Score
Clinical description
1
Patient anxious and agitated or restless or both
2
Patient cooperative, orientated, and tranquil
3
Patient asleep, responds to commands
4
Patient asleep, with brisk response to light glabellar tap or loud auditory stimulus
5
Patient asleep, with sluggish response to light glabellar tap or loud auditory stimulus
6
Unresponsive to any stimulus
Richmond Agitation Sedation Scale (RASS)
RASS needs minimal training, and less than 1 min is needed for assessment of the patient using this scale. However, RASS should be determined in the very first assessment of patient arousal state to see if the patient is “alert and calm” equaling “zero.” Then, throughout ICU care, the patient should be reassessed using Confusion Assessment Method in the ICU (CAM-ICU) and be rechecked (Table 9.2). In brief, RASS has ten grades ranging from +4 to −5:
Table 9.2
A summary of the Richmond Agitation Sedation Scale (RASS)
Score | Clinical term | Stimulus |
---|---|---|
+4 | Combative | VOICE (verbal stimulation) |
+3 | Very agitated | |
+2 | Agitated | |
+1 | Restless | |
0 | Alert and calm | |
−1 | Drowsy | |
−2 | Light sedation | |
−3 | Moderate sedation | |
−4 | Deep sedation | TOUCH (physical stimulation) |
−5 | Unarousable |
+4 to +1: combative to restless stages
0 (zero score): “alert and calm” patient
−1 to −5: drowsy to unarousable patient
Sedation Agitation Scale (SAS)
SAS is shorter than RASS; it starts from 1 and leads to 7. SAS has seven scores (without negative scores), while score 4 stands exactly at the middle and is for the “calm and cooperative patient” (Table 9.3) (Riker et al. 1999; Simmons et al. 1999).
Table 9.3
A summary of the Sedation Agitation Scale (SAS)
Score | Clinical term |
---|---|
7 | Dangerous agitation |
6 | Very agitated |
5 | Agitated |
4 | Calm and cooperative |
3 | Sedated |
2 | Very sedated |
1 | Unarousable |
Near Infrared Spectroscopy (NIRS)
Though introduced in laboratory studies for nearly 40 years, this is just about a decade that NIRS has been used in the clinical setting for CNS monitoring.
Nearly 40 years has passed from the time that Professor “Frans Jöbsis” introduced the technology of near infrared spectroscopy (NIRS) for clinical application as a monitor in 1977 (Wolf et al. 2007).
The monitor is at times known as cerebral oximetry because the frontal area of the cortex is the most common site for its application; however, other parts of the body, for example, the flanks, have been used frequently during the previous years as an index of somatic perfusion and oxygenation status; hence, NIRS is often the general accepted term.
NIRS is used in many clinical states and procedures including cardiac surgery; in cardiac surgery, NIRS is an important component of perioperative multimodal CNS monitoring.
Both animal models and human studies have demonstrated its usefulness; though some controversies may exist, including some types of cyanotic patients, these studies at times question the effect of NIRS application on patient outcome (especially the systematic review recently published by Zheng et al. (2013)). Also, other studies have some other considerations especially for pediatric congenital heart surgery patients (Gottlieb and Mossad 2014).
NIRS has these advantages:
Monitoring is noninvasive, providing real-time, continuous data.
Monitors not only the CNS status but also some aspects of hemodynamics since it monitors both oxygenation and perfusion status (i.e., mandates appropriate hemodynamic of CNS).
During all the perioperative period, NIRS has its merits.
During cardiopulmonary bypass or during cardiac arrest, NIRS still works, not needing a pulsatile flow like pulse oximetry.
Technology of NIRS: near infrared (NIR) light in the range of 700–1000 nm is radiated through self-adhesive optodes; then, penetration, passage, and reflection of NIR light are through the skull or other underlying tissues; specific calculations are used for estimation of NIRS. However, the NIR light, when penetrated through, for example, the skull bone, is partly absorbed by biologic chromophores like oxyhemoglobin (OHb), deoxyhemoglobin (HHb), and cytochrome oxidase, while the rest of the light is returned back and is used for data measurement and calculations of the software to demonstrate the final refracted number of the oximetry; the calculations are based on the “modified Beer-Lambert law.”
Light reflection is the basic mechanism of NIRS, while light transmission through, for example, the fingertip is used in pulse oximetry; this technology difference is the reason why we can attach the optodes of NIRS to the skull (for cerebral oximetry) or flank (for somatic and visceral oximetry), finally demonstrating the figures of rSO2 on the monitor screen. The NIRS optodes have a number of “light emitting diodes” (LEDs) (Fig. 9.1).


Fig. 9.1
(a) Schematic drawing of light transmission through skull with two depth of light penetration with resultant regional values; (b): the final figures of NIRS are the result of balance between tissue oxygen supply and consumption; (c) mechanism of NIRS in microvasculature (Published with kind permission of © Medtronic, 2016. All Rights Reserved)
The differential absorption of near infrared light in the range of 700–1000 nm through the skin, bone, or other underlying tissues is used for NIRS.
NIRS compares each patient with himself/herself; it means that for each patient, a baseline is measured at the first time; then, on a real-time basis, any subsequent change is compared with the baseline: more than 20 % change is considered as a significant change needing some intervention to restore NIRS numbers to normal. Also, the absolute number for NIRS is defined from 40 to 90 (Fig. 9.2).


Fig. 9.2
Intraoperative bilateral NIRS for cerebral oxygenation monitoring (Published with kind permission of © Medtronic, 2016. All Rights Reserved)
What Really NIRS Measures
NIRS measures O2 content of the target tissue; the final figures are the result of balance between tissue oxygen supply and consumption (Fig. 9.1); in other words, the trend of NIRS shows the trend of changes in tissue oxygen content. But what factors affect tissue oxygen content?
In the brain tissue, the following factors are among the main ones affecting cerebral oxygen content:
Cerebral tissue oxygen delivery to the organ through the blood which is determined by arterial oxygen saturation, hemoglobin, and arterial oxygen content.
Cerebral hemodynamics. A number of factors alter cerebral hemodynamics:
- 1.
Systolic, diastolic, and mean arterial pressure; hypotension decreases tissue oxygen delivery; carotid stump pressure is the most important pressure.
- 2.
Patency of cerebral arteries due to any underlying obstruction (permanent or transient), for example, extraordinary rotation of the head could affect tissue oxygen delivery, and mechanical impedance against blood flow decreases tissue oxygen delivery.
- 3.
Vasoactive drugs strongly affect cerebral blood and oxygen delivery.
- 4.
Cerebral venous drainage (including any hindrance in cerebral veins) decreases tissue oxygen delivery.
- 1.
Cerebral tissue oxygen expenditure which is determined by cerebral tissue metabolism:
- 1.
Seizure activity or fever increases tissue oxygen consumption.
- 2.
Level of anesthesia (including sedation or consciousness state) affects the level of cerebral activity; the deeper the patient, the less oxygen demand; insufficient anesthesia level increases tissue oxygen consumption.
- 3.
Tissue temperature affects tissue metabolism and hypothermia decreases tissue oxygen demand.
- 1.
Practical notes for using cerebral NIRS: there are a number of notes that one should consider during clinical practice for using cerebral NIRS:
Correct attachment of the probes: the underneath of the probes should be fine with no hair beneath; also, when using two probes, they should be symmetrical.
For neonates and small children, either neonatal probes should be used or one probe should be attached to the frontal area; however, when one probe is used, the differentiation property between right and left perfusion through carotids is not well gained; this is why using separate probes for each side of the frontal area is recommended.
Baseline figures should be taken for each patient and they are very important; each patient is compared with its baseline.
NIRS has been traditionally known as a CNS monitor; however, it has recently been used in pediatric patients and neonates as a somatic monitor; so it could monitor other tissues to check if their oxygenation is appropriate in some parts of the body like splanchnic perfusion, renal perfusion, and spinal cord perfusion (Fig. 9.3). The difference between somatic and cerebral NIRS should not be more than 15–20; increased difference suggests a drop in cerebral tissue oxygen content, and its etiology should be sought. Usually, somatic NIRS reading is higher than cerebral NIRS, but if somatic NIRS drops, there is something wrong in somatic perfusion mandating a revision of whole body perfusion state. This is why both cerebral and somatic NIRS could be used as appropriate surrogates for treatment of perfusion impairments, for both regional and global impairments (Nelson et al. 2008; Murkin and Arango 2009; Tweddell et al. 2010; Gil-Anton et al. 2015).
Fig. 9.3
(a) Intraoperative NIRS demonstrating both cerebral and somatic NIRS; the lower half of the monitor panel shows the results of somatic optodes (S 1 and S 2 ); (b) schematic drawing of cerebral and somatic NIRS (Published with kind permission of © Medtronic, 2016. All Rights Reserved)
When considering the postoperative period, acceptable NIRS results (cerebral and especially somatic) could be good predictors for successful weaning and extubation (Gil-Anton et al. 2015).
In patients with extracorporeal support devices (including ECMO, RV, or LV assist devices), NIRS could be of very good benefit (Papademetriou et al. 2012).
Some species of hemoglobin (like sickle cell) or very high concentration of hemoglobin (e.g., patients with severe polycythemia) might have interference with real results of cerebral NIRS; other factors like skin pigmentation, ambient light, or injected dyes might produce interference (Gottlieb and Mossad 2014).
Underlying cardiopulmonary impairments, anemia, or vascular diseases might be confounders of NIRS readings.
In clinical practice, any decline in regional cerebral NIRS reading often needs an algorithmic approach involving the following steps (Denault et al. 2007; Murkin and Arango 2009):
- 1.
Turn position of the head to a neutral position to relieve any underlying mechanical obstruction due to excessive lateral rotation.
- 2.
Ask surgeon to control the position of arterial and/or venous cannula to relieve obstructions in blood flow.
- 3.
Correct hypotension (especially mean arterial pressure).
- 4.
Diagnose and treat any underlying systemic desaturation (blood gas and/or pulse oximetry).
- 5.
Correct excessive hyperventilation; PaCO2 especially below 35 mmHg should be corrected.
- 6.
Diagnose and treat anemia especially when hematocrit is below 30 %.
- 7.
Check cerebral oxygen consumption state, treat any underlying seizure or convulsive activity, detect any episodes of hyperthermia or fever and treat them, check the level of anesthesia or sedation, and add the depth of anesthesia or sedate the patient more as needed.
- 8.
Diagnose and treat any underlying pump failure, including possible failing heart or inadequate flow of pump or inadequate perfusion by assist devices; modalities like echocardiography, jugular venous blood oxygen saturation (SjVO2), or metabolic assessments like lactate level could be useful guides.
- 9.
Diagnose and treat any possible etiologies causing cerebral edema and/or increased intracranial pressure; diagnose with imaging modalities or intracranial pressure monitors and treat increased ICP with positioning and medical treatments.
Electroencephalography (EEG) Including Traditional EEG, qEEG, and CCEEG
History of EEG
EEG is an invention by the German psychiatrist Hans Berger who described the electrical recordings of the brain in 1926 for the first time; his records are still valid. Nowadays, after decades and also with improvements in this technology, EEG is still a very useful diagnostic and monitoring tool, though it is considered a good CNS monitoring but not a perfect one.
Here we discuss EEG in two main topics:
- 1.
The basic standards of EEG including basic technical requirements and how EEG works
- 2.
Using EEG in perioperative period as continuous CNS monitor
In both of the above segments, we will always consider two age ranges:
- 1.
Adult and older pediatric patients
- 2.
Neonates and small pediatric group
The current discussion on EEG has contributions from two main sources:
The guidelines published by American Clinical Neurophysiology Society (1994, 2006a, b; Herman et al. 2015a, b); list of the guidelines and statements of the American Clinical Neurophysiology Society is available at its website: https://www.acns.org/practice/guidelines.
The guidelines and statements released by American Society of Neurophysiological Monitoring (Isley et al. 2009); their position statements are available at http://www.asnm.org/.
What Are the Applications of EEG in the Perioperative Period?
There are a number of main reasons for using EEG in the perioperative period, including (Isley et al. 2009; Edmonds et al. 2011):
Detection and diagnosis of any underlying CNS pathology or any baseline disorder; for this purpose, it is important to have a baseline EEG for further assessments and documentations; this is especially a major consideration in patients with underlying disease or potential risk factors for ischemia.
Detection and documentation of any new abnormality in CNS function in the perioperative period; these new findings should be diagnosed and if needed, be treated soon.
As a continuous monitoring device and a real-time neurologic assessment tool and a diagnostic tool for detection of seizure or other CNS events like coma, brain death, drug toxicities, or possibility of residual anesthetic effects.
Titrating the dosage of anesthetics and sedatives during perioperative period.
During early rewarming from cardiopulmonary bypass, often an imbalance between brain oxygen demands and oxygen delivery occurs which could be a potential etiology for brain ischemia.
A therapy tailoring guide which is used for monitoring the dosage of anticonvulsants and their efficacy in controlling clinical and subclinical seizure activity; so EEG works as a guide for titration of drug dosage (like anticonvulsant dose) in order to guarantee drug efficacy.
In barbiturate-induced or hypothermia-induced coma, when we need objective confirmation of cortical silence which needs monitoring the efficacy of cerebral protection strategies during the perioperative period.
Tailoring appropriate critical perioperative care in patients at risk of even borderline ischemic events; for example, prevention of adverse effects of hyperventilation on cerebral perfusion, prevention of deleterious effects of acute hemodilution (both intraoperative, during cardiopulmonary bypass, or postoperative), and prevention of adverse effects during rewarming from cardiopulmonary bypass or hyperthermia in postoperative period.
How EEG Works (Including 10/20 System and the Waves)
The standard electrode system is the 10/20 electrode system of the International Federation of Clinical Neurophysiology and American Clinical Neurophysiology Society (Klem et al. 1999; 2006e; Isley et al. 2009).
The main source for EEG waves is the postsynaptic activity of cortical neurons; these neurons, called “pyramid” cortical cells or “Betz” cells, are located in the outermost layer of the brain cortex just underneath and perpendicular to the skull. Having very long axons extending toward the inner parts of the brain, EEG is the summative activity of millions of these pyramids; i.e., only the postsynaptic electrical currents of pyramids (both excitatory and inhibitory functions) accumulate and create EEG waves; however, the axonal activity does not contribute in production of EEG waves. Often, the following characteristics are considered as the main features of EEG waves:
- 1.
Frequency: the number of time that each wave occurs in each second, presented as Hz; each EEG wave is usually consisted of at least two basic waves which are overlapped together and compose the final waves; the composing waves could be analyzed by Fourier analysis; Table 9.4 is a summary of basic EEG waves; we could use this mnemonic for EEG waves GBATDS.
Table 9.4
Normal EEG rhythms; i.e., EEG waves (mnemonics: GBATDS)
Wave category
Symbol
Frequency
Amplitude and/or voltage
Related activity
Clinical equivalent
Gamma rhythm
γ
25.1–55 Hz
High voltage and amplitude
Corticothalamic perception; both during wakefulness and sleep
Engaged in:
Sensory processing activities
Perception process
Beta rhythm
β
12.6–25 Hz
In adults 10–20 μV
Cortico-cortical network
Fully awake patient with:
Open eyes
Mental activity
Alpha rhythm
α
8–12.5 Hz
Relatively high voltage and amplitude: 30–50 μV
In adults: 10–20 μV
Mainly composed of electrical activity emerged from parietal and occipital lobes of the corticothalamic network
Awake, but relaxed individual with closed eyes
Equals drowsy state
Theta rhythm
θ
4–8 Hz
50–100 μV
In adults: 10–20 μV
Often seen in temporal lobes in the awake state but sleepy and relaxed
And corticothalamic activity and limbic activity
Equals stage 2 of sleep (i.e., light sleep) or drowsy state
Also, frequently seen in young children
Delta rhythm
δ
1–4 Hz
High amplitude
100–200 μV
Corticothalamic dissociation
Equals deep dreamless sleep (stage 3 of non-REM sleep); so helps defining the depth of sleep
Another name is slow wave sleep
Also, seen during coma or other brain disorders
Finally, seen in infancy
Slow rhythm
<1Hz
- 2.
Time: is demonstrated on horizontal axis of EEG records.
- 3.
Amplitude: EEG waves have an amplitude between 10 and 100 100 μV, which is about 100 times less than the amplitude of electrocardiography waves; with increasing age, the amplitude of EEG waves decreases.
- 4.
Symmetry: one should always seek for symmetry in EEG waves between the two hemispheres even in an anesthetized patient.
- 5.
Voltage: the EEG electrodes record the difference in their voltage between two electrodes across the time scale; in other words, deflections above or below the horizontal scale are the result of voltage difference between the two electrodes: negative “voltage difference” between the first and the second electrodes would be demonstrated as above the scale deflection (i.e., up deflection), while positive difference between the first and the second electrodes would appear as below the scale deflection (i.e., down deflection) on EEG.
EEG function is composed of thousands of EEG epochs; each epoch is an interval of “few seconds,” which records electrical activity in this time domain and is usually 2–4 s; then, the electrical activity of each epoch is analyzed by the device microprocessor and demonstrated as EEG waves on a time-based scale.
Electrode Arrangement on the Scalp
The standard order of EEG electrode arrangement on the scalp is named montage of electrodes. Basically, four main anatomic landmarks are used for electrode attachment over the scalp:
Anterior location: nasion
Posterior location: occipital or inion
Two preauricular locations
The above locations combined with alphanumeric coding are used to name the standard electrode positions, which help us locate the lesions and, also, to compare similar anatomical points on the two hemispheres (Fig. 9.4):


Fig. 9.4
The EEG electrode arrangement on the scalp
F: frontal electrodes.
O: occipital electrodes.
P: parietal electrodes.
T: temporal electrodes.
C: central electrodes.
A: auricular electrodes.
M: mastoid electrodes.
Even numbers as subscripts demonstrate right hemisphere.
Odd numbers as subscripts demonstrate left hemisphere.
z as subscript demonstrates midline electrodes (z: zero).
Guideline 5 of the American Clinical Neurophysiology Society describes the standard electrode position nomenclature (2006e).
The Standard Electrode System
The standard 10/20 electrode system could be used to have the standard 16 channel systems in recording conventional EEG; for intraoperative or postoperative CNS recording, we usually use 2–8 channels, and even many times during the perioperative period, only three electrodes are used which are attached to the frontal area: two of them are used to record the neuronal electrical activity as “differential amplifier with voltage difference,” while the third electrode is the “mandatory reference signal” electrode, although some authors believe that at least eight channels should be used. How we use the electrodes for electrode montage is described fully in Guideline 6 of the American Clinical Neurophysiology Society: A proposal for standard montages to be used in clinical EEG (2006f).
Grounding Electrode
According to the American Clinical Neurophysiology Society guideline (2008 version), except for a number of situations, we should always use a grounding electrode; these exceptions include those patients in which other electrical equipment are attached to the patient (like operating room or intensive care units); in these latter patients, we should avoid double grounding.
Different types of electrodes are available. However, it is more important to follow instructions for use more than just the electrode type; i.e., a constant type of electrode should be used for electrode montage; for artifact prevention, the electrodes should be well attached, appropriate electrode quality should be guaranteed, and, finally, enough gel should be pasted to prevent any extra resistance and guarantee low amount of impedance. The three electrode types are cup electrodes, silver-silver chloride electrodes, and needle electrodes:
“Cup” electrodes which are usually made from “tin, silver, or gold.”
“Silver-silver chloride” electrodes are the third type of EEG electrodes.
Gold disks or silver-silver chloride disks adhered to collodion are the best options, while other good-quality electrodes are acceptable.
Needle electrodes are not recommended unless in very special condition with related precautions.
All these three types of electrodes should be free of inherent noise; keep electrodes clean to decrease noise.
Besides good quality of electrodes, guaranteed adhesion with enough gel, and keeping electrodes clean, the EEG cables and wires should be avoided from the vicinity of other cables to prevent noise and improve signal quality; shielding the EEG leads also could improve the quality.
Special care should be given to prevent transmission of contagious diseases like HIV, Creutzfeldt-Jakob disease, viral hepatitis, or other similar agents.
Interpretation of EEG
For EEG interpretation, a number of variables should be considered, though this is far beyond the scope of this book; however, a summary of those indices which suggest abnormality is mentioned here:
Asymmetry: there should be symmetry between right and left hemispheres; asymmetry is suggestive of pathology; asymmetry could be sign of ischemia or arterial occlusion.
Spikes: the spike waves are suggestive of seizure activity which may or may not be accompanied with clinical convulsion.
Decreased frequency, decreased voltage, and decreased amplitude: during early stages of ischemia, frequency of waves decreases, while the voltage is preserved; in early ischemia, decreased frequency of waves is seen, while the voltage of EEG waves is preserved; however, with more severe ischemic time, both wave frequency and voltage are depressed; if there is an EEG amplitude drop more than 30 % or there is more than 30 s time interval of EEG changes, it should be considered with great caution as important indicators of ischemia.
Normal EEG waves seen in abnormal states: this could also be a sign of pathology (e.g., if appearance of delta waves in unusual state could be an indicator of a brain lesion).
The abnormal EEG changes are often specifically seen as significant decrease in “beta and the alpha” waves. Also, the following abnormal characteristics are seen in these patients:
Newly occurred abnormalities are often seen in the left hemisphere in the postoperative period.
More invasive, more complex, or longer procedure creates much severe postoperative changes.
Postoperative CNS ischemia (CBF < 22 mL/100 g/min) changes EEG waves from normal to ischemic pattern; this ischemic pattern occurs whenever cerebral blood flow drops below this critical threshold.
One should always consider the effects of altered hemodynamic status, temperature, and anesthetic drugs on EEG findings especially when interpreting abnormal waves.
Injuries due to cardiac surgery are similar in EEG to “organic brain syndrome” findings.
How to Report EEG
Based on Guideline 7 of American Clinical Neurophysiology Society, each report is recommended to be prepared under four main subclasses; a full description could be found in the guideline text (2006g); however, briefly speaking, the following items are the main components of an EEG record:
- 1.
Basic patient information: including age, sex, name, and EEG identification number.
- 2.
Introduction of report: how the patient was prepared, including any received drugs for sedation, sleep deprivation, fasting state, how many electrodes from the standard 21 electrodes of the 10/20 system were used, and how long was the total recording time.
- 3.
Description of the report: should include all findings, both normal and abnormal, away from interpretation; this interpretation should include all the findings in such a way that whenever another clinician reads the record, they could reach a decision even without looking at the EEG recordings.
- 4.
Interpretation of the report: interpretation should be prepared having these two parts: impression and clinical correlation.
Impression is the subjective finding of the interpreter about normality or abnormality of EEG; it should not be too long, and if it has any abnormality, it should contain the main 2–3 findings.
Clinical correlation is the other part that should be mentioned in interpretation of EEG, and it should correlate the EEG findings with clinical picture. If the EEG findings are mild, the phrase minor irregularities in cerebral function is suggested in the report; however, if it is more than mild, the term cerebral dysfunction is appropriate for the interpretation of the report. These terms are appropriate for using in the record: findings of EEG are consistent with the diagnosis, or supportive of the diagnosis, or compatible with clinical findings. If EEG findings are not compatible with clinical findings, it should be stated cautiously, in order not to directly question the clinical diagnosis. Also, if any medication has been used before EEG, it should be mentioned in the interpretation. Some samples of EEG could be added to the record, especially when using electronic recording.
Limitations of EEG
There are a number of limitations for EEG, and this is why we believe EEG is a good CNS monitoring but not a perfect one (Constant and Sabourdin 2012):
EEG works through electrodes which are usually attached to the scalp. These electrodes report the electrical activity of the cortex, i.e., the neurons located just beneath the cortical layer; however, this electrical activity does not include the full activity of the subcortical parts of the brain including the nuclei; hence, it could not alarm ischemia in subcortical regions.
Using conventional EEG in the perioperative period, especially inside the OR, is a difficult task due to its technical complexities; it is sometimes really cumbersome and inconvenient, though newer modalities of EEG are much more used especially in the postoperative period.
EEG is a biorhythm; so it will be affected like other biorhythms like age, environment, and circadian variations.
Ischemia in the perioperative period does not always present itself in the same manner; in other words, if the inhibitory neurons of the CNS suffer an ischemic insult, the result in EEG would be as EEG overactivity.
EEG could detect abnormalities including ischemia; however, it could not detect location of ischemia (ischemic site), definite mechanism of ischemia (the exact etiology), or the anatomic zone of ischemia (the scope of injury).
EEG Considerations in Pediatric and Neonatal Group
Amplitude Integrated EEG: aEEG
This version of EEG is used much more recently in critical care of CNS; aEEG is a bedside tool for neurophysiologic assessment and uses fewer channels than a standard EEG; so aEEG is much easier, both regarding its use and interpretation, and could help earlier diagnosis much more than conventional EEG; however, standard EEG is more sensitive for the diagnosis of seizure. However, it is not mandatory that aEEG be interpreted by a neurologist. Of course, standard EEG remains the decisive method of diagnosis for neurophysiologic assessments. In neonatal ICU, unless we use aEEG in NICU, a considerable part of patients with neonatal seizure would be undetected (Boylan et al. 2015; Kang and Kadam 2015).
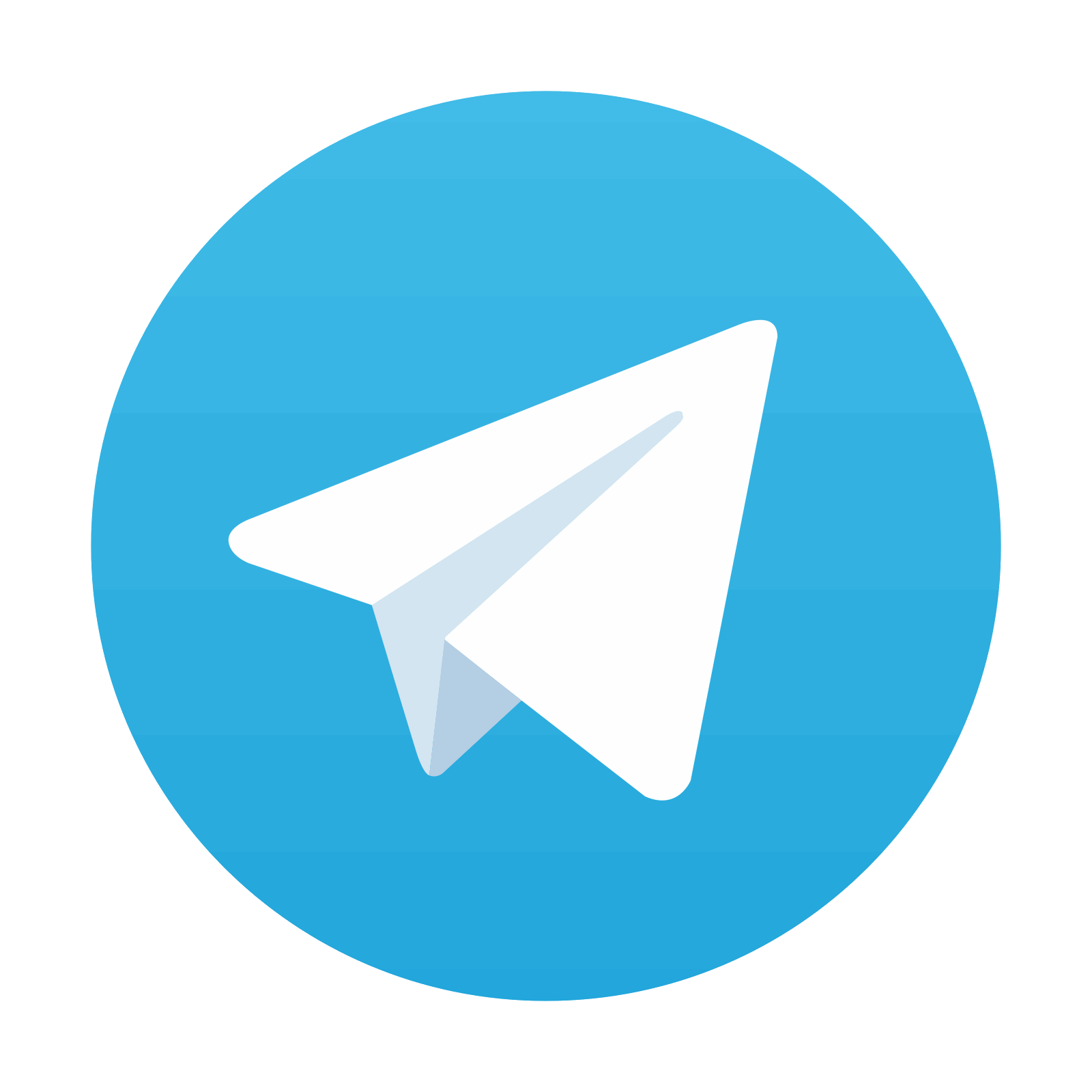
Stay updated, free articles. Join our Telegram channel

Full access? Get Clinical Tree
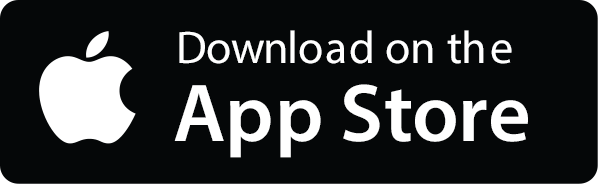
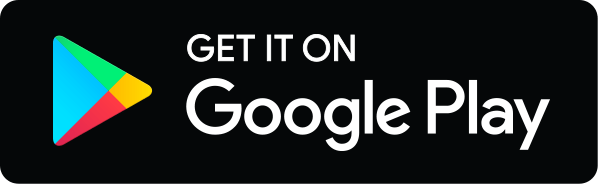