Fig. 33.1
Total cardiopulmonary bypass showing the venous cannulas in the superior and inferior vena cavae with constrictions placed around the respective veins. The venous blood is draining to the oxygenator and is propelled by the pump into the distal ascending aorta to maintain perfusion of the entire body. A cross-clamp is applied to the ascending aorta, and all chambers of the heart therefore are excluded from the perfusion system. Note that modern day systems often place the pump ahead of the oxygenator such that blood from the reservoir is actively pumped through the oxygenator. Ao aorta, RA right atrium, V ventricle
The breakthrough technologies that first allowed this type of open-heart operation were developed by two separate centers in the USA in the early 1950s. Importantly, Lillehei and Varco [1] at the University of Minnesota developed a cross-circulation technique . This technique utilized a human donor (usually the parents of a child undergoing cardiac surgery) who, in essence, functioned as an extracorporeal pump for the patient’s circulatory system. This type of extracorporeal circulation also allowed the blood to be drained from the child’s vena cava so that the surgical procedure could be performed within the empty heart. The subsequent development of the heart–lung machine by Gibbon [2] was considered revolutionary in that it eliminated the need for a support donor (a second patient). Gibbon’s system has been improved since the mid-1950s and has gradually evolved into the standardized, but very complex and sophisticated machine it is today. The bubble oxygenator developed by DeWald and Lillehei in 1955 was additive technology that also aided in the advancement of this field (see also Chap. 25).
The basic components of an extracorporeal circuit include: (1) a reservoir into which the patient’s blood is diverted; (2) an oxygenator that replaces the function of the lungs; and (3) a pump that propels the oxygenated blood back into the patient’s arterial circulation. In this manner, the machine bypasses both the heart and the lungs while maintaining the function of other organs during surgical interventions within the heart.
A solid understanding of cardiopulmonary bypass and ways to control the patient’s physiology is just as important as efficient and meticulous techniques to achieve the best outcomes in cardiac surgery. Today, dedicated perfusionists work closely with the surgical team to ensure that bypass runs properly; they rely on detailed communication from the operative field. This chapter details the components of cardiopulmonary bypass.
33.1.1 Venous Drainage
The venous blood that is normally delivered to the right atrium is commonly diverted to the heart–lung machine, either by cannulating the veins themselves or by cannulating the right atrial chamber. Surgery performed in or through the right atrial chamber requires that both the right atrium and right ventricle be empty. To do so, cannulas are typically placed directly into the superior and inferior vena cavae. Constricting tourniquets are then placed around the vein over the cannulas, and thus blood is diverted into the heart–lung machine (Fig. 33.1). This constitutes total cardiopulmonary bypass.
Cannulation of the superior vena cava (SVC) is normally performed by placing a purse string suture either directly in the SVC or in the right atrium. The cannula is advanced either directly into the SVC or indirectly through the right atrium. It should be noted that direct cannulation of the SVC generally provides more room for any work that may need to be done inside the right atrium. Conversely, for large ascending aortic aneurysms it may be necessary to advance the cannula indirectly through the atrium.
Cannulation of the inferior vena proceeds through purse strings placed on the inferior portion of the right atrium. By placing tourniquets around both cannulas and snaring down on them, the surgeon establishes total cardiopulmonary bypass. The only flow that enters the right atrium is that from the coronary sinus, i.e., until the aorta is clamped. With the aorta cross-clamped and no flow entering the aortic root and coronary arteries, the surgeon can work inside the atrium in a completely bloodless field. Typically, access to the left atrium is obtained by opening the fossa ovalis. A bloodless field here allows insertion of the retrograde coronary artery catheter directly into the coronary sinus for direct cardioplegia, as discussed later.
A modification of this type of bypass can be used when the cardiac chambers are not surgically entered, such as in coronary bypass operations involving procedures on the surface of the heart. In such cases, a double-staged cannula is placed with the tip of the cannula in the inferior vena cava and the side drainage holes positioned at the level of the right atrium. Coronary bypass surgery does not involve direct vision of the inside of the cardiac chambers so there is no need to constrict the superior or inferior vena cava. This configuration is often referred to as full cardiopulmonary bypass rather than total cardiopulmonary bypass. When coronary bypass operations are undertaken simultaneously with cardiac valve repairs or replacements, total cardiopulmonary bypass is typically implemented.
If venous drainage is not optimal, then two critical issues may result: (1) the heart may become distended with warm systemic blood, resulting in persistent myocardial activity or undue strain on the myocardium leading to injury; and (2) venous congestion may result in hepatic impairment. Thus, adequate venous drainage must be ensured prior to proceeding with these types of operations.
33.1.2 Arterial Return
Once the blood has been oxygenated in the heart–lung machine, it is returned to the patient’s general circulation via cannulas placed directly in the arterial system (Fig. 33.1). The most common method involves the placement of a cannula in the highest portion of the ascending aorta below or at the origin of the innominate artery. This is typically the first cannula that is inserted. The aorta must be free of calcific disease in order to cannulate it without increased risk of stroke. The axillary artery is the next best alternative in the setting of calcific aortic disease and/or for cases requiring circulatory arrest for replacement of all or parts of the aortic arch [3].
Depending on the type of surgery, other sites are also used, including cannulation of the femoral artery in the groin and infusion of the arterial system in a retrograde manner. This is useful for minimally invasive heart surgery in younger patients without calcific disease in whom the thoracic incision does not allow exposure to the ascending aorta. Yet, if calcific disease is present the retrograde flow can shower plaque up toward the carotids with increased risk of stroke.
Once the arterial cannula is secured and both the forward flow and venous drainage are adequate, the ascending aorta is cross-clamped. At this point no systemic blood enters the coronary artery circulation. The heart is, therefore, totally excluded from the circulation. Thus the heart needs to be protected by using one of a number of methods to infuse cardioplegic solutions (see Sect. 33.2). Any blood remaining in the operative field is removed via cardiotomy suction lines which are used to aspirate it back to the heart–lung machine, where it reenters the bypass circulation with the rest of the removed blood.
In some operations involving the descending thoracic aorta, total cardiopulmonary bypass is not necessary. For example, if the portion of the aorta that needs to be isolated lies between the left carotid artery and the diaphragm, only part of the total blood volume needs to be removed, and partial bypass can be implemented (Fig. 33.2). The blood is removed by the heart–lung machine via a cannula inserted into either the left atrium or left superior pulmonary vein. Then, the blood is infused back into the descending thoracic aorta beyond the level of distal aortic cross-clamp. Doing so allows the heart to continue to beat normally and helps maintain the viability of the proximal organs (head, neck, and arms), while the rest of the lower body is perfused and thus maintained by the pump. This technique is called left heart bypass because it involves only removal of blood and decompression of the left-side cardiac chambers. As shown in Fig. 33.2, after a descending thoracic aorta aneurysm operation is completed, the bypass is discontinued. The clamps which were placed to occlude the aorta in the arch and the descending portion are removed. The normal physiological perfusion of the body (which was interrupted during surgery without ever stopping the heartbeat) is thus reestablished.
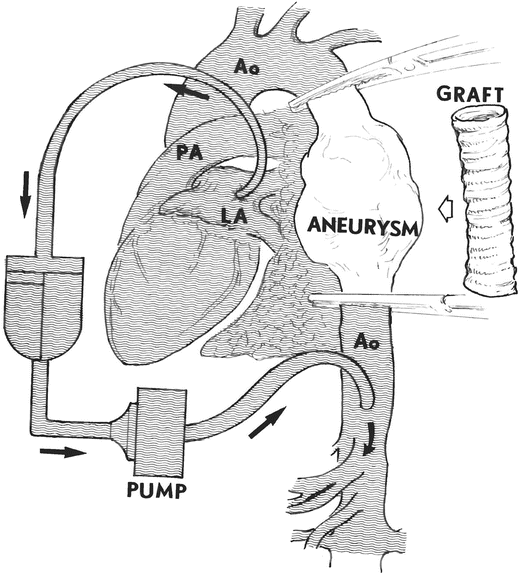
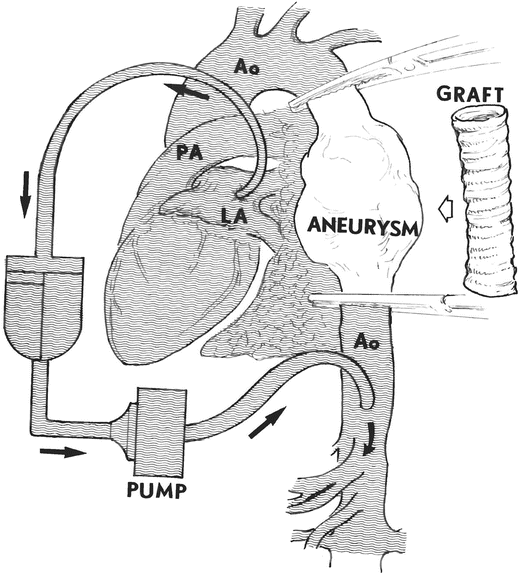
Fig. 33.2
Left heart bypass showing a cannula inserted in the left atrium draining approximately half of the cardiac output into the oxygenator through the pump, and reinfusing it in the distal aorta for perfusion of the abdominal organs. The excluded portion is only the descending thoracic aorta (between clamps). The left heart continues to beat and pumps half of the cardiac output to the head and upper organs. The graft is shown in the position in which it will be implanted after the aneurysm is resected. Ao aorta, LA left atrium, PA pulmonary artery
After an operation applying full or total cardiopulmonary bypass and cardiac arrest, the aortic clamp is released, allowing the general circulation to reperfuse the coronary arteries and to rewarm the heart. After the air is expelled from the cardiac chambers, the heart often elicits ventricular fibrillation. Such fibrillation normally requires cardioversion with an electric shock administered directly to the heart by employing paddles that deliver currents that vary from 20 to 30 W/s. The patient is then ventilated using the endotracheal tube connected to the anesthesia machine, which reinflates the lungs. After the normal sinus rhythm of the heart is reestablished, the patient is gradually weaned off the extracorporeal circulation until the heart takes over full function. At this point the heart–lung machine is stopped, and all cannulas are removed and access areas closed
In some complex surgical cases involving the aortic arch, separate independent perfusion of the arch vessels may require implementation, that is, in addition to the perfusion of the lower part of the body through the cannula inserted in the femoral artery. This is most commonly seen in situations where the arch and descending aorta are bypassed simultaneously. The perfusionist must monitor two separate infusions to regulate pressures and make certain that a balance and sufficient perfusion is achieved in both the upper and the lower areas of the patient’s body. With the advent of staged aortic procedures such as the “elephant trunk procedure” and hybrid stent grafting, it is less common to deal with such an extensive amount of aortic replacement in a single setting [4].
Another specialized bypass method that needs description is the deep hypothermia and total circulatory arrest technique . This type of total cardiopulmonary bypass requires decreasing body temperature to very low levels (15°–25 °C); this is typically accomplished using heat exchangers installed in the heart–lung machine circuits. Circulation is stopped altogether when the proper temperature is reached, and the heart is emptied for several minutes with the entire volume of the patient’s blood remaining in the reservoir of the heart–lung machine. The pump is then stopped and the arterial perfusion ceases. The venous return line, however, is left open to continue emptying the patient’s blood volume completely into the reservoir of the heart–lung machine.
This technique is used in special cases to allow repair of very complicated conditions. The period of total circulatory arrest induced during deep hypothermia is usually less than 45 min [5, 6]. This time restriction is to insure that the patient does not suffer neurological deterioration or central nervous system damage during such global ischemia. In general, cooling alone is sufficient for circulatory arrest times less than 20 min. However, since the time required to perform the aortic arch repair can be unpredictable, most surgeons will utilize some sort of brain perfusion strategy. Retrograde brain perfusion is commonly used for periods of 30–45 min. It requires the arterial outflow from the pump to be connected to the SVC cannula either directly or through a separate circuit. By snaring the SVC, oxygenated blood is delivered up the vena cava to perfuse the brain in a retrograde fashion. The flow rates are typically 300–500 mL/min and the central venous pressure is kept no higher than 35 mmHg.
Antegrade brain perfusion is more typically used for planned circulatory arrest periods ranging 45–90 min. In this mode of perfusion, the axillary artery graft is used to deliver oxygenated blood up the carotids once the innominate artery is snared; typical flow rates vary between 500 and 1000 mL/min. The patient should have some evaluation of the brain vessels beforehand to ensure a complete Circle of Willis, an anastomotic system of arteries that sits at the base of the brain.
As soon as the repair is completed, normal cardiopulmonary bypass is reestablished. The patient is gradually rewarmed to a normal core temperature of 37 °C prior to removal from extracorporeal circulation. The use of deep hypothermia always requires careful evaluation by the surgical team. In such clinical cases, the danger of inducing neurological damage must be weighed against the benefits of correcting a major cardiac anomaly. Conversely, the long-term quality of the arch repair should not be compromised by concerns over circulatory arrest, especially with proper usage of selective brain perfusion techniques.
It is important to note that aortic cannulation can have important consequences (pitfalls) associated with it. The patient must be completely anticoagulated before instituting flow through the cannula. If there is calcific disease, cannulating or clamping the aorta has been associated with increased incidences of stroke or distal embolic events. Finally, during aortic cannulation, there is commonly a 1–2 % incidence of aortic dissection related to a tear caused by the cannula. If such a condition is suspected due to high line pressures or enlargement of the ascending aorta, the flow through the cannula should be immediately stopped; a transesophageal echo can be used to confirm the diagnosis, and then an alternative cannulation strategy should be identified. This situation often requires circulatory arrest to repair the tear.
33.1.3 Anticoagulation
To prevent the formation of clots during cardiopulmonary procedures, both within the body and within the extracorporeal heart–lung machine, it is necessary to anticoagulate the patient’s blood. The most common agent used for such anticoagulation is heparin. It is commonly administered intravenously before cannulation at a dose of 300 units/kg. There are two types of heparin : (1) the lung beef type which is extracted from a bovine source; and (2) the porcine mucosal type which is from a swine source. Since the mid-1980s, the porcine mucosal heparin has been preferred because it is less likely to lead to thrombocytopenia and/or the production of heparin antibodies in the patient, a condition known as HIT syndrome [7].
A small percentage of patients have experienced heparin-induced thrombocytopenia (HIT) from prior heparin exposure. They have antibodies to heparin molecules which attack heparin-platelet aggregates and this, in turn, depletes their platelet pool to dangerously low levels.
The effectiveness of anticoagulation therapy requires testing, usually by measuring the activated clotting time (ACT) of the patient’s blood. These test results are expressed in seconds, with normal values ranging between 100 and 120 s. Heparinization is deemed adequate for cardiopulmonary bypass when the ACT runs above 400 s. Typically, the anticoagulant effects induced during such surgeries must be reversed postoperatively. Still today, porotamine sulfate is the drug of choice to neutralize the effects of the heparin and allow the patient to elicit normal clotting values. Yet, this drug is a macromolecule compound that may produce pulmonary vasoconstriction and severe hypotension [8, 9], particularly in diabetic patients. Nevertheless, such side effects are rare and, in most patients, this drug can be used safely, as it neutralizes the effects of heparin. Naturally, the amount of protamine necessary to achieve neutralization depends on the amount and timing of therapeutic heparin administered. Initially, a test dose is given; if no reaction occurs, protamine is then administered in the appropriate amounts. Its effects are monitored by measuring ACTs until they return to a normal range. It should be noted that if any reaction or side-effects occur, additional treatments are commonly employed, such as the administration of epinephrine, calcium, steroids, or fluids [9].
Occasionally patients cannot be given heparin because they have developed heparin antibodies from previous exposure. Other anticoagulant agents are studied and occasionally used in such cases, including hirudin (Lepirudin) [10], a potent anticoagulant that is extracted from leaches and lampreys. Other drugs include the heparinoids [11] like Orgaran (Org10172, Organon Company, West Orange, NJ, USA), for which a different monitoring protocol is implemented. Unfortunately, to date, no drug has been identified that can reverse the effects of Orgaran , thus it must be metabolized by the human body. For such patients bleeding is a constant, and often very difficult, postoperative complication. Bivalrudin (Angiomax) is currently the most commonly used agent for cardiac surgery in patients with HIT [12].
If the cardiopulmonary bypass takes an extended time, coagulopathies often pose complications. In such cases, the body, primarily the liver, is unable to produce the appropriate clotting factors to reverse the anticoagulation status. Other factors that can contribute to coagulopathies include ischemia of the abdominal organs, particularly if necrosis occurs in the liver cells and/or in the intestine. Bleeding, therefore, can be a very serious and difficult complication to treat; in such patients, the administration of multiple coagulation factors, platelets, and cryoprecipitates may be required.
33.1.4 Temperatures of Perfusion
Since their inception, cardiopulmonary bypass and extracorporeal circulation have been implemented using some degree of hypothermia. Lowering core body temperature decreases the overall oxygen demands of body tissues, and a more desirable protective state during pulseless circulation is provided by the heart–lung machine.
Several degrees of clinical hypothermia are commonly identifiable relative to extracorporeal circulation interventions. Normothermia indicates that core body temperature is between 35.5° and 37 °C [13], mild hypothermia is between 32° and 35 °C, and moderate hypothermia is between 24° and 32 °C. An important distinction must be made between mild and moderate hypothermia. If the heart is perfused at mild levels (above 31 °C), the heart will continue to beat although at slower rates. Therefore, this mild level of hypothermia allows surgical correction of some congenital anomalies without arresting the heart. An additional level of hypothermia used occasionally is deep or profound hypothermia , which usually brings the body temperature below 20 °C.
Currently, most open-cardiac operative procedures are conducted under conditions somewhere between moderate and mild hypothermia. Some centers routinely use moderate hypothermia, while others employ normothermia [14, 15]. One reason to maintain normothermic perfusion is to avoid coagulopathies that may develop when body temperature is lowered to the moderate levels, and thus allow for normal function of the body’s enzyme systems. Normothermic temperatures also enable the kidneys to respond better to diuretics.
Several reports have indicated the relative safety of normothermic perfusion [13–19], but an equal number have suggested complications with this modality [20, 21]. As a result, the spontaneous drifting to mild hypothermic levels is generally preferred. Deep or profound hypothermia is associated with the implementation of total circulatory arrest as mentioned before. With this level of hypothermia, body temperature is lowered to between 15° and 18 °C. Such operations are usually prolonged given the time it takes to cool the body to those levels before surgery and also by the required time to rewarm it afterwards. The goal of systemic cooling is not so much to decrease metabolic demands in the peripheral organs, but rather to drop the temperature of collateral arterial flow that reaches the heart such as the bronchial arteries. If one stays normothermic and has difficulty maintaining cardiac arrest, it is likely that the warm bronchial artery flow is making its way through the pulmonary arteries or veins and warming the inside of the heart.
33.1.5 Perfusion Pressures
Under normal physiological conditions, the heart provides a pulsatile pressure and flow. The systolic pressure depends on the ventricular function. The diastolic pressure in normal states is primarily regulated by the blood volume and the vascular tonus (the degree of constriction experienced by venous vessels relative to their maximally dilated states). During cardiopulmonary bypass , the heart–lung machine facilitates pulseless perfusion; there is no systolic or diastolic pressure, but rather one steady mean pressure throughout the arterial circulatory system. Therefore, this pressure should be high enough to provide adequate blood oxygen to all organs of the body, particularly the brain and kidneys. Since the patient is typically hypothermic, the oxygen requirements are lower; the perfusion pressure is usually maintained around 70 mm of mercury Hg. Occasionally, specifically in patients with severe obstructive carotid disease, a higher perfusion pressure is recommended to ensure proper perfusion of the brain. Nevertheless, this recommendation is somewhat debated because the brain is known to have its own regulatory system to maintain low resistance near obstructed areas [15, 17]. A useful rule of thumb is to keep the mean arterial pressure close to the patient’s decade of life (i.e., 74 years old = 70 mmHg, 86 years old = 80 mmHg).
During cardiopulmonary bypass, if the patient shows decreased vascular tonus (despite adequate volume of fluid), vasoconstrictors are routinely used; a typical therapy is a bolus or drips of neosynephrine [13]. A decreased vascular tonus is common in septic patients with bacterial endocarditis, for whom an emergency operation sometimes is necessary to replace the affected valve and reverse the profound heart failure. Remember that the perfusion pressure is a product of the cardiopulmonary flow and resistance. If the flow has been optimized (ideal flow = 2.4 × body surface area) then the resistance needs to be increased to meet the desired perfusion pressure. Markers of end organ perfusion during bypass include: urine output, mixed venous saturation, lactic acid, and base deficit.
33.1.6 Hemodilution
Up to a certain level, hemodilution can be a desirable side effect of cardiopulmonary bypass. Lowering the hematocrit prevents clumping of the red cells or sludging, thereby providing better circulation at the capillary level; viscosity of the circulating blood is decreased, on the other hand, to also ensure that oxygen is adequately delivered to the body’s tissues during cardiopulmonary bypass. Hematocrit levels are monitored and maintained at a minimum between 22 and 26 %. Toward the end of the bypass operation, typically the perfusionist deliberately removes some of the fluid from the patient’s circulation to hemoconcentrate the blood toward more normal hematocrit levels [22, 23]; this rises to above 30 % by the time the patient is removed from cardiopulmonary bypass. Subsequent diuresis and/or removal of red blood cells (RBC) will further aid in reestablishing the hematocrit to normal levels.
Transfusion of RBC is sometimes necessary if the hematocrit cannot be maintained above 22 %. Risk factors for a low hematocrit on bypass include: (1) the female gender; (2) older age; (3) lower body mass index; (4) a high New York Heart Association class and combined valve and coronary bypass procedures; and/or (5) anemia, the strongest risk factor [24].
It is important to note that there are multiple problems associated with RBC transfusion including: (1) increased renal dysfunction; (2) arrhythmias and infections; and, (3) increased long-term mortality [24]. Interestingly, anemia and RBC transfusions for the bypass patient have additive adverse effects [25]. Therefore, every effort should be made to prevent severe anemia without transfusion, including: (1) use of smaller bypass circuits and less priming volumes; (2) proper preoperative hemoglobin optimization; (3) increasing RBC transfusion thresholds; (4) retrograde autologous priming; and (5) meticulous hemostasis [26].
Pulseless perfusion , as provided by the heart–lung machine, and hemodilution will both invariably lead to a transfer of fluid across the capillary walls into the third space (interstitial). Therefore, all patients develop, to some degree, peripheral third spacing or edema, which often develops within the first 24–72 h after bypass. This usually manifests as pulmonary edema, pleural effusions, atrial fibrillation, and lower extremity edema. This is why patients often require diuresis on day 2–4 following bypass. In an attempt to avoid edema, plasma expanders (such as albumin, hetastarch, dextran, and mannitol) are usually added to the priming solution of the heart–lung machine.
33.1.7 Heart–Lung Machine Basics
The basic components and sequence of modern day heart–lung machines are as follows: venous drainage (vacuum assist) → reservoir → perfusion pump → oxygenator (with heat exchanger) → arterial line filters → aorta. The reservoir of the cardiopulmonary bypass machine is important for proper drainage and emptying of the heart. Without the reservoir, the blood in the human body and the blood in the machine would be in a constant equilibrium of roughly 1:1. With the reservoir, approximately 100 mL to 2 L of volume can be housed outside of the body during perfusion. This negative volume balance drains the human venous capacitance system and leaves the heart flat and empty. An empty heart does little work and consumes minimal oxygen. This is different than the modern day extracorporeal membrane oxygenator circuits which do not have a reservoir and do not allow decompression of the ailing heart.
Although the classic heart–lung machine used gravity to drain the venous blood into the reservoir, modern machines like the Performer CPB (Medtronic, Inc., Minneapolis, MN, USA) (Fig. 33.3) employ an active vacuum in a very small system that reduces the need for large volumes in the reservoir [27, 28]. The Performer CPB is smaller in size and can be placed closer to the operating table, saving tubing length, and it accommodates to any position. In addition, the tubing used for the heart–lung machine where the blood circulates has undergone significant improvement with the use of Carmeda [29, 30], which is a bioactive surface with which the inner side of the tubing is coated. Carmeda is bonded to the wall tubing, mimicking the human endovascular endothelium to reduce coagulation and inflammatory responses of the patient’s body, due to the blood-material surface interaction. The Performer CPB machine displayed in Fig. 33.3 incorporates multiple safety features consisting of alarm sensors for air bubbles, debris, pressure changes, and temperatures, making its use practically fool proof for protection of the patient.
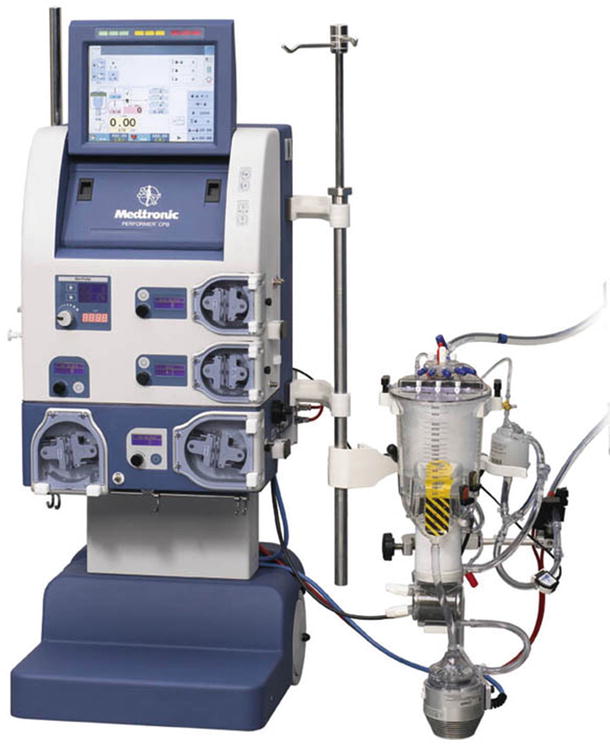
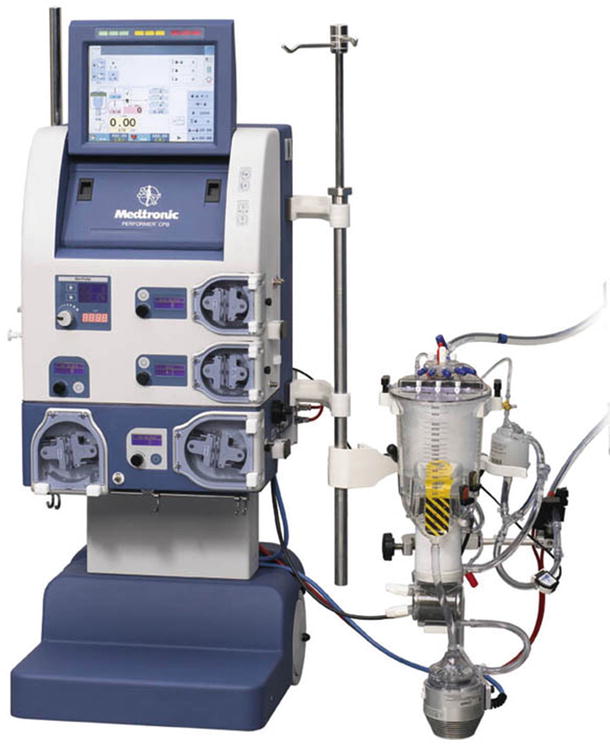
Fig. 33.3
Performer CPB cardiopulmonary bypass machine manufactured by Medtronic, Inc. (Minneapolis, MN, USA) shows a smaller size adjustable to any position. Attached to the machine is the Resting Heart Device which eliminates most of the large size reservoirs. It contains the filters and monitoring devices. All tubing is coated with Carmeda (see description in the text)
In addition, the newer centrifugal pumps (Fig. 33.3), like the Bio-Medicus and the Performance CPB (both from Medtronic, Inc.), offer a distinct advantage over the older roller-type pumps such as the standard DeBakey type. More specifically, the roller pumps use occlusive pressure to propel the blood within the tubing, and can cause damage to the RBC and dislodge debris from the tubing material. In contrast, the newer centrifugal pumps minimize trauma to the RBC because the motion required to move the blood does not constrict the tubing. Although the bubble oxygenator has been used for many years, it has been largely supplanted by the membrane oxygenator. The membrane oxygenator is associated with less trauma to RBC and is less likely to produce micro-bubbles that might pass into the patient’s arterial system and cause air embolism.
As the blood is oxygenated, it passes through heat exchangers that cool or rewarm it as necessary for a given stage of the operation. Typically, one heat exchanger provides temperature control for systemic perfusion to the patient’s body, whereas a second exchanger controls the temperature within the cardioplegia line. In most current systems, the temperature within each circuit is separately controlled by a central regulatory unit. The blood is then filtered which helps prevent microembolization when it is returned to the patient’s arterial system. In addition, two suction lines are employed to aspirate any blood from the operative field, thus recovering and returning the blood to the reservoir where it is oxygenated before being pumped back into the patient’s arterial system.
Importantly, the perfusionist needs to monitor the general circulation flow, electrolyte parameters, anticoagulation parameters, and the ultrafiltration system (which extracts fluids from the patient’s arterial system to avoid over hydration). The perfusionist is also responsible for maintaining the proper pressures within each circuit and monitoring the temperature of the cardioplegic solution that will be used to protect the heart during the period of its exclusion from general circulation. Normally, at 15- to 20-min intervals, the perfusionist apprises the surgeon of the elapsed time of perfusion and reinfuses the heart as necessary to maintain a temperature within the appropriate range (below 15 °C). The perfusionist also remains in direct communication with the anesthesiologist to coordinate administration of any drugs or any other action necessary to maintain the balance of the patient’s other organ systems. Finally, at the end of cardiopulmonary bypass, the perfusionist administers protamine in an amount sufficient to neutralize the effects of the heparin, thus returning the patient’s coagulation system to normal function.
At the conclusion of the surgery, cardiopulmonary bypass is discontinued and the patient’s heart resumes systemic blood circulation. A small volume of blood often remains in the pump and needs to be reinfused into the patient. This remaining blood is sometimes reinfused directly from the reservoir or it may be concentrated and reinfused later.
33.1.8 Heart–Lung Machine Priming
Before cardiopulmonary bypass is undertaken, the heart–lung machine needs to be primed. For an adult patient, a typical 1500 mL of priming fluid is primarily a basic crystalloid solution. Plasmalite is the preferred crystalloid solution to which albumin or hetastarch (about 500 mL for a normal sized patient) is typically added. Doing so helps maintain osmolality and volume in the intravascular space, and helps prevent peripheral third spacing and edema. Red cell sludging usually is prevented within the system by the addition of extra heparin, however with the new systems like the Performer CPB, this is no longer required. In the pediatric patient, ideally one should have much less volume than what is required in adults. In such cases, the so-called Resting Heart System provided by Medtronic, Inc. (Fig. 33.4) can be integrated into the regular Performer CPB system to significantly reduce the entire volume needed for priming the pump. This integrated unit also provides for an automatic venous air removal and integrated air removal from the cardioplegic system. As mentioned before, at the end of the perfusion the blood is hemoconcentrated to normal levels by eliminating the extra fluid from the circulation; all the air is eliminated as well.
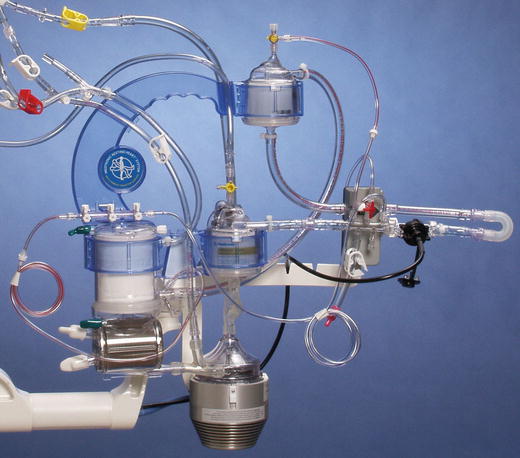
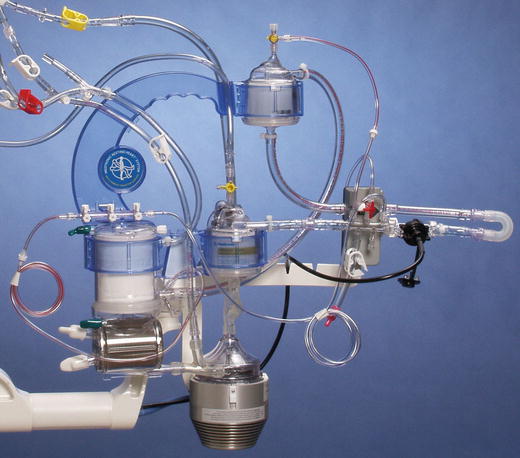
Fig. 33.4
Close-up image of the Resting Heart System showing the small reservoir in the center. The centrifugal pump is shown at the bottom. The rest are filters provided with alarm systems for air venting
33.1.9 Hemodynamics
As cardiopulmonary bypass is implemented, the patient’s blood pressure usually drops briefly as the blood is diverted from the heart to the heart–lung machine. This drop is precipitated by the cold (ambient) temperature of the fluid, that was used to prime the machine and which the heart–lung machine has now introduced into the patient’s aorta. It also results from the emptying of blood from the heart. This drop should last no more than a minute or two, i.e., before the proper pressure and flow is reestablished. The surgeon should not continue with the procedure unless s/he is confident that forward flow and drainage are optimized. In general, it is preferable to maintain a systemic pressure of approximately 70 mmHg and flows between 1500 and 2500 mL-m2 of body surface area throughout the entire surgical procedure (ideally 2.4 × body surface area). If the systemic pressure tends to sag, which can happen because of the various factors (e.g., loss of vascular tonus), the anesthesiologist and perfusionist must coordinate administration of vasoconstrictor agents (such as neosynepherine). If the pressure is too high, vasodilators are administered and/or the rate of perfusion is decreased to restore safe pressures.
Importantly, venous pressure and oxygen saturations should be monitored very carefully throughout any bypass procedure. An altered venous pressure is one of the most important indicators that a potential obstruction in the venous return has occurred, either at the level of the venous cannula or within the superior or inferior vena cava. Such obstructions will often lead to major procedural complications if they are not monitored and immediately corrected. Typically the perfusionist reports any concern to the surgeon so that s/he can check whether any obstruction may exist. During cardiopulmonary bypass, the venous pressure should usually be near zero and saturation above 70 % because all the blood is completely diverted into the heart–lung machine. Once the pressures are equilibrated, the temperatures must be maintained at the level of hypothermia that the surgeon has chosen.
Elevated central venous pressures and pulmonary artery pressures suggest poor drainage, as does a heart that is distending. Markers of end organ perfusion during bypass include: urine output, mixed venous saturation, lactic acid, and/or base deficit. Acidosis, low urine output, and decreased mixed venous saturation suggest poor oxygen delivery to the tissues.
< div class='tao-gold-member'>
Only gold members can continue reading. Log In or Register a > to continue
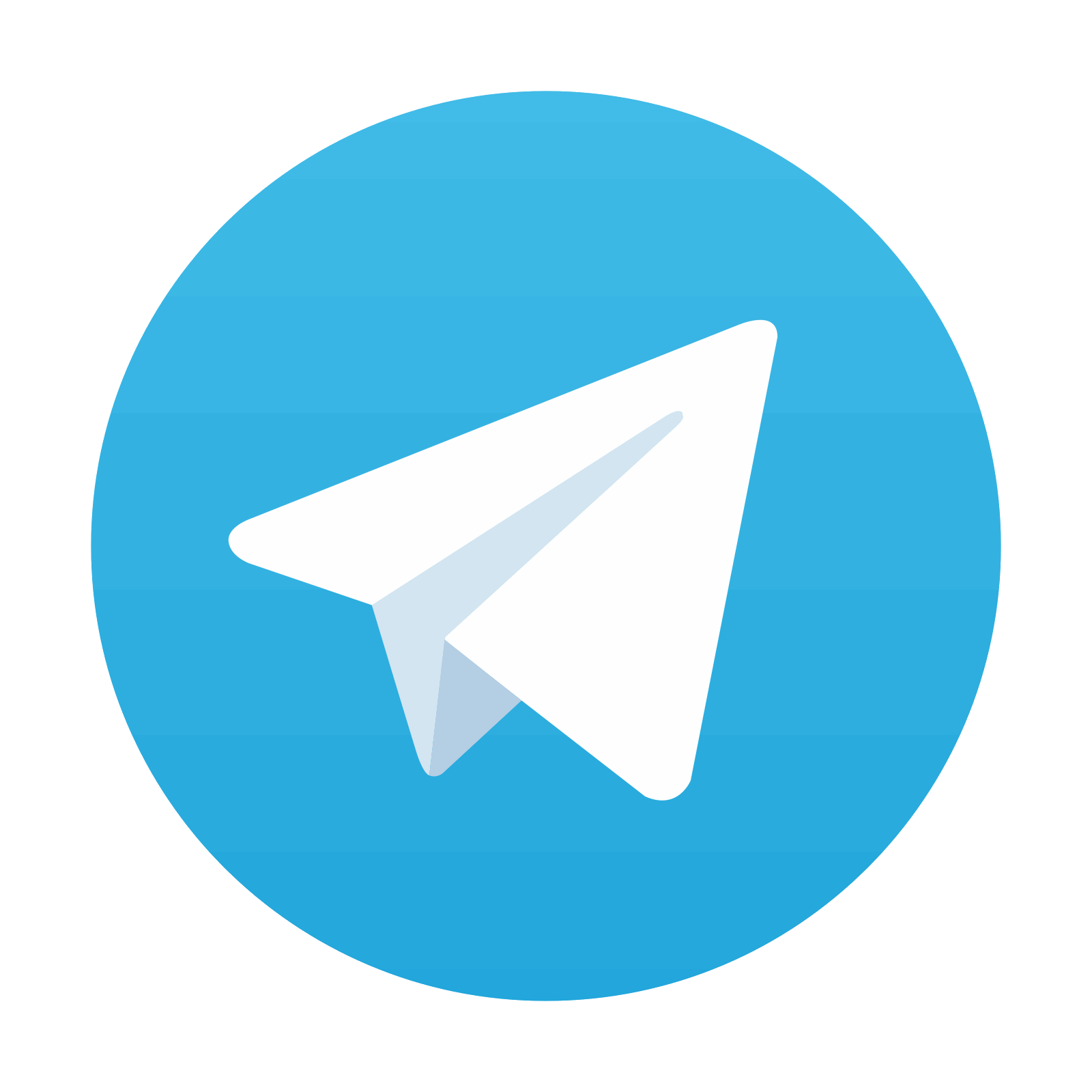
Stay updated, free articles. Join our Telegram channel

Full access? Get Clinical Tree
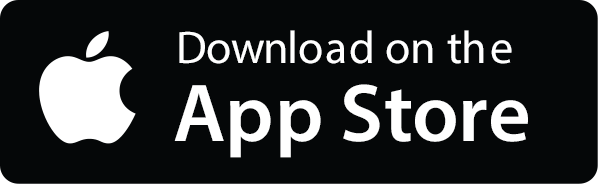
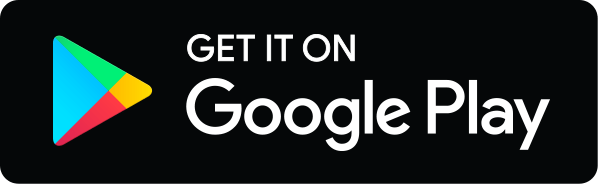