Candidacy for heart transplantation is influenced by the severity of pulmonary hypertension. In this study, invasive hemodynamics from right-sided cardiac catheterization were compared with values obtained by validated equations from Doppler 2-dimensional transthoracic echocardiography. This prospective study was conducted in 40 patients with end-stage heart failure evaluated for heart transplantation or ventricular assist device implantation. Transthoracic echocardiography and right-sided cardiac catheterization were performed within 4 hours. From continuous-wave Doppler of the tricuspid regurgitation jet, pulmonary artery systolic pressure was calculated as the peak gradient across the tricuspid valve plus right atrial pressure estimated from inferior vena cava filling. Mean pulmonary artery pressure was calculated as (0.61 × pulmonary artery systolic pressure) + 2. Pulmonary vascular resistance (PVR) was calculated as (tricuspid regurgitation velocity/right ventricular outflow tract time-velocity integral × 10) + 0.16. Pulmonary capillary wedge pressure was calculated as 1.91 + (1.24 × E/E′). Pearson’s correlation and Bland-Altman analysis of mean differences between echocardiographic and right-sided cardiac catheterization measurements were statistically significant for all hemodynamic parameters (pulmonary artery systolic pressure: r = 0.82, p <0.05, mean difference 3.1 mm Hg, 95% confidence interval [CI] −0.2 to 6.3; mean pulmonary artery pressure: r = 0.80, p <0.05, mean difference 2.5 mm Hg, 95% CI 0.3 to 4.6; PVR: r = 0.52, p <0.05, mean difference 0.8 Wood units, 95% CI 0.3 to 1.4; pulmonary capillary wedge pressure: r = 0.65, p <0.05, mean difference 2.2 mm Hg, 95% CI 0.1 to 4.3). Compared with right-sided cardiac catheterization, PVR by Doppler echocardiography identified all patients with PVR >4 Wood units (n = 4), 73% of patients with PVR <2 Wood units (n = 8), and 52% of patients with PVR from 2 to 4 Wood units (n = 10). In conclusion, echocardiographic estimation of cardiopulmonary hemodynamics is reliable in patients with end-stage cardiomyopathy. The noninvasive assessment of hemodynamics by echocardiography may be able to decrease the number of serial right-sided cardiac catheterizations in selected patients awaiting heart transplantation. However, in patients with borderline PVR, right-sided cardiac catheterization is indicated to assess eligibility for transplantation.
Noninvasive hemodynamic assessment obtained with Doppler echocardiography has been used for many years. Several equations to estimate pulmonary vascular hemodynamics have been derived from echocardiographic data. These have been correlated with invasive hemodynamics by right-sided cardiac catheterization and validated, mainly in patients with primary pulmonary artery hypertension and in those with chronic heart failure. Fewer studies have been done in patients with advanced heart failure. It is unclear if echocardiographic hemodynamics can be reliably applied to the pre–heart transplantation population to monitor pulmonary hypertension and pulmonary vascular resistance (PVR) and eliminate or reduce the frequency of right-sided cardiac catheterization. We conducted this study to compare the hemodynamics by right-sided cardiac catheterization (the gold standard) with the values obtained by the latest validated equations by Doppler 2-dimensional transthoracic echocardiography.
Methods
This prospective study was conducted in 40 patients with end-stage heart failure who were evaluated for heart transplantation or left ventricular assist device placement per current guidelines and presented for scheduled echocardiography and right-sided cardiac catheterization on the same day. The study was approved by the institutional review board at the University of Utah and the Veterans Affairs Medical Center, Salt Lake City, Utah. Patients with congenital heart disease, those with atrial fibrillation at the time of enrollment, and those who had undergone heart transplantation were excluded. Those aged >68 years, which is a selection criterion for heart transplantation at our center, were also excluded. After informed consent was obtained, noninvasive systemic blood pressure and heart rate were recorded. Then, patients underwent 2-dimensional transthoracic echocardiographic evaluation, followed by right-sided cardiac catheterization within an interval of 2 to 4 hours. Normal mean pulmonary artery pressure (mPAP) was defined as <25 mm Hg at rest. Mild pulmonary hypertension was defined as mPAP 26 to 35 mm Hg, moderate as 36 to 45 mm Hg, and severe as >45 mm Hg. Pulmonary capillary wedge pressure (PCWP) >15 mm Hg was considered abnormal. Per the updated classification, pulmonary hypertension attributable to left-sided cardiac pathology was categorized as pulmonary hypertension with normal or near normal PVR <3 Wood units and normal transpulmonary gradient (defined as mPAP − PCWP) <12 mm Hg and pulmonary arterial hypertension as pulmonary hypertension with elevated PVR or elevated transpulmonary gradient.
All measurements were performed by a single echocardiographer who was blinded to the right-sided cardiac catheterization values. Studies were done using a GE Vivid ultrasound system (GE Medical Systems, Milwaukee, Wisconsin). By continuous-wave Doppler, tricuspid regurgitation (TR) velocity was recorded from different views to measure the peak velocity. It was measurable in all except 1 patient. TR was graded semiquantitatively as grades 1 to 4. Pulmonary artery systolic pressure was calculated from the peak velocity gradient across the tricuspid valve, and pulmonary artery diastolic pressure (PADP) was calculated from the gradient in the TR jet at the time of pulmonary valve opening using the modified Bernoulli equation. Unlike in previous studies, we added right atrial pressure estimated from inferior vena cava size and respiratory dynamics to the 2 gradients to obtain pulmonary artery systolic pressure and PADP. Images of the inferior vena cava were obtained from the subcostal view, and the right atrial pressure was estimated from the inferior vena cava on the basis of the size and collapsibility with sniff test (>50%) as normal size and collapsible (5 mm Hg), dilated >2 cm and collapsible (10 mm Hg), dilated >2 cm and <50% collapsible (15 mm Hg), and dilated >2 cm and noncollapsible (20 mm Hg). The mPAP was calculated by using the formula mPAP 1 = (0.61 × pulmonary artery systolic pressure) + 2, which was validated and found to be the most accurate compared with 4 other formulas by Chemla et al in patients with pulmonary hypertension. It was also calculated as mPAP 2 = (2 × PADP + pulmonary artery systolic pressure)/3. In the parasternal short-axis view, the right ventricular outflow tract time-velocity integral was obtained by pulsed-wave Doppler. PVR 1 was calculated from peak TR velocity and right ventricular outflow tract time-velocity integral as PVR 1 = (TR velocity/right ventricular outflow tract time-velocity integral) × 10 + 0.16. PVR 2 was calculated using the formula (mPAP − PCWP)/cardiac output.
From the parasternal long-axis view, the left ventricular end-diastolic and end-systolic dimensions and left atrial anteroposterior dimension were recorded. From the apical 4-chamber view, end-diastolic and end-systolic volumes were measured to calculate the left ventricular ejection fraction using Simpson’s method. Cardiac output was calculated as heart rate × stroke volume (end-diastolic volume − end-systolic volume). In this view, mitral and tricuspid inflow velocities were recorded to measure early (E) and late (A) diastolic flow velocities and E-wave deceleration time. By pulsed-wave tissue Doppler imaging, medial mitral annular relaxation velocity (E′) was obtained. PCWP was calculated as 1.91 + (1.24 × E/E′). Mitral regurgitation was graded semiquantitatively as grades 1 to 4. In addition to right ventricular outflow tract time-velocity integral, right ventricular function was assessed by the lateral tricuspid annular peak systolic velocity using tissue Doppler imaging.
A Swan-Ganz catheter was introduced via the internal jugular or femoral vein under fluoroscopic guidance for hemodynamic measurements. The pressures in the right atrium, right ventricle, and pulmonary artery (pulmonary artery systolic pressure, PADP, and mPAP) as well as PCWP were recorded, and measurements were standardized to respiratory phase in end-expiration. Cardiac output was calculated using the Fick principle (with an assumed oxygen consumption), and PVR was calculated as (mean PAP − PCWP)/cardiac output. The 2 physicians performing, interpreting, and reporting the right-sided cardiac catheterization hemodynamics were blinded to the measurements on echocardiography.
Continuous variables are presented as mean ± SD and dichotomous data as numbers and percentages. Correlations between echocardiographic and right-sided cardiac catheterization measurements were done using Pearson’s bivariate correlation. Linear regression was used to generate the graphs showing the correlations. A 2-tailed p value ≤0.05 was considered statistically significant. Bland-Altman analysis was used to obtain the mean differences between echocardiographic and right-sided cardiac catheterization measurements.
Results
Forty patients were enrolled in the study, and 37 patients were included in the analysis. One patient was excluded from analysis because right-sided cardiac catheterization was not performed because of difficulties with venous access, and adequate echocardiographic windows to determine TR were not obtained. A second patient was excluded for congenitally corrected transposition of the great arteries. The third patient was excluded for mechanical mitral prosthesis. One patient had no significant TR, 27 patients had grade 1 or 2 TR, and 9 patients had grade 3 or 4 TR. All patients were in sinus rhythm with normal heart rates, except for 2 patients with sinus tachycardia with rates of 112 and 116 beats/min at the time of imaging. From the study cohort of 37 patients, 6 patients were considered for left ventricular assist device implantation, and the remaining 31 patients were evaluated for heart transplantation. The baseline clinical characteristics of the study patients are listed in Table 1 . Of the 37 patients, 20 had cardiac resynchronization therapy plus implantable cardioverter-defibrillators, and 10 patients had only implantable cardioverter-defibrillators. Pearson’s correlation and Bland-Altman analysis of the mean differences between echocardiographic and right-sided cardiac catheterization measurements are listed in Table 2 .
Characteristic | Value |
---|---|
Age (years) | 55 ± 10 |
Men | 30 (81%) |
Cause of cardiomyopathy (ischemic/nonischemic) | 20 (54%)/17 (46%) |
Hypertension | 19 (51%) |
Diabetes mellitus | 11 (30%) |
Sleep apnea | 9 (24%) |
Serum creatinine (mg/dl) | 1.2 ± 0.3 |
Cardiac resynchronization therapy/implantable cardioverter-defibrillator only | 20 (54%)/10 (27%) |
New York Heart Association functional class (III/IV) | 27 (73%)/10 (27%) |
Mean blood pressure (mm Hg) | 80 ± 11 |
Heart rate (beats/min) | 81 ± 15 |
Medications | |
β blockers | 36 (97%) |
Angiotensin-converting enzyme inhibitors/angiotensin receptor blockers | 30 (81%) |
Diuretics | 35 (95%) |
Digoxin | 13 (35%) |
Aldosterone receptor blockers | 24 (65%) |
Echocardiographic parameters | |
LV end-diastolic dimension (cm) | 6.7 ± 1.2 |
LV end-systolic dimension (cm) | 5.9 ± 1.3 |
LV fractional shortening (%) | 12 ± 7 |
LV ejection fraction (%) | 24 ± 8 |
Left atrial anteroposterior dimension (cm) | 4.5 ± 0.9 |
Right ventricular end-diastolic dimension (cm) | 4.3 ± 0.8 |
Mitral regurgitation (grades 1/2/3/4) | 20/7/4/3 |
TR (grades 1/2/3/4) | 14/12/9/1 |
TR velocity (m/s) | 2.7 ± 0.7 |
Tricuspid lateral annular peak systolic velocity (cm/s) | 9 ± 2 |
Right ventricular outflow tract time-velocity integral (cm) | 13 ± 5 |
E | 0.8 ± 0.2 |
E′(m) | 0.05 ± 0.01 |
E/E′ | 16 ± 5 |
E-wave deceleration time (ms) | 161 ± 55 |
Hemodynamic parameter | Echocardiography | Right-Sided Cardiac Catheterization | r | p Value | Bland-Altman Mean Difference (95% Confidence Interval) |
---|---|---|---|---|---|
Right atrial pressure (mm Hg) | 10 ± 6 | 9 ± 6 | 0.69 | <0.001 | 0.94 (−0.67 to 2.55) |
PASP (mm Hg) | 43 ± 16 | 46 ± 15 | 0.82 | <0.0001 | 3.08 (−0.16 to 6.33) |
Pulmonary artery diastolic pressure (mm Hg) | 21 ± 9 | 20 ± 8 | 0.65 | 0.0002 | 0.94 (−1.55 to 3.42) |
mPAP 1 (mm Hg) | 28 ± 10 | 31 ± 10 | 0.80 | <0.0001 | 2.48 (0.34 to 4.61) |
mPAP 2 (mm Hg) | 28 ± 10 | 31 ± 10 | 0.75 | <0.0001 | 2.50 (0.08 to 4.92) |
PCWP (mm Hg) | 22 ± 6 | 19 ± 8 | 0.65 | <0.0001 | 2.20 (0.10 to 4.30) |
Transpulmonary gradient (mm Hg) | 9 ± 7 | 12 ± 6 | 0.72 | <0.001 | 2.56 (0.83 to 4.29) |
PVR 1 (Wood units) | 2.6 ± 1.0 | 3.4 ± 1.9 | 0.52 | 0.001 | 0.82 (0.27 to 1.37) |
PVR 2 (Wood units) | 2.4 ± 2.2 | 3.4 ± 1.9 | 0.54 | <0.001 | 0.91 (0.22 to 1.61) |
Cardiac output (L/min) | 4.2 ± 1.5 | 3.8 ± 1.1 | 0.62 | 0.0001 | 0.47 (−0.02 to 0.96) |
Cardiac index (L/min/m 2 ) | 2.1 ± 0.7 | 1.9 ± 0.5 | 0.50 | 0.002 | 0.19 (−0.03 to 0.42) |
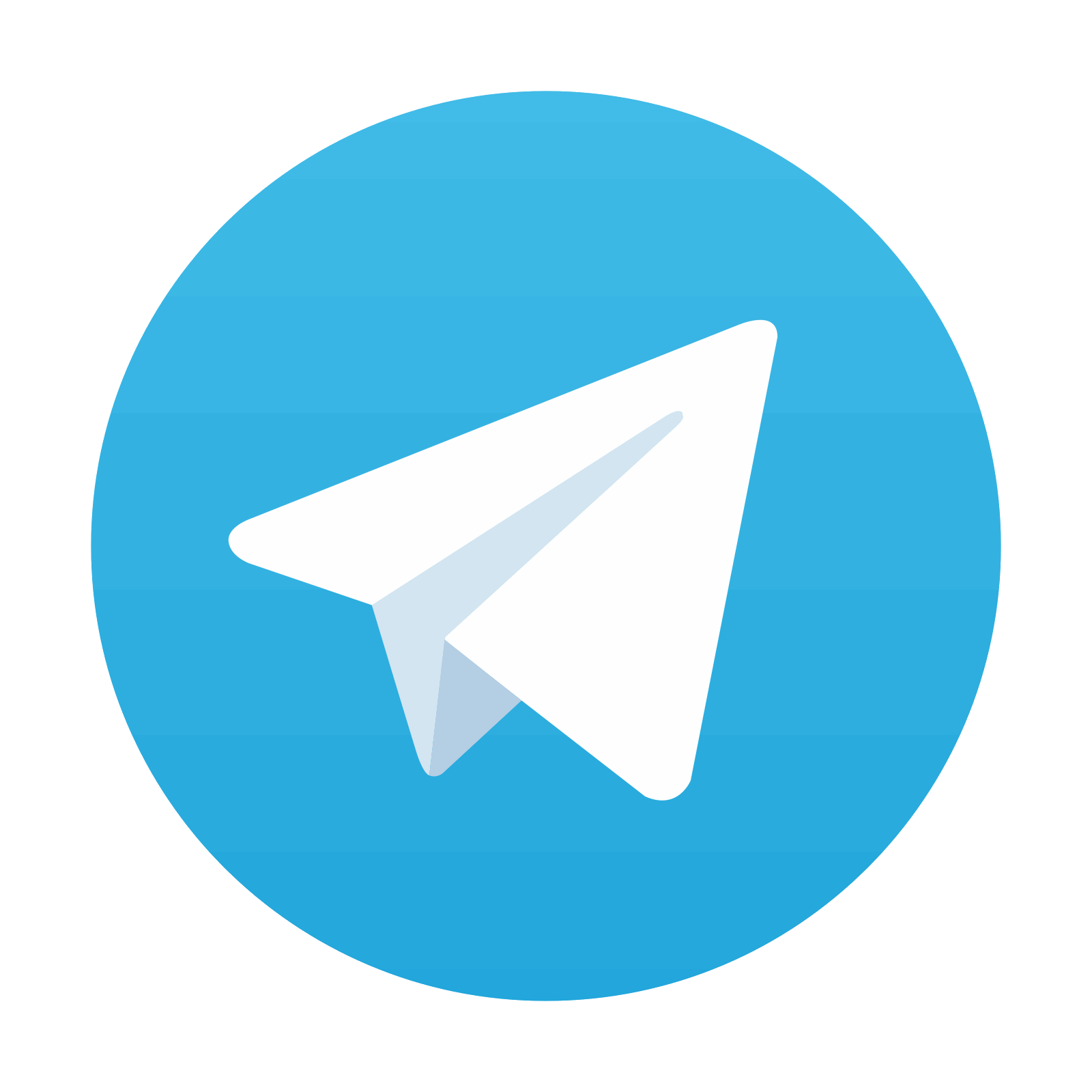
Stay updated, free articles. Join our Telegram channel

Full access? Get Clinical Tree
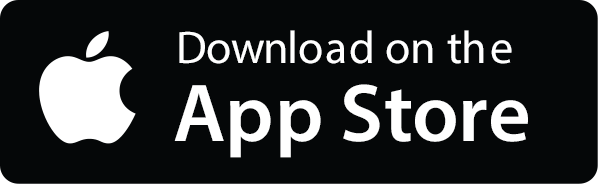
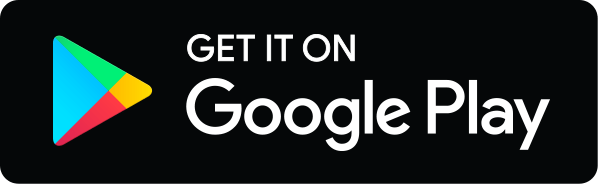
