: B) |
In low-risk men younger than 50 years of age or women 60 years of age or younger, measurement of CRP is not recommended for cardiovascular risk assessment. (Level of Evidence: B) |
Use of Multiple Biomarkers to Improve Cardiovascular Risk Prediction
Atherogenesis involves a complex myriad of distinct pathways and molecular mechanisms that often overlap each other. Different biomarkers represent different pathways; therefore, an approach that uses several biomarkers representing different pathways might, in theory, further improve our predictive tools [47, 87]. Using a similar approach, both the Malmö Diet and Cancer study and the Cardiovascular Health study found that the addition of more novel biomarkers to risk models based on conventional risk factors resulted in small increases in the power to identify high-risk patients [88, 89].
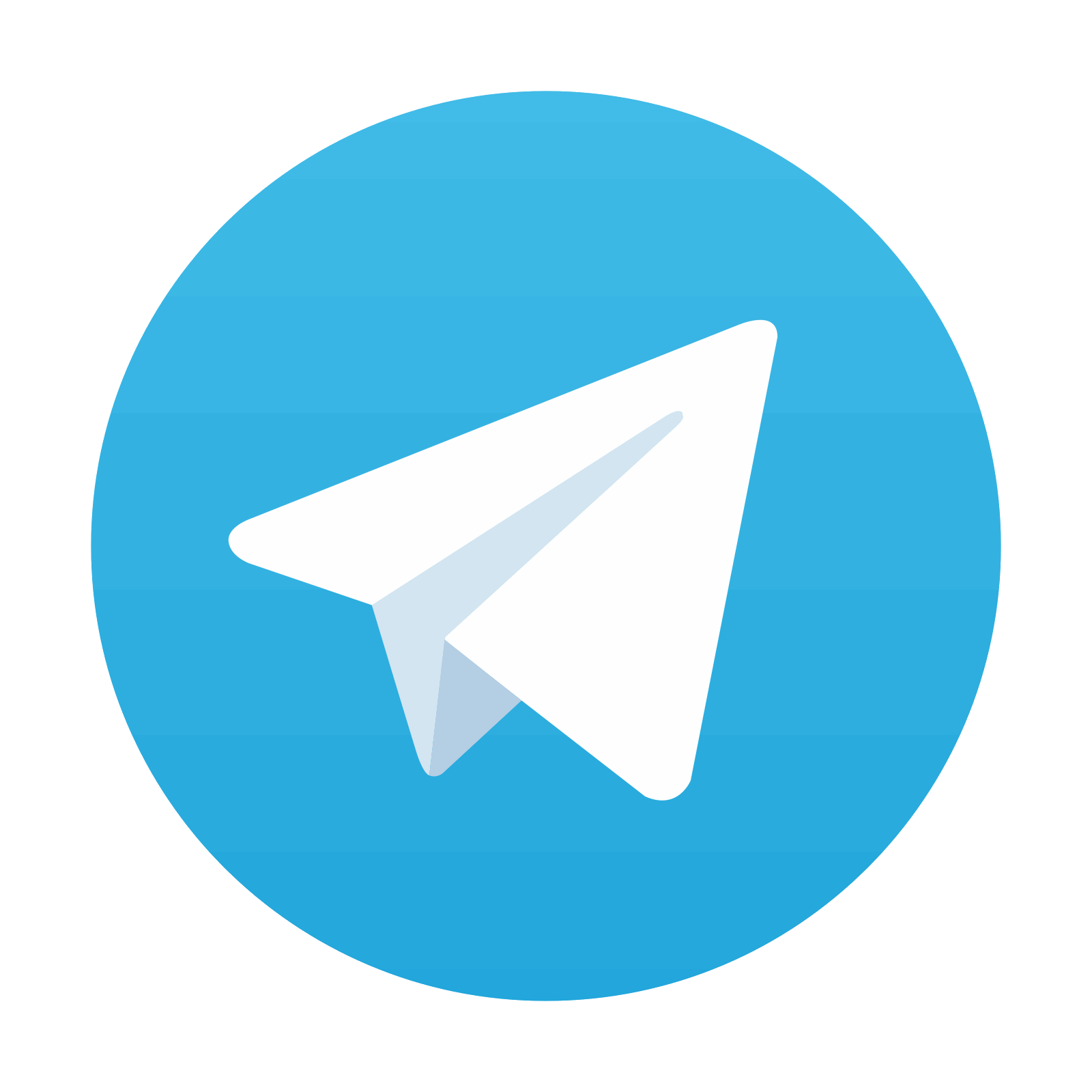
Stay updated, free articles. Join our Telegram channel

Full access? Get Clinical Tree
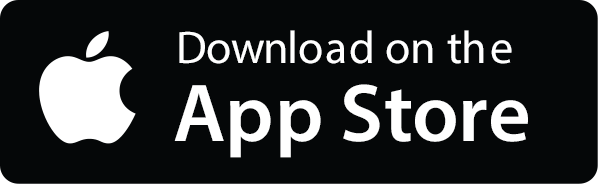
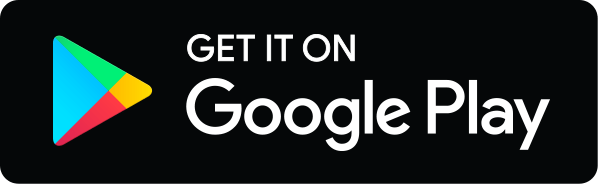