Chapter 55 Biliary System
Anatomy And Physiology
Biliary anatomy is extremely variable, and precise knowledge of the normal and anatomic variants is critical to surgical intervention in the biliary tree. The distal common bile duct (CBD) inserts into the duodenum via the ampulla of Vater, passing through the sphincter of Oddi. Ascending from the duodenum, the common bile duct may join with the pancreatic duct in the wall of the duodenum, within the pancreas prior to insertion into the duodenal wall, or may enter the duodenum separately from the pancreatic duct (Fig. 55-1). The most inferior portion of the CBD is encompassed by the head of the pancreas. Superior to this portion, the common bile duct is divided into retroduodenal and supraduodenal segments. The insertion of the cystic duct marks the differentiation of the common hepatic duct above and the common bile duct below.
The cystic duct drains the gallbladder, which is divided into the neck, infundibulum with Hartmann’s pouch, body, and fundus of the gallbladder. Roughly the size and shape of a common light bulb, the gallbladder holds 30 to 60 mL of bile as an extrahepatic reservoir. The gallbladder is attached to the inferior surface of the liver and is enveloped by liver for a variable portion of its circumference. Although some gallbladders are almost enveloped by liver parenchyma, others hang on a mesentery, predisposing to volvulus. The attachment of the gallbladder to the liver, known as the gallbladder fossa, identifies the separation of the left and right lobes of the liver (Fig. 55-2). Where the gallbladder attaches to the liver, Glisson’s capsule does not form, and this common surface provides the venous and lymphatic drainage of the gallbladder. The cystic duct drains at an acute angle into the common bile duct and can range from 1 to 5 cm in length. There are a number of anatomic variations in insertion of the cystic duct, including into the right hepatic duct (Fig. 55-3). Within the neck of the gallbladder and cystic ducts lie folds of mucosa oriented in a spiral pattern, known as the spiral valves of Heister, which act to keep gallstones from entering the common bile duct, in spite of distention and intraluminal pressure. The dependent portion of Hartmann’s pouch may overlie the common hepatic or right hepatic ducts, thus placing these structures at risk during the performance of a laparoscopic cholecystectomy.
Vascular Anatomy
The segmental anatomy of the liver parenchyma is based on the vascular supply and drainage, and the biliary drainage is described by the corresponding vascular segment. The hepatic parenchyma is divided into lobes, each of which is divided into lobar segments (Fig. 55-4) to define the basic hepatic anatomic resections. The left lobe is comprised of medial and lateral segments. The right lobe is divided into posterior and anterior segments. Alternatively, the hepatic parenchyma can be divided into segments based on the specific hepatic venous drainage and portal inflow, allowing for a more precise description of anatomic pathology. In this classification system, as developed by Couinard,1 the liver is composed of eight segments. Segment I refers to the caudate lobe. The left lobe of the liver, supplied by the left portal vein, constitutes segments II through IV. The left lobe is further subdivided by the falciform ligament, which separates segments II and III, also known as the left lateral segment, from segment IV. Within the left lateral segment, segment II lies superior to the insertion of the portal vein and segment III lies inferior to it. Segment IV is similarly divided into segments IVA, above, and segment IVB, below the portal vein insertion. The right portal vein supplies the right lobe of the liver and divides into the posterior and anterior sector. Each sector is then subdivided based on its relative location compared with the portal vein. Segment V is supplied by the inferior branch of the anterior sector and segment VIII is supplied by the superior branch. In the posterior sector, segment VI is supplied by the inferior branch and segment VII is supplied by the superior branch. There are three major hepatic veins that drain into the inferior vena cava, in addition to a number of small veins that drain directly from the right lobe. The right hepatic vein constitutes most of the venous drainage from the right lobe and generally lies in the intersegmental fissure between the anterior and posterior sectors of the right lobe. The middle hepatic vein drains the medial segment of the left lobe and a small amount of the medial portions of segments V and VIII. In most cases, the middle hepatic vein fuses with the left hepatic vein that drains the left lateral segment.
Normally, the cystic artery arises from the right hepatic artery, which can pass posterior or anterior to the common bile duct to supply the gallbladder. Similar to the variability of the cystic duct, the cystic artery may arise from the right hepatic, left hepatic, proper hepatic, common hepatic, gastroduodenal, or superior mesenteric artery. Although variable, the cystic artery generally lies superior to the cystic duct and is usually associated with a lymph node, known as Calot’s node (Fig. 55-5). Because this node provides some of the lymphatic drainage of the gallbladder, it can be enlarged in the setting of gallbladder pathology, whether inflammatory or neoplastic.
Both within the liver and immediately outside the parenchyma, the bile ducts generally lie superior to the corresponding portal veins, which in turn are superior to the arterial supply (Fig. 55-6). Retaining a longer extrahepatic segment before inserting into the liver, the left hepatic duct travels under the edge of segment IV before slipping superior and posterior to the left portal vein. During this transverse portion, it can receive a few subsegmental branches from segment IV. The left duct drains segments II, III, and IV, with the most distal branch draining segment IVa. Further superolateral, the ducts draining segment IVb arise, and further up the left duct are the ducts for segments II and III. These fused ducts can generally be found just posterior and lateral to the umbilical recess. The caudate lobe drains via smaller ducts that enter the right and left hepatic duct systems. The drainage of the right duct system includes segments V, VI, VII, and VIII and is substantially shorter than the left duct, bifurcating almost immediately. The fusion of two sectoral ducts, posterior and anterior, creates this short right hepatic duct. The anterior sectoral duct runs in a vertical direction to drain segments V and VIII, whereas the posterior sectoral duct follows a horizontal course to drain segments VI and VII.
Physiology
Bile salts, such as cholic acid and deoxycholic acid, are originally created from cholesterol and secreted into bile canaliculi as cholic acid and its metabolite, deoxycholic acid. The liver actually makes only a small amount of the total bile salt pool used on a daily basis, because most bile salts are recycled after use in the intestinal lumen (Fig. 55-7). After passage into the intestinal tract and reabsorption by the terminal ileum, bile acids are transported back to the liver for recycling bound to albumin. Less than 5% of bile salts are lost each day in the stool. When sufficient quantities of bile salts reach the colonic lumen, the powerful detergent activity of the bile salts can cause inflammation and diarrhea.
The distal portion of the bile duct passes through the sphincter of Oddi (Fig. 55-8). The musculature of this sphincter is independent from that of the duodenal intestinal wall and responds differently to neurohumoral controls. This muscular sphincter, which normally maintains high tonic and phasic activity, is inhibited by CCK. With CCK-induced relaxation of the sphincter, bile flows more readily from the biliary tree. Coordinated with gallbladder contraction, the relaxation of this sphincter allows for evacuation of up to 70% of the gallbladder contents within 2 hours of CCK secretion. During the fasting state, the oblique passage of the bile duct through the duodenal wall and the tonic activity of the sphincter prevent duodenal contents from refluxing into the biliary tree.
General Considerations In Biliary Tree Pathophysiology
Imaging Studies
Ultrasound
Transabdominal ultrasound is a sensitive, inexpensive, reliable, and reproducible test to evaluate most of the biliary tree, being able to separate patients with medical jaundice from those with surgical jaundice. Therefore, this modality is seen as the study of choice for the initial evaluation of jaundice or symptoms of biliary disease. The finding of a dilated common bile duct in the setting of jaundice suggests an obstruction of the duct from stones, usually associated with pain, or from a tumor, which is commonly painless (Fig. 55-9). Gallbladder diseases are regularly diagnosed by ultrasound, because its superficial location with no overlying bowel gas enables its evaluation by sound waves. Ultrasound has a high specificity and sensitivity for cholelithiasis, or gallstones. The density of gallstones allows crisp reverberation of the sound wave, showing an echogenic focus with a characteristic shadowing behind the stone (Fig. 55-10). Most gallstones, unless impacted, will move with positional changes in the patient. This feature allows their differentiation from gallbladder polyps, which are fixed, and from sludge which will move more slowly and does not have the sharp echogenic pattern of gallstones. Pathologic changes seen in many gallbladder diseases can be identified by ultrasound. For example, the gallbladder wall thickening and pericholecystic fluid seen in cholecystitis are visible by ultrasound (Fig. 55-11). Porcelain gallbladder, with its calcified wall, will appear as a curvilinear echogenic focus along the entire gallbladder wall, with posterior shadowing (Fig. 55-12). In addition to division of medical versus surgical jaundice, ultrasound can sometimes identify the cause of obstructive jaundice, showing common bile duct stones or even cholangiocarcinoma.
Hepatic Iminodiacetic Acid Scan
Although incapable of providing precise anatomic delineation of pathophysiology, biliary scintigraphy, also known as a hepatic iminodiacetic acid scan (HIDA) scan, can be used to evaluate the physiologic secretion of bile. The injection of an iminodiacetic acid, which is processed in the liver and secreted with bile, allows identification of bile flow. Therefore, the failure to fill the gallbladder 2 hours after injection demonstrates obstruction of the cystic duct, as seen in acute cholecystitis (Figs. 55-13 and 55-14). In addition, the scan will identify obstruction of the biliary tree and bile leaks, which may be useful in the postoperative setting. HIDA scans can also be used to determine gallbladder function, because the injection of CCK during a scan will document physiologic ejection of the gallbladder. This may be useful in patients with biliary tract pain but without stones, because some patients have pain from impaired emptying, known as biliary dyskinesia. As a nuclear medicine test, the test demonstrates physiologic flow, but does not provide fine anatomic detail, nor can it identify gallstones.
Computed Tomography
Although ultrasound is clearly the first test of choice for delineation of biliary pathology, computed tomography (CT) provides superior anatomic information. Because most gallstones are radiographically isodense to bile, many will be indistinguishable from bile. However, because ultrasound is operator-dependent and provides no anatomic reconstruction of the biliary tree, CT can be used to identify the cause and site of biliary obstruction (Fig. 55-15). When performed for the evaluation of hepatic or pancreatic parenchyma or possible neoplastic processes, CT is invaluable in preoperative planning, and the use of arterial phase, portal venous phase, and delayed phase imaging, known as a triple-phase CT, has essentially replaced diagnostic angiography of the liver.
Magnetic Resonance Imaging and Magnetic Resonance Cholangiopancreatography
Magnetic resonance imaging (MRI) uses the water in bile to delineate the biliary tree and thus provides superior anatomic definition of the intrahepatic and extrahepatic biliary tree and pancreas. Although management of most patients with biliary pathology does not require the fine detail of anatomic evaluation shown by cross-sectional imaging, MRI is noninvasive, requires no radiation exposure, and can prove extremely useful when planning resection of biliary or pancreatic neoplasms or management of complex biliary pathology. By using the water content of bile, a cholangiopancreatogram can be created (Fig. 55-16).
Endoscopic Retrograde Cholangiopancreatography
Endoscopic retrograde cholangiopancreatography (ERCP) is an invasive test using endoscopy and fluoroscopy to inject contrast through the ampulla and image the biliary tree (Fig. 55-17). Although it does carry a complication rate of up to 10%, its usefulness lies in its ability to diagnose and treat many diseases of the biliary tree. For patients with malignant obstruction, ERCP can be used to provide tissue samples for diagnosis while also decompressing an obstruction, but does not stage patients accurately. Many benign diseases, such as choledocholithiasis, can be easily treated by endoscopic means. ERCP has also proven extremely useful in the diagnosis and treatment of complications of biliary surgery.
Intraoperative Cholangiography
Another imaging tool for the diagnosis of biliary tract abnormalities is the use of intraoperative cholangiography. With the injection catheter inserted via the cystic duct during a cholecystectomy or through another point in the biliary tree, intraoperative cholangiography can help delineate anomalous biliary anatomy, identify choledocholithiasis, or guide biliary reconstruction. Some surgeons advocate routine cholangiography during cholecystectomy. Advocates for routine cholangiography note that common duct injuries are less frequent when cholangiography is used routinely. However, because it adds operative time and fluoroscopic exposure to the operation, many surgeons use intraoperative cholangiography selectively during the performance of a cholecystectomy. Indications for the selective use of cholangiography include pain at the time of operative intervention, abnormal hepatic function panel, anomalous or confusing biliary anatomy, or inability to perform ERCP following cholecystectomy, dilated biliary tree, or any preoperative suspicion of choledocholithiasis (Box 55-1).
Endoscopic Ultrasound
Although of limited use in the evaluation of gallbladder pathology or intrahepatic disease of the biliary tree, endoscopic ultrasound (EUS) is valuable in the assessment of distal common bile duct and ampulla. With the close apposition of the distal common bile duct and pancreas to the duodenum, sound waves generated by EUS provide detailed evaluation of the bile duct and ampulla and have proven most useful in assessing tumors for invasion into vascular structures. Echoendoscopes are subdivided into those that scan perpendicular to the long axis of the endoscope, known as radial echoendoscopes, and those that scan parallel, known as linear echoendoscopes. Radial echoendoscopes are most useful for providing a tomographic evaluation (Fig. 55-18), whereas linear echoendoscopes can guide interventions such as needle biopsies under real-time ultrasound guidance (Fig. 55-19).
Benign Biliary Disease
Calculous Biliary Disease
Gallstones can be subclassified into two major subtypes, depending on the principle solute that precipitates into a stone. More than 70% of gallstones in America are formed by precipitation of cholesterol and calcium, with pure cholesterol stones accounting for only a small (<10%) portion. Pigment stones, further subclassified as black or brown stones, are caused by precipitation of concentrated bile pigments, the breakdown products of hemoglobin. Four major factors explain most gallstone formation—supersaturation of secreted bile, concentration of bile in the gallbladder, crystal nucleation, and gallbladder dysmotility. High concentrations of cholesterol and lipid in bile secretion from the liver constitute one predisposing condition to cholesterol stone formation, whereas increased hemoglobin processing is seen in most patients with pigment stones. Once in the gallbladder, bile is concentrated further through the absorption of water and NaCl, increasing the concentrations of the bile solutes and calcium. With respect to cholesterol stones (Fig. 55-20), cholesterol precipitates out into crystals when the concentration in vesicles exceeds the solubility of cholesterol (Fig. 55-21).2 This process of crystal formation is further accelerated by pronucleating agents, including glycoproteins and immunoglobulins. Finally, abnormal gallbladder motility can increase stasis in the gallbladder, allowing more time for solutes to precipitate in the gallbladder. Therefore, increased stone formation can be seen in conditions associated with impaired gallbladder emptying, such as prolonged fasting states, use of total parenteral nutrition, postvagotomy, and use of somatostatin analogues.
Chronic Cholecystitis
Diagnosis
The diagnosis of symptomatic cholelithiasis, the clinical manifestation of chronic cholecystitis, relies on a history consistent with biliary tract disease. Transabdominal ultrasonography reliably documents the presence of cholelithiasis. Ultrasound can provide other important information, such as common bile duct dilation, gallbladder polyps, porcelain gallbladder, or evidence of hepatic parenchymal processes. Cholesterolosis, or the accumulation of cholesterol found in gallbladder mucosal macrophages, can also be seen (Fig. 55-22). Even in the absence of frank stones, so-called sludge found in the gallbladder on ultrasonography, with appropriate symptoms, is consistent with biliary colic.
Acute Calculous Cholecystitis
Obstruction of the cystic duct from stone impaction eventually causes acute calculous cholecystitis. Temporary impaction, as seen with biliary colic, does not create inflammation as the obstruction resolves. If it does not resolve, however, inflammation ensues, with edema and subserosal hemorrhage, a process known as acute cholecystitis. Infection of the stagnant pool of bile is a secondary phenomenon; the primary pathophysiology is unresolved cystic duct obstruction. Without resolution of the obstruction, the gallbladder will progress to ischemia and necrosis. Eventually, acute cholecystitis becomes acute gangrenous cholecystitis and, when complicated by infection with a gas-forming organism, acute emphysematous cholecystitis (Fig. 55-23).
Diagnosis
Transabdominal ultrasonography is a sensitive, inexpensive, and reliable tool for the diagnosis of acute cholecystitis, with a sensitivity of 85% and specificity of 95%. In addition to identifying gallstones, ultrasound can demonstrate pericholecystic fluid, gallbladder wall thickening, and even a sonographic Murphy’s sign, documenting tenderness specifically over the gallbladder (Fig. 55-24). In most cases, an accurate history and physical examination, along with supporting laboratory studies and an ultrasound, make the diagnosis of acute cholecystitis. In atypical cases, a HIDA scan may be used to demonstrate obstruction of the cystic duct, which definitively diagnoses acute cholecystitis. Filling of the gallbladder during a HIDA scan essentially eliminates the diagnosis of cholecystitis. CT may show similar findings to ultrasound with pericholecystic fluid, gallbladder wall thickening, and emphysematous changes, but CT is less sensitive than ultrasound for the diagnosis of acute cholecystitis.
Treatment
Cholecystectomy, whether open or laparoscopic, is the treatment of choice for acute cholecystitis. The timing of operative intervention in acute cholecystitis has long been a source of debate. In the past, many surgeons advocated for delayed cholecystectomy, with patients managed nonoperatively during their initial hospitalization and discharged home with resolution of symptoms. An interval cholecystectomy was then performed at approximately 6 weeks following the initial episode. More recent studies have shown that when performed early in the disease process (within the first week), the operation can be performed laparoscopically with equivalent or improved morbidity, mortality, and length of stay, as well as a similar conversion rate to open cholecystectomy.3 Additionally, approximately 20% of patients initially admitted for nonoperative management failed medical treatment prior to the planned interval cholecystectomy and required surgical intervention. Initial nonoperative therapy remains a viable option for patients who present in a delayed fashion and should be decided on an individual basis.
Choledocholithiasis
Many common duct stones are clinically silent and may be identified only during cholangiography, if performed routinely during cholecystectomy. Without pain or an abnormal liver function panel, a setting in which selective cholangiography is not performed, 1% to 2% of patients following cholecystectomy will present with a retained stone. When performed routinely, intraoperative cholangiography identifies choledocholithiasis in approximately 10% of asymptomatic patients, suggesting that most choledocholithiasis remains clinically silent.4,5
Diagnosis
ERCP is highly sensitive and specific for choledocholithiasis (Fig. 55-25) and can usually be therapeutic by clearing the duct of all stones in approximately 75% of patients during the first procedure and approximately 90% with repeat ERCP. During the endoscopic procedure, a sphincterotomy is performed with a balloon sweep and extraction of the stone, all of which have a complication rate of 5% to 8%. Indications for preoperative ERCP prior to cholecystectomy include cholangitis, biliary pancreatitis, limited surgeon experience with common duct exploration, and patients with multiple comorbidities.
Alternatively, magnetic resonance cholangiopancreatography (MRCP) is highly sensitive (>90%) with an almost 100% specificity for the diagnosis of common duct stones (Fig. 55-26). As a noninvasive test, MRCP provides accurate imaging of the biliary tree but, in the setting of choledocholithiasis, does not provide a therapeutic solution. A clear cholangiogram by MRCP eliminates the need for ERCP. However, choledocholithiasis identified by MRCP requires intervention by some other method. With more surgeons becoming adept at laparoscopic common duct exploration, the inability of MRCP to remove common duct stones may prove less clinically relevant.
Treatment
Endoscopic Retrograde Cholangiopancreatography
Endoscopic sphincterotomy with stone extraction is effective for the treatment of choledocholithiasis. When used in the preoperative setting, it can avoid an open procedure and, when unsuccessful at removing stones, will alter intraoperative decision making. Common reasons for endoscopic failure include large stones, intrahepatic stones, multiple stones, altered gastric or duodenal anatomy, impacted stones, and duodenal diverticula. Sphincterotomy with stone extraction does not eliminate the risk of recurrent biliary stone disease. When managed by ERCP and sphincterotomy, almost 50% of all patients have recurrent symptoms of biliary tract disease if not also treated by cholecystectomy.6 More than one third of these patients eventually require cholecystectomy, suggesting that cholecystectomy should be offered to patients who present with choledocholithiasis. Interestingly, older patients (>70 years), have only a 15% rate of symptom recurrence, so cholecystectomy can be offered selectively to this patient population.
< div class='tao-gold-member'>
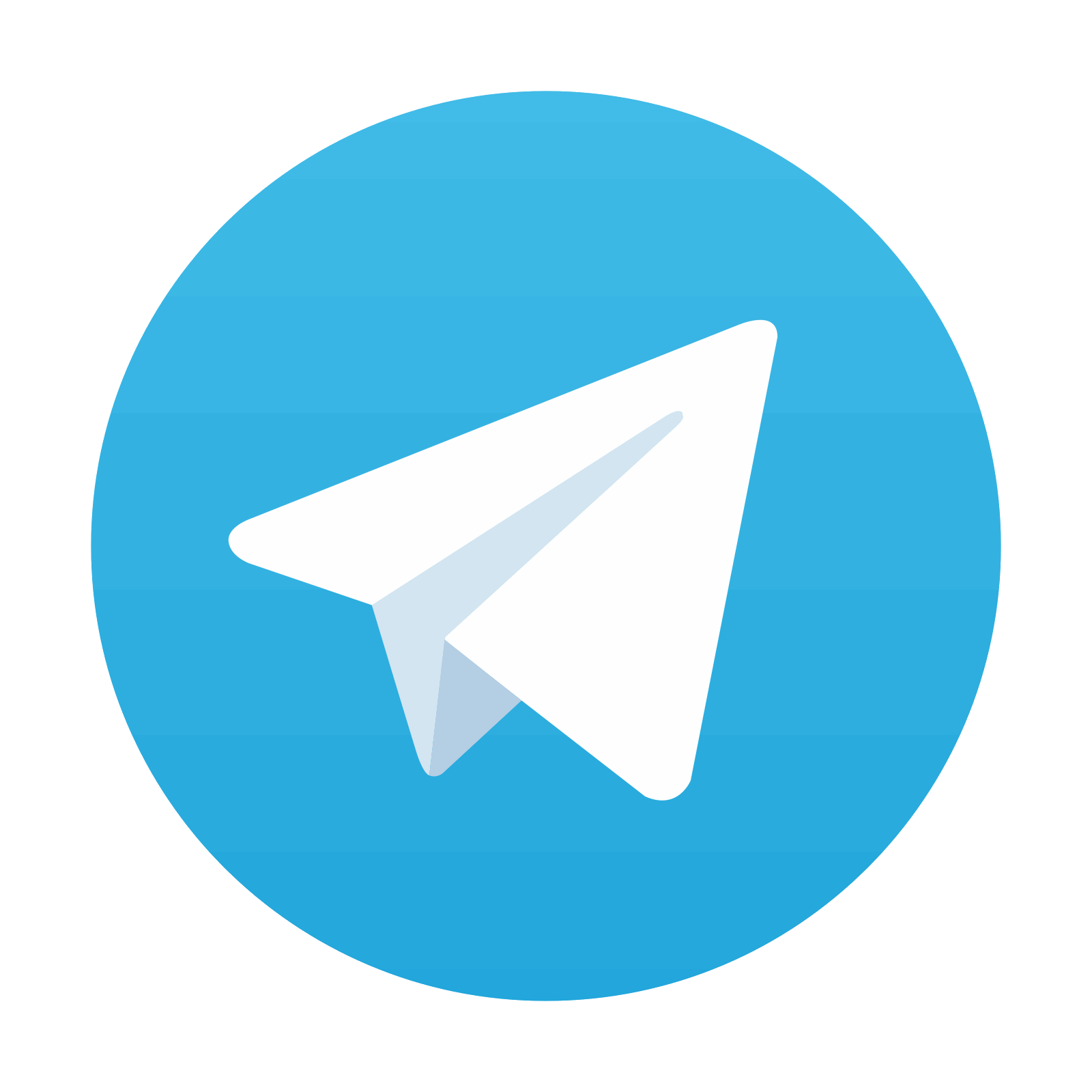
Stay updated, free articles. Join our Telegram channel

Full access? Get Clinical Tree
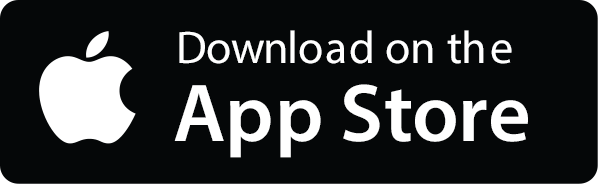
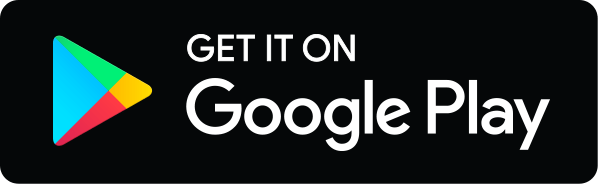