In patients with heart failure, exercise echocardiography can help in risk stratification and decision making. The prognostic significance of exercise pulmonary hypertension (PH) in patients with secondary mitral regurgitation (MR) remains unknown. The aim of the present study was to assess the prognostic value of exercise PH in patients with secondary MR and narrow QRS intervals. From 2005 to 2012, 159 consecutive patients with secondary MR, narrow QRS intervals, left ventricular dysfunction (mean ejection fraction 36 ± 7%), and measurable systolic pulmonary arterial pressure (SPAP) during exercise echocardiography were included. Resting and exercise PH were defined as SPAP >50 and >60 mm Hg, respectively. Exercise PH was more frequent than resting PH (40% vs 13%, p <0.0001). On multivariate logistic regression, the independent determinants of exercise PH were resting SPAP (p <0.0001), exercise MR severity (p <0.0001), and e′-wave velocity (p = 0.004). The incidence of cardiac events during follow-up was significantly higher in patients with exercise PH compared with those without exercise PH (4 years: 40 ± 7% vs 20 ± 5%, p <0.0001). Patients with exercise PH exhibited higher rates of cardiac events and death than those with resting PH. In a multivariate Cox proportional hazards model, exercise PH was independently associated with the occurrence of cardiac events (p <0.0001). In conclusion, in patients with secondary MR, exercise PH is determined mainly by resting SPAP, left ventricular diastolic burden, and exercise MR severity. Exercise PH is a powerful predictor of poor outcomes, with a 5.3-fold increased risk for cardiac-related death during follow-up. These results highlight the added value of exercise echocardiography in secondary MR.
In asymptomatic valvular heart disease, exercise pulmonary hypertension (PH) was recently identified by our group as a powerful marker of advanced risk for cardiac events. In secondary mitral regurgitation (MR), exercise systolic pulmonary arterial pressure (SPAP) is determined mainly by dynamic MR and is involved in the pathogenesis of acute pulmonary edema. The most recent guidelines recommend mitral valve surgery in asymptomatic patients with preserved left ventricular (LV) ejection fractions and severe primary MR, in the presence of exercise PH (a European Society of Cardiology class IIb indication ). There is no overt indication for surgery in patients with secondary MR and exercise PH, but the European Society of Cardiology guidelines state that exercise PH might be an additional motivation to perform surgery in patients with moderate MR who undergo coronary artery bypass graft surgery (level of evidence C). Nevertheless, the prognostic significance of exercise PH in patients with secondary MR remains unknown. The aim of the present study was to assess the prognostic value of exercise PH in patients with secondary MR. We hypothesized that exercise PH is an independent predictor of the occurrence of acute pulmonary edema, cardiac events, and mortality.
Methods
From 2005 to 2012, any patients with secondary MR and chronic LV systolic dysfunction (LV ejection fraction <45%) referred to our heart valve clinic to undergo exercise stress echocardiography were screened for ischemia, exercise tolerance, and dynamic hemodynamic alterations (increase in MR severity, PH, increase in LV filling pressures) (n = 315). Patients fulfilling the following inclusion criteria were eligible to be included in this study: (1) chronic secondary MR, (2) sinus rhythm, (3) narrow QRS interval (<120 ms), and (4) measurable SPAP during exercise echocardiography. Of note, 19 patients (6%) were excluded from the final analysis according to this last exclusion criterion, resulting in a feasibility rate in the measurement of exercise SPAP of 94%. The final studied population was composed of 159 consecutive patients with heart failure (mean LV ejection fraction 36 ± 7%, range 20% to 44%). All had been stable for ≥2 months, and none met the following exclusion criteria (1) technically inadequate echocardiogram, (2) more than trivial aortic regurgitation or mild or greater aortic stenosis or severe tricuspid regurgitation, (3) history of myocardial infarction <6 months, (4) atrial fibrillation or flutter, (5) evidence of inducible ischemia, and (6) extensive myocardial ischemia requiring coronary angiography and revascularization. None of the patients were in New York Heart Association functional class IV. All patients gave their informed consent, and the local ethics committee approved the protocol.
The presence of hypercholesterolemia was defined as total plasma cholesterol level >6.2 mmol/L or the use of cholesterol-lowering medications. Systemic hypertension was defined as the use of antihypertensive medications or having known but untreated hypertension (blood pressure ≥140/90 mm Hg).
All echocardiographic parameters were obtained at rest and at peak exercise in the same cycling semisupine position (Vivid 7 or 9; GE Healthcare, Little Chalfont, United Kingdom). All Doppler echocardiographic recordings were stored on a dedicated workstation for subsequent off-line analysis. For each measurement, ≥2 cardiac cycles were averaged. MR was quantified as previously described ( Figure 1 ). Regurgitant volume (RV) and effective regurgitant orifice area (EROA) area were calculated with standard formulas. The biplane method of discs summation (modified Simpson’s rule) was applied to quantify LV end-diastolic and end-systolic volumes and the LV ejection fraction. From the mitral inflow, the E- and A-wave velocities, E-wave deceleration time, and E/A velocity ratio were measured. Left atrial maximal volume was measured using the modified Simpson’s rule. Using color tissue Doppler imaging, peak velocities during early (e′) diastole obtained at the level of septal and lateral annulus were averaged. The E/e′ ratio was then calculated. SPAP was derived from the regurgitant jet of tricuspid regurgitation using the systolic transtricuspid pressure gradient ( Figure 1 ) and the addition of 10 mm Hg for right atrial pressure, as previously performed. Resting PH and exercise PH were defined as SPAP >50 and >60 mm Hg, respectively. Right atrial pressure was assumed to be constant from rest to exercise.
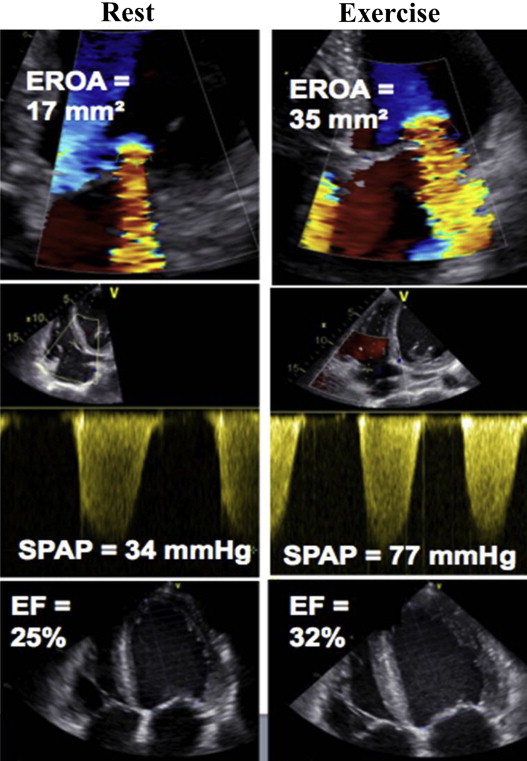
Beta blockers were withdrawn on the day of the test. A symptom-limited graded bicycle exercise test was performed in the semisupine position on a tilting exercise table. After an initial workload of 25 W maintained for 2 minutes, the workload was increased every 2 minutes by 25 W. Blood pressure and a 12-lead electrocardiogram were recorded every 2 minutes. Two-dimensional and Doppler echocardiographic recordings were available throughout the test.
Follow-up information was obtained from interviews with the patients, their relatives, or their physicians. Clinical management was determined independently by the patient’s personal physician using all information available. The primary end point was cardiac mortality. The combined end point included the following: cardiac-related death, heart failure decompensation (worsening heart failure requiring hospitalization, acute pulmonary edema), need for cardiac resynchronization therapy or implantable cardiac defibrillator, or heart transplantation. Nonfatal myocardial infarction was reported in the population with histories of ischemic heart disease but was not considered a PH-related event.
Continuous variables are expressed as mean ± SD, unless otherwise specified. Group comparisons for categorical variables were obtained with chi-square tests and for continuous variables with Student’s t tests. Probabilities of event-free survival were obtained by Kaplan-Meier estimates for the 2 groups and then compared using a 2-sided log-rank test. The impact of exercise PH on the occurrence of the end point was assessed with Cox proportional hazards models in univariate and multivariable analyses. Variables with a univariate p values <0.10 were incorporated into the multivariate models. We used penalized smoothing splines to illustrate the association of SPAP as a continuous variable and the risk for cardiac event. A p value <0.05 was considered to indicate statistical significance.
Results
The demographic and clinical data of the 159 included patients are listed in Table 1 . None of the patients, at the time of the study, had suspected significant coronary artery disease requiring myocardial revascularization. The prevalence of exercise PH was significantly higher than resting PH (40% vs 13%, p <0.0001). There was no significant difference between patients with exercise PH and those without exercise PH regarding demographic and clinical data, as well as baseline medication ( Table 1 ).
Variable | Whole Cohort (n=159) | No Exercise Pulmonary Hypertension (n=96) | Exercise Pulmonary Hypertension (n=63) | p |
---|---|---|---|---|
Age (years) | 65±11 | 64±11 | 66±10 | 0.27 |
Male gender | 105 (66%) | 62 (65%) | 43 (68%) | 0.63 |
Resting heart rate (bpm) | 75±12 | 75±12 | 74±11 | 0.70 |
Resting systolic blood pressure (mmHg) | 128±15 | 127±14 | 129±15 | 0.49 |
Exercise heart rate (bpm) | 113±13 | 114±13 | 113±12 | 0.84 |
Exercise systolic blood pressure (mmHg) | 153±19 | 152±16 | 154±22 | 0.70 |
Smoker | 101 (63%) | 59 (62%) | 42 (67%) | 0.50 |
Hypercholesterolemia | 72 (45%) | 44 (46%) | 28 (44%) | 0.86 |
Hypertension | 70 (44%) | 39 (41%) | 31 (49%) | 0.29 |
Diabetes | 38 (24%) | 75 (78%) | 46 (73%) | 0.46 |
NYHA class III | 38 (24%) | 21 (22%) | 17 (27%) | 0.56 |
Previous myocardial infarction | 129 (81%) | 79 (82%) | 50 (79%) | 0.84 |
Previous coronary artery bypass graft | 28 (18%) | 18 (19%) | 10 (16%) | 0.64 |
Medication | ||||
Angiotensin converting enzyme inhibitor | 125 (79%) | 76 (79%) | 49 (78%) | 0.83 |
β-blockers | 119 (75%) | 71 (74%) | 48 (76%) | 0.75 |
Nitrate | 69 (43%) | 43 (45%) | 26 (41%) | 0.66 |
Lasix | 83 (52%) | 49 (51%) | 34 (54%) | 0.72 |
Aldacton | 48 (30%) | 26 (27%) | 22 (35%) | 0.29 |
In the whole cohort, SPAP significantly increased from rest to exercise (+19 ± 13 mm Hg, p <0.0001). Patients with exercise PH had statistically lower resting E- and e′-wave velocities but higher E/e′ ratios ( Table 2 ). Resting SPAP was significantly higher in the exercise PH group. Of note, there was no significant difference between the 2 groups regarding resting MR severity ( Table 2 ). During exercise, e′-wave velocity was lower in exercise PH group compared with the no exercise PH group, resulting in significantly higher exercise E/e′ ratios. During exercise, MR severity was available (i.e., quantifiable) in 120 patients (75%); MR increased significantly in the whole cohort (EROA +8.5 ± 11 mm 2 , p <0.0001, and RV +12 ± 15 ml, p <0.0001). Exercise ( Table 2 ) and exercise-induced increase in MR severity were significantly higher in patients with exercise PH than in those with no exercise PH (EROA +14 ± 11 vs +5 ± 10 mm 2 , p <0.0001, and RV +19 ± 16 vs +8 ± 14 ml, p <0.0001).
Variable | Whole Cohort (n=159) | No Exercise Pulmonary Hypertension (n=96) | Exercise Pulmonary Hypertension (n=63) | p |
---|---|---|---|---|
Resting Left ventricular and left atrial parameters | ||||
Left ventricular end-systolic volume (mL) | 93±25 | 94±26 | 92±22 | 0.61 |
Left ventricular end-diastolic volume (mL) | 144±30 | 145±31 | 143±28 | 0.72 |
Left ventricular ejection fraction (%) | 36±7 | 35±7 | 36±7 | 0.72 |
Wall motion score index | 1.65±0.27 | 1.65±0.25 | 1.65±0.3 | 0.96 |
E-wave velocity (cm/s) | 69±24 | 72±25 | 65±22 | 0.047 |
A-wave velocity (cm/s) | 62±27 | 63±26 | 60±28 | 0.56 |
e’-wave velocity (cm/s) | 5.0±1.8 | 5.5±1.8 | 4.2±1.4 | <0.0001 |
E/e’ ratio | 15.2±6.8 | 14.2±6.3 | 16.7±7.3 | 0.025 |
Left atrial area (cm 2 ) | 18.7±4.4 | 18.8±4.7 | 18.6±3.8 | 0.78 |
Resting mitral regurgitation severity | ||||
Effective regurgitant orifice area (mm 2 ) | 16±10 | 15±10 | 17±11 | 0.39 |
Regurgitant volume (mL) | 19±13 | 18±13 | 14±19 | 0.25 |
Resting systolic pulmonary arterial pressure (mmHg) | 37±10 | 33±9 | 42±10 | <0.0001 |
Exercise left ventricular and left atrial parameters | ||||
Left ventricular end-systolic volume (mL) | 82±27 | 82±29 | 81±25 | 0.71 |
Left ventricular end-diastolic volume (mL) | 143±32 | 145±34 | 140±29 | 0.27 |
Left ventricular ejection fraction (%) | 43±10 | 44±9 | 43±11 | 0.46 |
Wall motion score index | 1.47±0.32 | 1.47±0.31 | 1.49±0.34 | 0.73 |
E-wave velocity (cm/s) | 100±25 | 99±24 | 104±28 | 0.24 |
A-wave velocity (cm/s) | 67±26 | 71±27 | 59±23 | 0.12 |
e’-wave velocity (cm/s) | 6.3±2.3 | 7.1±2.4 | 5.1±1.5 | <0.0001 |
E/e’ ratio | 18±8.4 | 15.7±8 | 21.5±7.7 | <0.0001 |
Left atrial area (cm 2 ) | 19.9±4.7 | 19.7±4.7 | 20.3±4.7 | 0.46 |
Exercise mitral regurgitation severity | ||||
Effective regurgitant orifice area (mm 2 ) | 25±14 | 21±11 | 32±15 | <0.0001 |
Regurgitant volume (mL) | 32±20 | 27±19 | 40±19 | <0.0001 |
Exercise systolic pulmonary arterial pressure, mmHg | 55±17 | 44±11 | 72±9 | By design |
Exercise induced changes | ||||
Exercise-induced increase in Effective regurgitant orifice area | 127 (79%) | 72 (81%) | 55 (96%) | 0.006 |
Exercise-induced increase in regurgitant volume | 118 (74%) | 67 (77%) | 52 (93%) | 0.013 |
Exercise-induced no or reduced Left ventricular ejection fraction | 6 (4%) | 3 (3%) | 3 (5%) | 0.60 |
In simple linear regression, exercise SPAP was significantly correlated ( Table 3 ) with resting and exercise e′-wave velocity, resting SPAP, exercise E/e′ ratio, and exercise EROA and RV. Of note, exercise-induced changes in SPAP were well correlated with exercise-induced changes in RV and in EROA ( Figure 2 ) Using multiple linear regression analysis, resting SPAP, exercise EROA, and e′-wave velocity were identified as independent determinants of exercise SPAP ( Table 4 ). Similar results were found when exercise RV was included in the model instead of exercise EROA (β = 0.26 ± 0.05, p <0.0001).
Variable | Exercise Systolic Pulmonary Arterial Pressure | |
---|---|---|
r | p | |
Resting left ventricular and left atrial parameters | ||
Left ventricular end-systolic volume | -0.01 | 0.91 |
Left ventricular end-diastolic volume | 0.01 | 0.93 |
Left ventricular ejection fraction | 0.03 | 0.67 |
Wall motion score index | -0.07 | 0.41 |
E-wave velocity | -0.10 | 0.20 |
A-wave velocity | -0.05 | 0.49 |
e’-wave velocity | -0.25 | 0.002 |
E/e’ ratio | 0.13 | 0.10 |
Left atrial area | -0.05 | 0.57 |
Resting MR severity | ||
Effective regurgitant orifice area | 0.16 | 0.84 |
Regurgitant volume | 0.08 | 0.34 |
Resting systolic pulmonary arterial pressure | 0.58 | <0.0001 |
Exercise LV and LA parameters | ||
Left ventricular end-systolic volume | -0.01 | 0.87 |
Left ventricular end-diastolic volume | -0.06 | 0.47 |
Left ventricular ejection fraction | -0.01 | 0.92 |
Wall motion score index | 0.04 | 0.62 |
E-wave velocity | 0.06 | 0.44 |
A-wave velocity | -0.02 | 0.91 |
e’-wave velocity | -0.31 | <0.0001 |
E/e’ ratio | 0.26 | 0.001 |
Left atrial area | 0.04 | 0.67 |
Exercise MR severity | ||
Effective regurgitant orifice area | 0.34 | <0.0001 |
Regurgitant volume | 0.31 | <0.0001 |
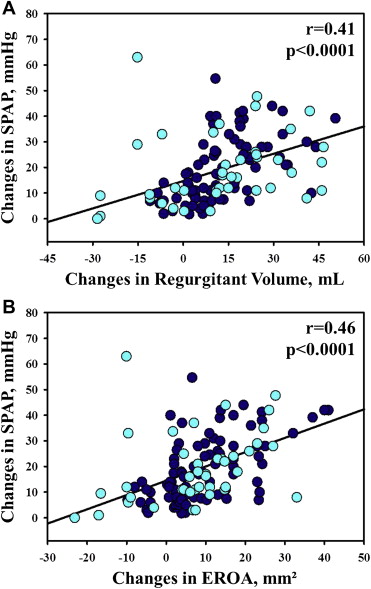
Variable | Exercise systolic pulmonary arterial pressure | ||
---|---|---|---|
β | Standard error | p | |
Age | 0.099 | 0.091 | 0.277 |
Male gender | 1.931 | 2.073 | 0.353 |
Resting systolic pulmonary arterial pressure | 0.947 | 0.096 | <0.0001 |
Exercise effective regurgitant orifice area | 0.578 | 0.089 | <0.0001 |
e’-wave velocity | -1.258 | 0.429 | 0.004 |
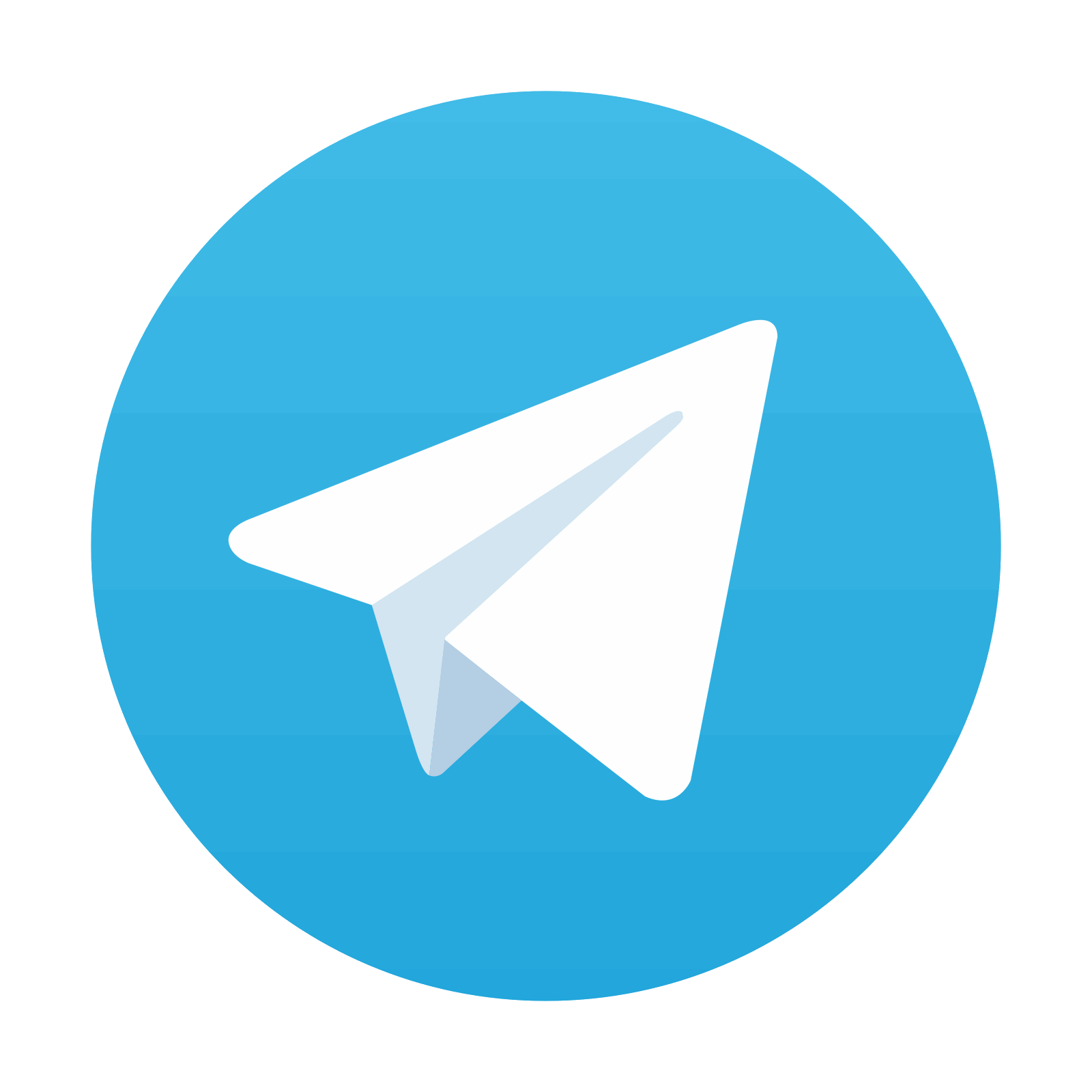
Stay updated, free articles. Join our Telegram channel

Full access? Get Clinical Tree
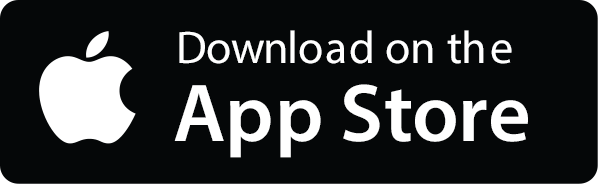
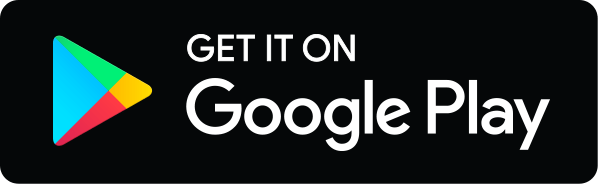
