Fig. 3.1
The four major contributors to heart development illustrated in the chick model system: first heart field, second heart field, cardiac neural crest, and the proepicardial organ. (A) Day 1 chick embryo (equivalent to day 20 of human development). Red denotes first heart field cells and yellow denotes second heart field cells. (B) Day 2.5 chick embryo (equivalent to approximately 5 weeks of human development). Color code: green = cardiac neural crest cells; red = first heart field cells; yellow = second heart field cells; blue = proepicardial cells. (C) Day 8 chick heart (equivalent to approximately 9 weeks of human development). Color code: green = derivatives of the cardiac neural crest; yellow = derivatives of the second heart field; red = derivatives of the first heart field; blue = derivatives of the proepicardial organ. Ao aorta, APP anterior parasympathetic plexus, APS aorticopulmonary septum, BA branchial arch, Co coronary vessels, E eye, H heart, IFT inflow tract, IVS interventricular septum, LA left atrium, LV left ventricle, LVAB left ventral arterial branch of the Xth (vagal) cranial nerve, Mb midbrain, NF neural folds, OFT outflow tract, Otc otic placode, P pulmonary artery, RA right atrium, RDAB right dorsal arterial branch of the Xth (vagal) cranial nerve, RV right ventricle, SMC smooth muscles cells, T trunk
Table 3.1
Developmental timeline of human heart embryology
Human development (days) | Developmental process |
---|---|
0 | Fertilization |
1–4 | Cleavage and movement down the oviduct to the uterus |
5–12 | Implantation of the embryo into the uterus |
13–14 | Primitive streak formation (midstreak level contains precardiac cells) |
15–17 | Formation of the three primary germ layers (gastrulation): ectoderm, mesoderm, and endoderm; midlevel primitive streak cells that migrate to an anterior and lateral position form the bilateral first heart field and a more medially located the second heart field |
17–18 | Lateral plate mesoderm splits into the somatopleuric mesoderm and splanchnopleuric mesoderm; splanchnopleuric mesoderm contains the myocardial and endocardial cardiogenic precursors in the region of the first heart field and second heart field |
18–26 | Neurulation (formation of the neural tube) |
20 | Cephalocaudal and lateral folding brings the bilateral endocardial tubes into the ventral midline of the embryo |
21–22 | Heart tube fusion |
22 | Heart tube begins to beat |
22–28 | Heart looping and the accretion of cells from the first and second heart fields; proepicardial cells invest the outer layer of the heart tube and eventually form the epicardium and coronary vasculature; neural crest migration starts |
32–37 | Cardiac neural crest migrates through the aortic arches and enters the outflow tract of the heart |
57+ | Outflow tract and ventricular septation complete |
Birth | Functional septation of the atrial chambers, as well as the pulmonary and systemic circulatory systems |
3.2 First Heart Field Contribution to the Linear Heart Tube, Left Ventricle, and Atria
The cells that will become the heart are among the first cell lineages formed in the vertebrate embryo [23, 24]. By day 15 of human development, the primitive streak has formed [1] and the first mesodermal cells to migrate (gastrulate) through the primitive streak are also the cells fated to become myocytes or heart cells [25, 26] (Fig. 3.2). These mesodermal cells dedicated for heart development migrate to an anterior and lateral position where they initially form a bilateral FHF and a more medially located SHF [10, 11, 15, 16, 27] (Fig. 3.1A). Specifically, the posterior border of the bilateral FHF reaches down to the first somite in the lateral mesoderm on both sides of the midline [8, 28] (Fig. 3.1A). At day 18 of human development, the lateral plate mesoderm is split into two layers—somatopleuric and splanchnopleuric [1]. It is the splanchnopleuric mesoderm layer that contains the myocardial, smooth muscle, and endocardial cardiogenic precursors in the region of the FHF and SHF, as defined above. Presumptive endocardial cells delaminate from the splanchnopleuric mesoderm in the FHF and coalesce via vasculogenesis to form two lateral endocardial tubes [29]. During the third week of human development, two bilateral layers of myocardium surrounding the endocardial tubes are brought into the ventral midline during closure of the ventral foregut via cephalic and lateral folding of the embryo [1] (Fig. 3.2A). The lateral borders of the myocardial mesoderm layers are the first heart structures to fuse, followed by the fusion of the two endocardial tubes which then form one endocardial tube surrounded by splanchnopleuric-derived myocardium (Fig. 3.2B, C). The medial borders of the myocardial mesoderm layers are the last to fuse [30]. Thus, the early heart is continuous with splanchnopleuric mesoderm across the dorsal mesocardium (Fig. 3.2C). This will eventually partially break down to form the ventral aspect of the linear heart tube with a posterior inflow (venous pole) and anterior outflow (arterial pole), as well as the dorsal wall of the pericardial cavity [18, 30]. During the fusion of the endocardial tubes, the myocardium secretes an extracellular (acellular) matrix (enriched in chondroitin sulfate, versican, heparan sulfate, hyaluronic acid, hyaluronan, and proteoglycans), forming the cardiac jelly layer separating the myocardium and endocardium [31]. By day 22 of human development, the linear heart tube begins to beat. As the human heart begins to fold and loop from day 22 to day 28 (described below), epicardial cells from the PEO will invest the outer layer of the heart tube (Figs. 3.1B and 3.3A), resulting in a heart tube with four primary layers: endocardium, cardiac jelly, myocardium, and epicardium [1] (Fig. 3.3B).
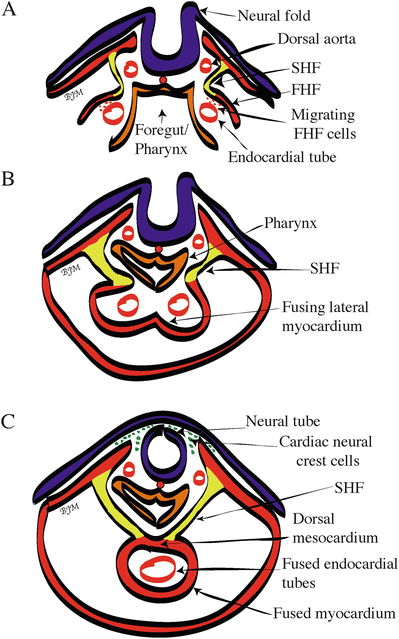
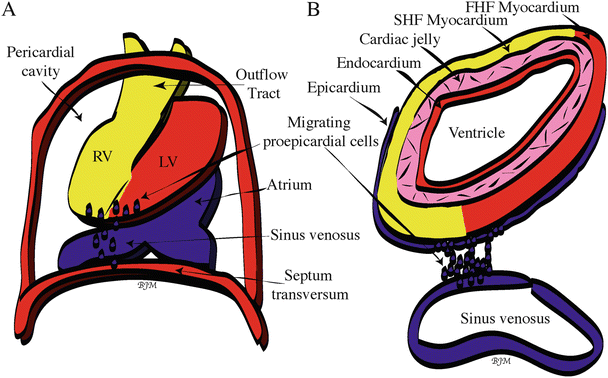
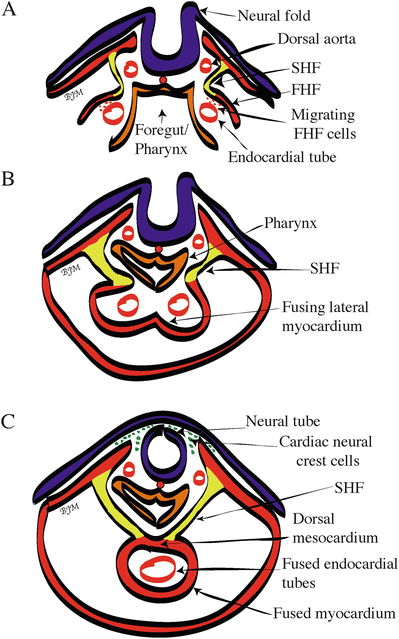
Fig. 3.2
Cross-sectional view of human heart tube fusion . (A) Day 20, cephalocaudal and lateral folding brings bilateral endocardial tubes into the ventral midline of the embryo. (B) Day 21, start of heart tube fusion. (C) Day 22, complete fusion, resulting in the beating primitive heart tube. Color code of the embryonic primary germ layer origin: blue/purple = ectoderm; red = mesoderm; orange = endoderm; yellow = second heart field. FHF first heart field, SHF second heart field
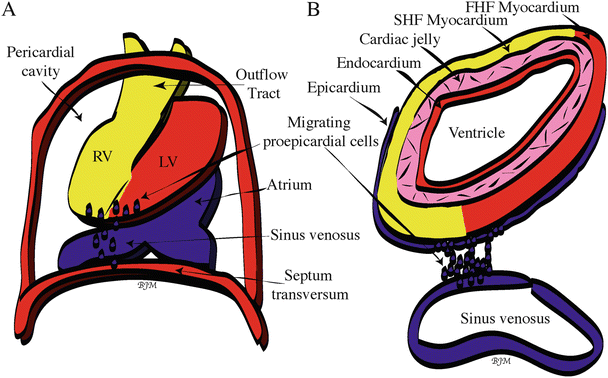
Fig. 3.3
Origin and migration of proepicardial cells . (A) Whole mount view of the looping human heart within the pericardial cavity at day 28. Proepicardial cells (blue dots) emigrate from the sinus venosus and possibly the septum transversum and then migrate out over the outer surface of the ventricles, eventually surrounding the entire heart. (B) Cross-sectional view of the looping heart showing the four layers of the heart: epicardium, myocardium, cardiac jelly, and endocardium. Color code: yellow = second heart field (SHF)-derived cells; red (within heart) = first heart field (FHF)-derived cells. LV left ventricle, RV right ventricle
3.3 Second Heart Field Contribution to the Outflow Tract, Right Ventricle, and Atria
A cascade of signals identifying the left and right sides of the embryo is thought to initiate the process of primary linear heart tube looping [32]. The primary heart tube loops to the right of the embryo and bends to allow convergence of the inflow (venous) and outflow (arterial) ends between day 22 and day 28 of human development (Fig. 3.4). This process occurs prior to the division of the heart tube into four chambers and is required for proper alignment and septation of the mature cardiac chambers. During the looping process, the primary heart tube increases dramatically in length (by four- to fivefold) on both the outflow and inflow poles via the addition of progenitor cells originating from the SHF (pharyngeal mesoderm) [7–18]. These multipotent progenitor cells within the developing heart give rise to myocardium, smooth muscle, and endothelial cells [12]. Previous experiments in the 1970s already revealed that the distal right ventricle and outflow tract (OFT) are added later to the looping heart by addition of cells lying outside the early heart [12, 33]. Researchers at that time, however, still assumed that the primary linear heart tube already contained all the cell lineages to build the adult heart. It was not until the rediscovery of these progenitor cells in 2001 (at the time termed anterior heart field or secondary heart field) that the clinical relevance of congenital heart defects was correlated to cells in this heart field—a big step in truly understanding heart development [7–9, 12]. The terms anterior heart field and secondary heart field are now considered to be a subpopulation of the SHF, a larger field of progenitor cells in pharyngeal mesoderm [12, 34]. The SHF is then contained within a larger field of multipotent cranial mesoderm (cardiocraniofacial field) that plays a critical role in development of both the arterial pole of the heart and craniofacial morphogenesis [12]. Specifically, the SHF (Figs. 3.1b and 3.2c) is located along the splanchnopleuric mesoderm (beneath the floor of the foregut) at the attachment site of the dorsal mesocardium [7–18]. During looping, the anterior SHF (previously termed anterior heart field or secondary heart field) cells undergo epithelial-to-myocardial transformation at the outflow (arterial) pole and add additional myocardial cells onto the then developing outflow tract, creating the great vessels (aorta and pulmonary trunk) and the right ventricle. This lengthening of the primary heart tube appears to be an important process for the proper alignment of the inflow and outflow tracts prior to septation. If this process does not occur normally, ventricular septal defects and malpositioning of the aorta may occur [30]. Recent evidence also indicates that the posterior SHF contributes to the inflow tract, creating parts of the left and right atria. Thus, the SHF contains two primary regions: (1) an anterior region or compartment that contributes to the outflow tract and (2) a posterior region or compartment that contributes to the inflow tract, as well as possibly the PEO [10, 15, 17, 35–37]. Defects in posterior SHF development result in conotruncal, atrial, and atrioventricular septal defects, major forms of congenital heart defects in humans [12].
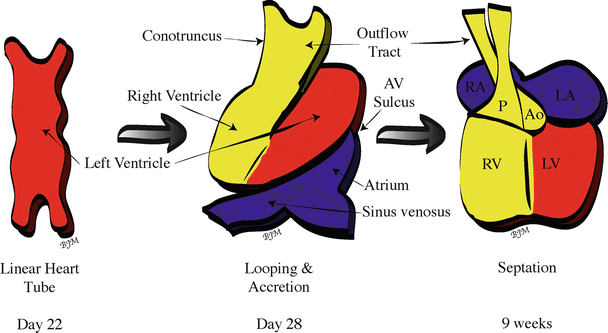
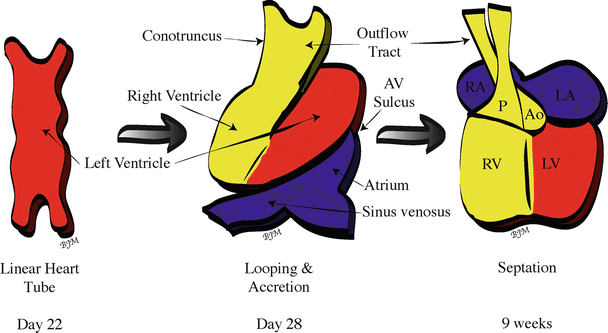
Fig. 3.4
Looping, accretion, and septation of the human primary linear heart tube . Blue (first heart field- and second heart field-derived cells) and yellow (second heart field-derived cells) regions represent tissue added during looping; red = first heart field-derived cells. Ao aorta, AV atrioventricular, LA left atrium, LV left ventricle, P pulmonary trunk, RA right atrium, RV right ventricle
By day 28 of human development, the chambers of the heart are in position and are demarcated by visible constrictions and expansions which denote the sinus venosus, common atrial chamber, atrioventricular sulcus, ventricular chamber, and conotruncus (proximal and distal outflow tract) [1, 30] (Fig. 3.4).
3.4 Cardiac Neural Crest Contribution and Septation of the Outflow Tract and Ventricles
Once the chambers are in the correct position after looping, extensive remodeling of the primitive vasculature and septation of the heart can occur. The CNC is an extracardiac population of cells (from outside of the first or SHFs) that arise from the neural tube in the region of the first three somites up to the midotic placode level (rhombomeres 6, 7, and 8) (Fig. 3.5) [2, 38, 39]. CNC cells leave the neural tube during weeks 3–4 of human development and then migrate through aortic arches 3, 4, and 6 (Fig. 3.1b) and eventually into the developing outflow tract of the heart (during weeks 5–6). These cells are necessary for complete septation of the outflow tract and ventricles (completed by week 8 of human development), as well as the formation of the anterior parasympathetic plexus which contributes to cardiac innervation and regulation of heart rate [1, 2, 20, 38–42]. Recent evidence shows that CNC cells migrate to the venous pole of the heart as well and that their role is in the development of the parasympathetic innervation, the leaflets of the atrioventricular valves, and possibly the cardiac conduction system [43–45]. The primitive vasculature of the heart is bilaterally symmetrical but, during weeks 4–8 of human development, there is remodeling of the inflow end of the heart so that all systemic blood flows into the future right atrium [1]. In addition, there is also extensive remodeling of the initially bilaterally symmetrical aortic arch arteries into the great arteries (septation of the aortic and pulmonary vessels) that is dependent on the presence of the CNC [30, 46]. The distal outflow tract (truncus) septates into the aorta and pulmonary trunk via the fusion of two streams or prongs of CNC that migrate into the distal outflow tract. In contrast, the proximal outflow tract septates by fusion of the endocardial cushions and eventually joins proximally with the atrioventricular endocardial cushion tissue and the ventricular septum [47, 48]. The endocardial cushions are formed by both atrioventricular canal and outflow tract endocardial cells that migrate into the cardiac jelly, forming bulges or cushions.
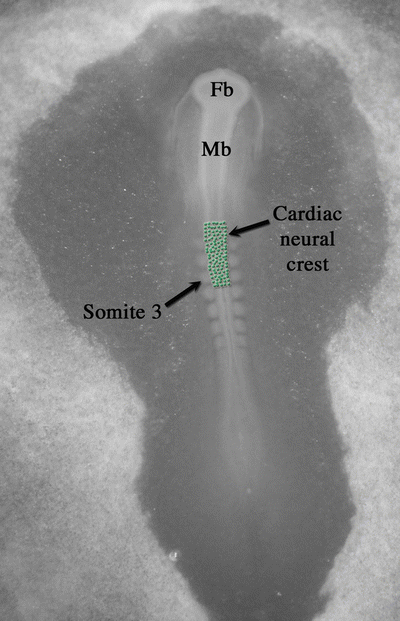
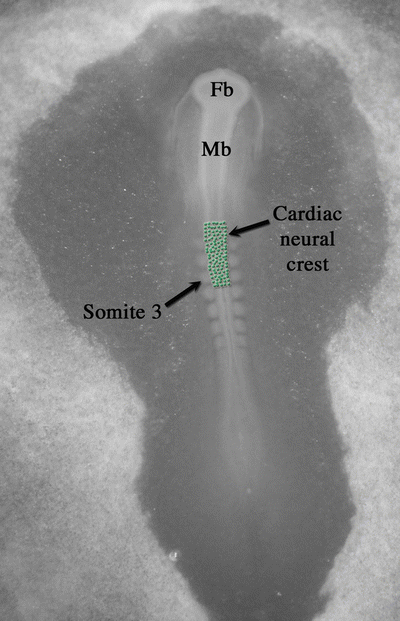
Fig. 3.5
Origin of the cardiac neural crest within a 34-h chick embryo. Green dots represent cardiac neural crest cells in the neural folds of hindbrain rhombomeres 6, 7, and 8 (the region of the first three somites up to the midotic placode level). Fb forebrain, Mb midbrain
Despite its clinical importance, to this date, almost nothing is known about the molecular pathways that determine cell lineages in the CNC or regulate outflow tract septation [30, 49, 50]. However, it is known that if the CNC is removed before it begins to migrate, conotruncal septa completely fail to develop, and blood leaves both the ventricles through what is termed a persistent truncus arteriosus , a rare congenital heart anomaly that can be seen in humans [20, 40]. Failure of outflow tract septation may also be responsible for other forms of congenital heart disease including transposition of the great vessels, high ventricular septal defects, and tetralogy of Fallot [1, 20, 38, 40]. Additional information on these congenital defects can be found in Chap. 10.
The septation of the outflow tract (conotruncus) is tightly coordinated with the septation of the ventricles and atria to produce a functional heart [1, 51, 52]. All of these septa eventually fuse with the atrioventricular (AV) cushions that also divide the left and right AV canals and serve as a source of cells for the AV valves. Prior to septation, the right atrioventricular canal and right ventricle expand to the right, causing a realignment of the atria and ventricles so that they are directly over each other. This allows venous blood entering from the sinus venosus to flow directly from the right atrium to the presumptive right ventricle without flowing through the presumptive left atrium and ventricle [1, 30]. The new alignment also simultaneously provides the left ventricle with a direct outflow path to the truncus arteriosus and subsequently to the aorta.
Between weeks 4 and 7 of human development, the left and right atria undergo extensive remodeling and are eventually septated. Yet, during the septation process, a right-to-left shunting of oxygenated blood (oxygenated by the placenta) is created via a series of shunts, ducts, and foramens (Fig. 3.6). Prior to birth, the use of the pulmonary system is not necessary, but eventually a complete separation of the systemic and pulmonary circulatory systems will be required for normal cardiac and systemic function [1]. Initially, the right sinus horn is incorporated into the right posterior wall of the primitive atrium, and the trunk of the pulmonary venous system is incorporated into the posterior wall of the left atrium via a process called intussusception . At day 26 of human development, a crescent-shaped wedge of tissue called the septum primum begins to extend into the atrium from the mesenchyme of the dorsal mesocardium. As it grows, the septum primum diminishes the ostium primum, a foramen allowing the shunting of blood from the right to left atrium. However, programmed cell death near the superior edge of the septum primum creates a new foramen, the ostium secundum, which continues the right-to-left shunting of oxygenated blood. An incomplete, ridged septum secundum with a foramen ovale near the floor of the right atrium forms next to the septum primum, both of which fuse with the septum intermedium of the AV cushions [1]. At the same time as atrial septation is beginning, about the end of the fourth week of human development, the muscular ventricular septum begins to grow toward the septum intermedium (created by the fusion of the atrioventricular cushions), creating a partial ventricular septum. By the end of the ninth week of human development, the outflow tract septum has grown down onto the upper ridge of this muscular ventricular septum and onto the inferior endocardial cushion, completely separating the right and left ventricular chambers.
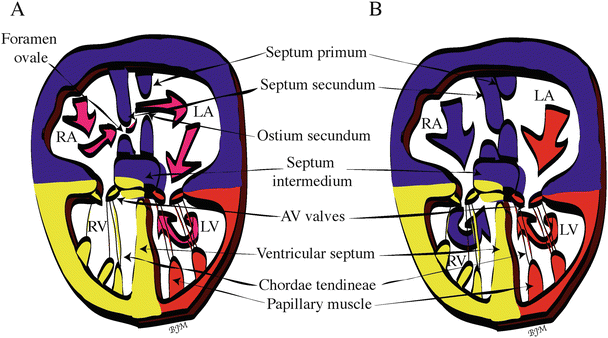
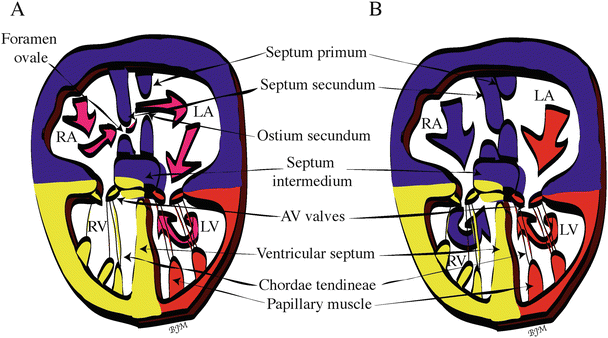
Fig. 3.6
Transition from fetal dependence on the placenta for oxygenated blood to self-oxygenation via the lungs. (A) Circulation in the fetal heart before birth. Pink arrows show right-to-left shunting of placentally oxygenated blood through the foramen ovale and ostium secundum. (B) Circulation in the infant heart after birth. The first breath of the infant and cessation of blood flow from the placenta cause final septation of the heart chambers (closure of the foramen ovale and ostium secundum) and thus separation of the pulmonary and systemic circulatory systems. Blue arrows show the pulmonary circulation and red arrows show the systemic circulation within the heart. Color code: Blue (first heart field- and second heart field-derived cells), red (first heart field-derived cells), and yellow (second heart field-derived cells). AV atrioventricular, LA left atrium, LV left ventricle, RA right atrium, RV right ventricle
It is not until after birth, however, that the heart is functionally septated within the atrial region. At birth, dramatic changes in the circulatory system occur due to the transition from fetal dependence on the placenta for oxygenated blood to self-oxygenation via the lungs. More specifically, during fetal life, only small amounts of blood (about 5 % of the cardiac output) are flowing through the pulmonary system because the fluid-filled lungs create high flow resistance, resulting in low-volume flow into the left atrium from the pulmonary veins. This allows the high-volume blood flow coming from the placenta to pass through the inferior vena cava into the right atrium, where it is then directed across the foramen ovale into the left atrium. The oxygenated blood then flows into the left ventricle and directly out to the body of the fetus via the aorta. At birth, the umbilical blood flow is interrupted, stopping the high-volume flow from the placenta. In addition, the alveoli and pulmonary vessels open when the infant takes its first breath, dropping the resistance in the lungs and allowing more flow into the left atrium from the lungs. This reverse in pressure difference between the atria pushes the flexible septum primum against the ridged septum secundum and closes off the foramen ovale and ostium secundum, resulting in the complete septation of the heart chambers [1] (Fig. 3.6). For more information on defects and repairs of the foramen ovale, see Chap. 37.
3.5 Proepicardial Organ and Coronary Artery Development
The last major contributor to vertebrate heart development discussed in this chapter is the PEO [15, 21, 22]. Prior to heart looping, the primary heart tube consists of endocardium, cardiac jelly, and myocardium. It is not until the start of heart looping that epicardial cells from the PEO surround the myocardium, forming the fourth layer of the primary heart tube called the epicardium [15, 53] (Fig. 3.3). This population of cells will eventually give rise to the coronary vasculature. A neural crest origin of the coronary vessels was originally hypothesized, but recent lineage tracing studies have shown that the neural crest gives rise to cells of the tunica media of the aortic and pulmonary trunks but not the coronary arteries [29, 54]. These experiments eventually showed that the coronary vasculature is derived from the PEO, a nest of cells in the dorsal mesocardium of the sinus venosus or septum transversum. These cells, which are derived from an independent population of splanchnopleuric mesoderm cells, migrate onto the primary heart tube (Fig. 3.3) between days 22 and 28 of human development, just as the heart initiates looping [1, 30]. Prior to migration, these cells are collectively called the PEO (or proepicardium). Interestingly, three lineages of the coronary vessel cells (smooth muscle, endothelial, and connective tissue cells) are segregated in the PEO prior to migration into the heart tube [29, 55]. These cells will coalesce to form coronary vessels de novo via the process of vasculogenesis [56]. Recently, it has also been shown that the epicardium provides a factor needed for normal myocardial development and is a source of cells forming the interstitial myocardium and cushion mesenchyme [30, 36]. It is considered that understanding the embryological origin of the vascular system and its molecular regulation may help to explain the varying susceptibility of different components of the vascular system to atherosclerosis [29, 57]. Recently, it has also been suggested that epicardium-derived cells may provide a source of cells for myocardial regeneration after a myocardial infarction [22]. Lastly, among the different stem cell populations identified in the later heart, Isl1-positive cells may be a population of resident cardiovascular stem cells derived from residual SHF cells [12, 58, 59]. Thus, approaches aimed at cardiac repair by manipulation of cardiac progenitor cells will depend on properly understanding how lineage choices are regulated in the SHF and PEO [12, 60].
< div class='tao-gold-member'>
Only gold members can continue reading. Log In or Register a > to continue
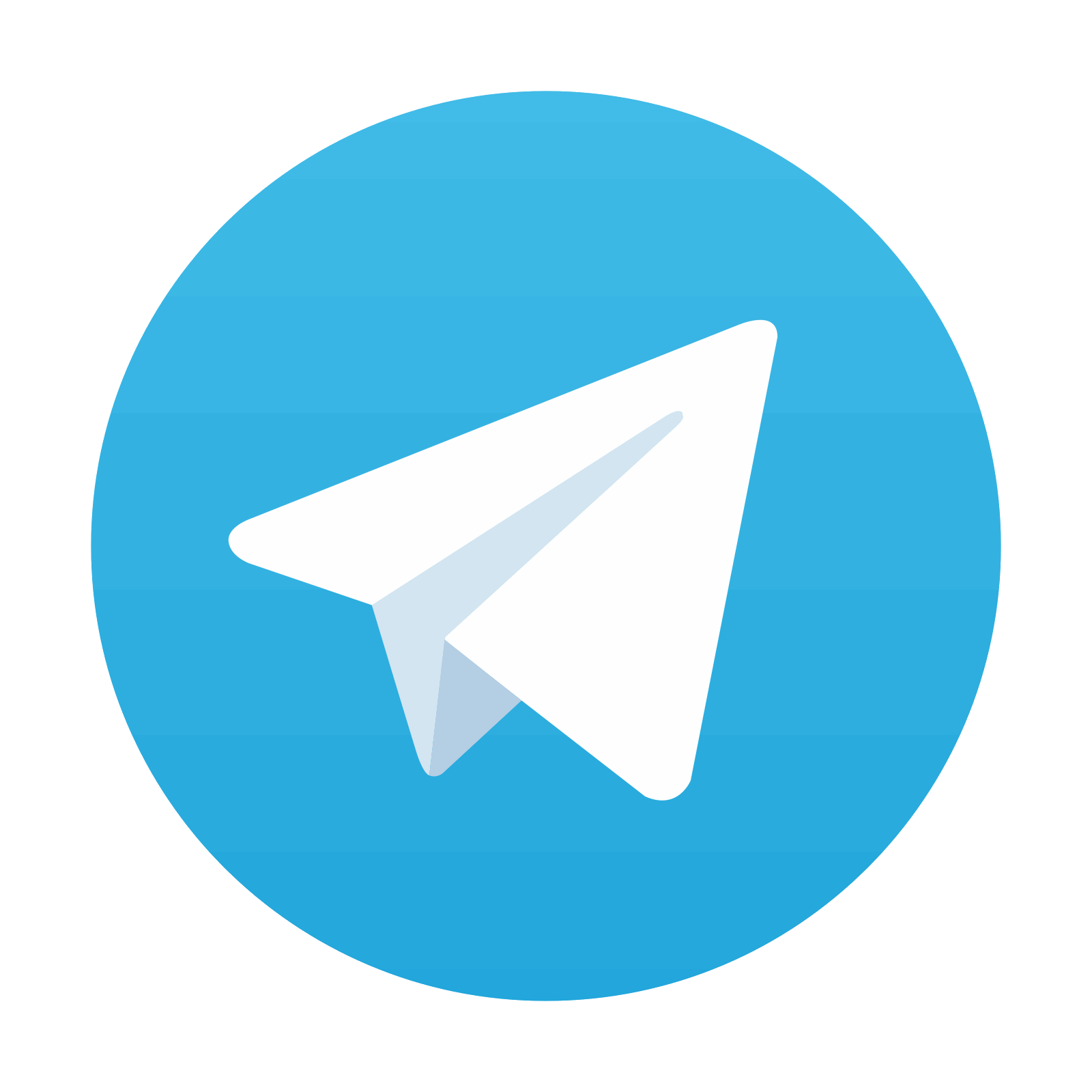
Stay updated, free articles. Join our Telegram channel

Full access? Get Clinical Tree
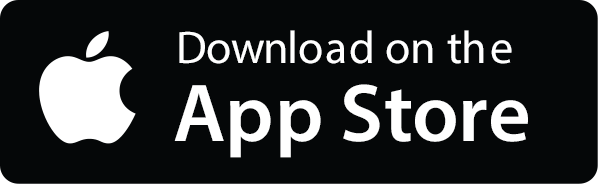
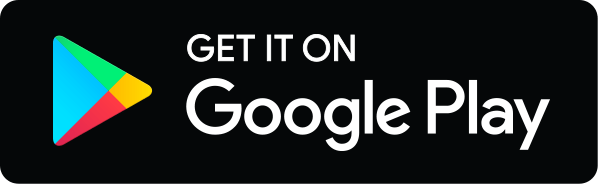