Fig. 14.1
General afferent and efferent pathways/connections of the hypothalamus (medial and lateral), the pituitary gland (adeno- and neurohypophysis), the limbic system, the thalamus, and the mesencephalon. Note the medial hypothalamus, via the neuroendocrine interface, controls the primary functions of the pituitary gland
The tight control of homeostatic functions that are modulated via the hormone system is accomplished by a multilevel, multi-hormone feedback mechanism. For example, the blood levels of releasing hormones and the released hormones can both be sensed within the medial hypothalamus (Fig. 14.2). Interestingly, electrical stimulation of nearly any region in the hypothalamus is likely to cause a cardiovascular response (change in activity). The hypothalamic effects on this system are mediated through both synergistic parasympathetic and sympathetic pathways. Additionally, afferent inputs for this control are many and include those from baro-, chemo-, and mechanoreceptors in the atria, ventricles, aorta, and elsewhere (see below).
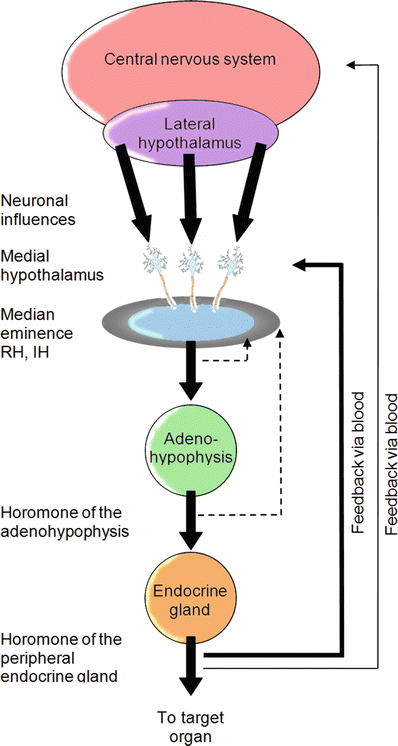
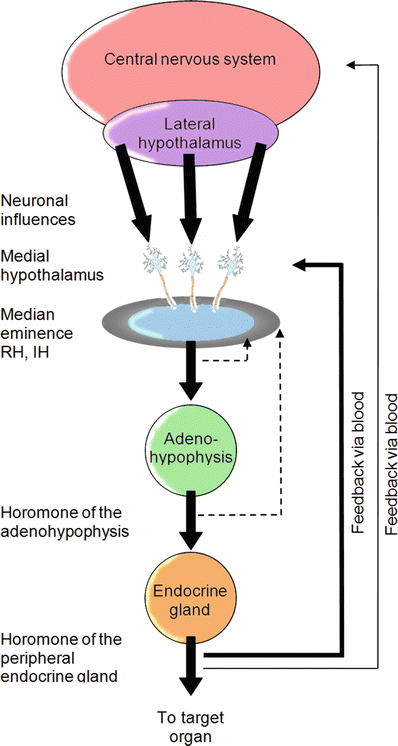
Fig. 14.2
Multilevel feedback loops are employed to regulate both hormone levels and neural responses. For example, the medial hypothalamus can sense blood levels of releasing hormones, the hormone levels released by the pituitary gland, and also those released by target endocrine glands
In other words, the hypothalamus controls the autonomic nervous system which is organized into parasympathetic and sympathetic subdivisions and integrates efferent and afferent fibers that regulate the activities of the majority of organs (including the heart), glands, and smooth musculature found in the human body. The presynaptic cell bodies of these neurons originate in the gray matter of the spinal column but are classified by fundamental differences. Anatomically, the origin of the sympathetic (thoracolumbar) division of the central nervous system lies between the first thoracic (T1) and the second or third lumbar section (L2 or L3). In contrast, the exiting fibers of the parasympathetic division (craniosacral) originate from both the medulla oblongata and sacral portion of the spinal cord (S2–S4). The primary neurotransmitter released during depolarization is another means of characterizing these two subdivisions of the autonomic nervous system. In the sympathetic branch, norepinephrine is the principal postsynaptic neurotransmitter, whereas acetylcholine is the chief neurotransmitter found throughout the parasympathetic fibers. The primary physiological response induced by each respective neurotransmitter is also a useful way to categorize the divisions of the autonomic nervous system. Such classifications are important considerations when investigating the autonomic nervous system regulation of the heart.
14.2 Sympathetic Anatomy
Cell bodies of presynaptic sympathetic efferent neurons are found in the paired lateral horns of the spinal cord, an area identifiable between the T1 and L2 or L3 vertebrae. The axons of these cells exit the interior of the spinal cord through ventral rootlets, which coalesce to form the larger ventral roots and eventually become ventral rami. Sympathetic fibers almost immediately divert into white rami communicantes (Fig. 14.3) branching from these spinal nerves, which connect them to paired columns of sympathetic ganglia located on either side of the spinal cord called the sympathetic trunks. Vertebrae from T1 to S5 have corresponding pairs of ganglia, each of which are interconnected with both ascending and descending nerve fibers, forming the complex column-like structures; these structures allow for rapid, coordinated, multi-segmental control of the cardiovascular system.
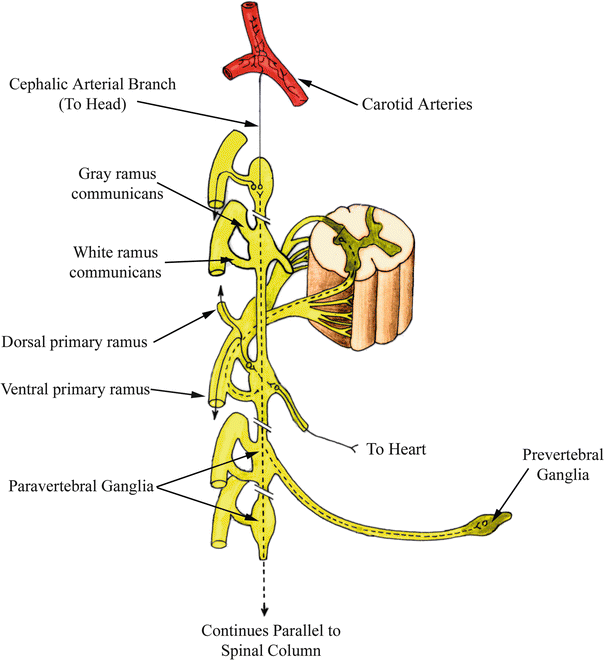
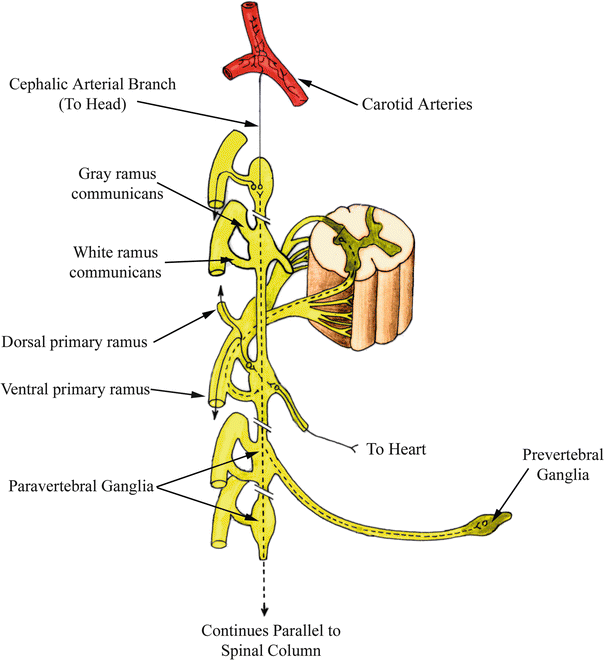
Fig. 14.3
Pathways of sympathetic motor fibers . The three potential paths of travel taken by presynaptic sympathetic motor fibers are shown. Preganglionic fibers traveling to the heart and other areas of the thoracic cavity synapse either immediately upon reaching the sympathetic trunk or traverse to other spinal levels to synapse. Complete passage through the paired trunks also occurs with prevertebral ganglia. Modified from Moore and Dalley [1]
Preganglionic sympathetic neurons synapse within the ganglia of the sympathetic trunk. The 10–20 nm separation distance [1] between presynaptic and postsynaptic cells is called the synaptic cleft, where neurotransmitter is released from synaptic vesicles. Note that acetylcholine is the neurotransmitter released from preganglionic neurons in both the sympathetic and parasympathetic branches of the autonomic nervous system (Fig. 14.4). This compound binds to receptors on postsynaptic cell bodies, causing localized depolarization of cell membranes, which may subsequently initiate action potentials that propagate down the axons of postsynaptic cells. In the sympathetic nervous system, norepinephrine is the primary postsynaptic neurotransmitter released. Such junctions can also be activated by epinephrine, and both can often be found with cotransmitters such as dopamine [2] and/or histamine [3]. Both norepinephrine and epinephrine play important roles during sympathetic stimulation of the heart, as will be discussed in later portions of this chapter.
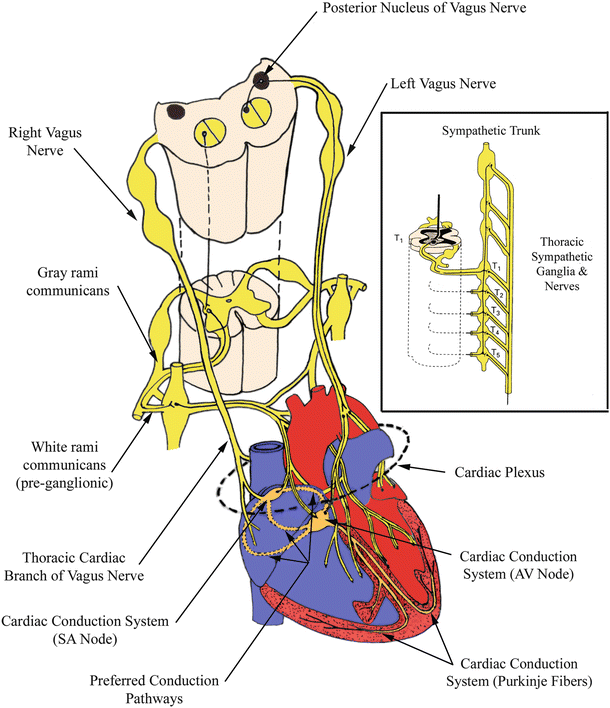
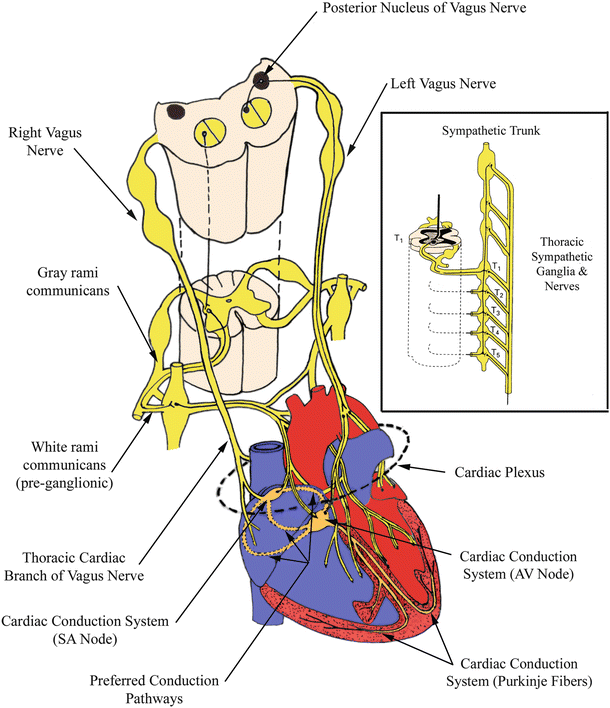
Fig. 14.4
Autonomic innervation of the heart . Vagal innervation of the right atrium can be observed. The area where many axons congregate just prior to innervation of the heart is depicted as the cardiac plexus. Sympathetic fibers branching from an arbitrary vertebral level of the paired sympathetic trunks is also illustrated. AV atrioventricular, SA sinoatrial. Modified from Martini [4]
Three primary paths of travel are commonly identified for presynaptic (also referred to as preganglionic) nerve fibers upon reaching the sympathetic trunk. A preganglionic fiber can immediately synapse on the cell body of a postganglionic fiber at the level of the trunk upon which the fiber entered. Preganglionic fibers can also follow a route that traverses through the sympathetic trunk, either ascending or descending to synapse within a higher- or lower-level ganglion. A third but less common path of travel for presynaptic neurons involves passing through the sympathetic trunk completely, then synapsing within a prevertebral ganglion in close proximity to the viscera innervated. In general, presynaptic fibers traveling to the head, neck, thoracic cavity, and limbs will follow one of the first two courses. Innervation of organs and glands located in the abdominopelvic cavity follow the third path through prevertebral ganglia (Fig. 14.3).
A variation of the second path discussed above occurs primarily with innervation of sweat glands, hair follicles, and peripheral arteries. Presynaptic nerves that arrive at the paired sympathetic ganglion, as discussed previously, traverse through the white rami communicantes. Rather than immediately continuing to peripheral regions of the body after synapsing, the postsynaptic neurons next travel through gray (unmyelinated) rami (Fig. 14.3) and exit along large bundles of nerve fibers called primary rami. From the primary rami, smaller nerve branches bifurcate and act to control the vasculature (either vasodilatation or vasoconstriction), hair follicle stimulation, and/or sweating. If the nerves are destined for the head, their cell bodies are located in the superior cervical ganglion and their axons follow the path of the carotid arteries to their respective destinations; the muscles of the eye are also innervated by this collection of sympathetic neurons.
Nerve fibers traveling from the central nervous system to a destination elsewhere in the body are termed efferents. In contrast, afferents carry sensory information from various locations in the body to the central nervous system. Frequently, these respective paths of travel occur in parallel but the flow of excitation is in the opposite direction; these fibers are typically bunched closely together to form larger nerve branches. The main nerve branches controlling the sympathetic behavior of the heart and lungs are the cardiopulmonary splanchnic nerves, which consist of both efferent and afferent fibers. Efferent nerves navigate a route originating from the ganglia in the upper cervical region (superior, middle, and inferior cervical ganglia) and the upper thoracic (T1–T5) levels of the sympathetic trunk. The inferior, middle, and superior cardiac nerves, in turn, originate from corresponding cervical ganglia and approach the base of the heart before splitting into smaller nerves and distributing themselves throughout much of the myocardium and vasculature. The cardiac plexus can be considered as groupings of the nerve bundles destined to and originating from the heart (Fig. 14.4); they are extremely difficult to visualize with the naked eye.
Incoming postsynaptic sympathetic neurons , which innervate the human heart, are highly concentrated around and near the aortic arch. Some of this innervation occurs throughout the aortic arch itself, as well as at the base of the ascending portion of this vessel. Many branches from these nerves continue down the aorta or under the arch to the pulmonary trunk, where they again diverge and track with the pulmonary arteries. Still more neuronal bifurcations have been identified which extend to reach other areas of the heart, including both atria and the right and left ventricles. Sympathetic innervation of both the sinoatrial and atrioventricular nodes is important for control of heart rate, but has not been distinguished in greater concentration at these areas relative to elsewhere in the atria [5, 6]. Such nerves have been identified epicardially, often following the path of the coronary arteries and veins [5, 7]. In general, sympathetic innervation is more highly concentrated in the ventricles than in the atria [8]. Within the ventricles, a higher distribution is observed toward the base of the heart as opposed to the apex, with nerves in the epicardium at a slightly greater concentration than in the endocardium. This latter tendency is also evident in the atria [8].
14.3 Adrenal Medulla
The sympathetic nervous system also controls the hormonal secretions of the paired suprarenal (adrenal) glands lying within the abdomen, considered as components of the endocrine system. Specifically, preganglionic fibers, with their cell bodies located in the lower thoracic (T10–T12) segments of the spinal cord, travel to the adrenal medulla by means of the abdominopelvic splanchnic nerves. It is in the medulla, or central portions of these suprarenal glands, that both norepinephrine and epinephrine are released into the bloodstream [1]. The release of these catecholamines into the blood is considered a postsynaptic response initiated from this type of sympathetic activation. Specifically, the cortex surrounding the medulla portions of the adrenal glands are responsible for producing multiple steroid hormones. As blood drains from the highly vascularized cortex to the medulla, the aforementioned hormones can be used to convert norepinephrine to epinephrine. The respective mechanisms of action for these two similarly structured catecholamines will be discussed later in this chapter .
14.4 Parasympathetic Anatomy
The parasympathetic (craniosacral) nervous system branches from four paired cranial nerves and the lower sacral segment of the spinal cord (S2–S4). The vagus nerve (cranial nerve X) is the main effector pathway for modulating cardiac function, i.e., controlled by input from the parasympathetic subdivision of the autonomic nervous system (Fig. 14.4). Efferent fibers of the vagus nerves originate in the medulla oblongata and weave through the neck alongside the carotid arteries to the thoracic and abdominopelvic cavities, bifurcating many times along the way to innervate an assortment of organs including the heart. More specifically, the efferent fibers of the cranial parasympathetic branch communicate with blood vessels of the head and other viscera; the sacral portion of the spinal cord innervates viscera of the lower abdominopelvic cavity like the urinary bladder and colon, as well as their respective blood vessels.
Unlike the short sympathetic preganglionic fibers, the parasympathetic division of the autonomic nervous system generally has very long preganglionic fibers and short postsynaptic fibers. Hence, the parasympathetic ganglia are often located very proximal to, or actually within, the target organ. As discussed previously, acetylcholine is this branch of the autonomic nervous system and is the primary neurotransmitter released at both preganglionic and postganglionic junctions.
Within the heart, the majority of the parasympathetic ganglia are located near the sinoatrial node and within the conduction tissue surrounding the atrioventricular node [5]. Consequently, the right and left vagus nerves envelope a large and overlapping portion of the atria, where short postsynaptic fibers from both branches act on the conduction centers of the heart (Fig. 14.4). However, the endings of the right vagus primarily innervate the sinoatrial nodal region, while a great number of projections from the left are typically observed innervating the atrioventricular node [5]. In fact, high concentrations of vagal innervation situated within a localized region of epicardial fat near the atrioventricular node have been described for the human heart, and it is hypothesized that nerves located within this “pad” have little effect on behavior of the sinoatrial node [9]. Thus, it is likely that each region is controlled independently of the other. Parasympathetic junctions are also observed in the ventricles, but only at one-half to one-sixth as frequently as sympathetic innervation [8]. Nevertheless, nerves of the parasympathetic division of the autonomic nervous system outnumber those of the sympathetic division in the atria by some 30–60 % [8]. Interestingly, while sympathetic innervation has been described to occur at approximately an equal distribution between the endocardial and epicardial surfaces of the heart, vagal nerve endings are reportedly located at almost twice the density (1.7–1) transmurally (within the myocardium) when compared with their epicardial distribution [8] .
14.5 Baroreceptors
The autonomic nervous system plays a vital role in the overall regulation of blood pressure within the human body. Specialized receptors sensitive to changes in arterial diameter are located at various strategic locations within the upper thoracic cavity and neck; these nerve clusters are commonly known as arterial baroreceptors . Substantial groupings of such baroreceptors can be found at the arch of the aorta and on the internal carotid arteries (just distal to where the common carotid bifurcates). This focal density of carotid baroreceptors is also referred to as a carotid sinus . The majority of receptors are located at areas within these arteries where the walls decrease in thickness, enabling pressure changes to be somewhat magnified at these locations (Fig. 14.5). Under even minimal pressure increases, these large arteries will elicit detectable wall dilatations. In contrast, under decreased pressure, their internal diameters will decline, also resulting in changes of the firing frequencies of these receptors. The axons of these afferent neurons travel from baroreceptors along parasympathetic corridors to the medullary cardiovascular center in the brainstem. Under increases in the mean pressure detected by these arterial baroreceptors, efferent sympathetic stimulation will decrease, which is accompanied by an increase in parasympathetic outflow to the heart. This neural activity is intended to return the mean blood pressure to a normal state. The opposite autonomic response would commence if the mean arterial pressure at the baroreceptor locations decreased. The synergistic functioning, briefly noted here between both divisions of the autonomic nervous system, will be discussed in much greater detail in the following sections. It should be noted that the direct application of pressure to an individual’s neck at the site of the carotid sinus can induce a reflex decrease in blood pressure, even to the point of causing unconsciousness, i.e., the so-called sleeper hold.
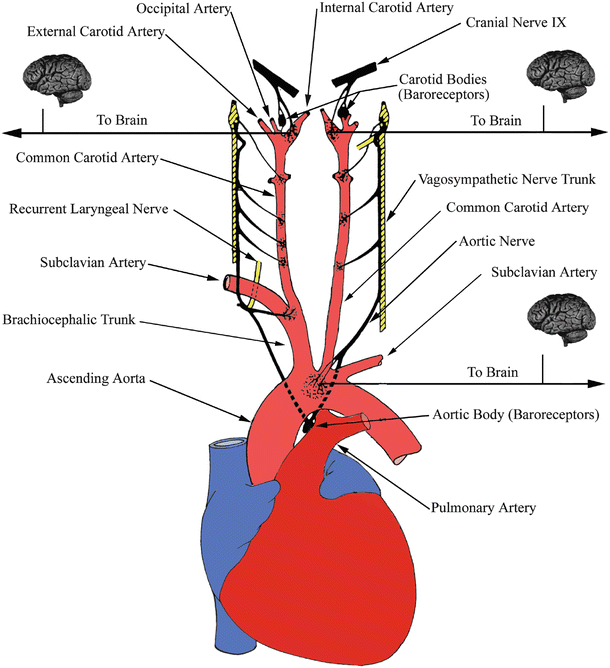
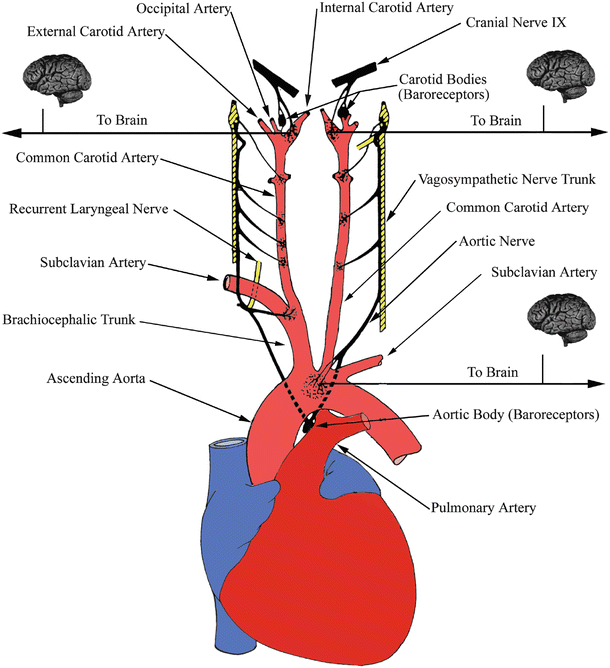
Fig. 14.5
Arterial baroreceptors. Receptors located at the bifurcations of the carotid arteries and aortic arch convey information to the brain and vasculature to help regulate pressure fluctuations. Modified from Mountcastle [10]
14.6 Homeostasis
The tendency to maintain the internal environment of the body at a relatively constant level is known as homeostasis. The heart itself exerts perhaps the greatest control on countless parameters involving the circulation of blood throughout the body. The heart communicates with the central nervous system via both branches of the autonomic nervous system. Both the sympathetic and parasympathetic divisions work together in synergistic control of their antagonistic influences, in order to prevent potentially harmful fluctuations in many vital bodily functions. While it is obvious that significant changes do occur, an array of physiologic responses involving the heart (homeostatic control mechanisms) mediated by the autonomic nervous system quickly reverses these changes to return within the reasonable ranges required to maintain overall health. In general, homeostatic control functions are the underlying determinants of the relative degrees of both parasympathetic and sympathetic activation .
14.7 Hypothalamic Control
More specifically, the autonomic control of the heart is greatly influenced by activity within the portion of the brain known as the hypothalamus. Afferent fibers from the brainstem (medulla oblongata) and spinal cord convey information via the autonomic afferent system to the hypothalamic nuclei within the central nervous system [11], whereas impulses that leave the hypothalamus travel along efferent fibers to the various sympathetic and parasympathetic ganglia as noted above. Most parasympathetic response signals have been determined to originate from the anterior portions of the hypothalamus, while sympathetic activity stems primarily from the posterior portions [6, 11]. Direct electrical stimulation of specific sites within the hypothalamus can initiate preprogrammed, simultaneous, patterned changes in heart rate, blood pressure, and peripheral resistance [5] (Fig. 14.6).
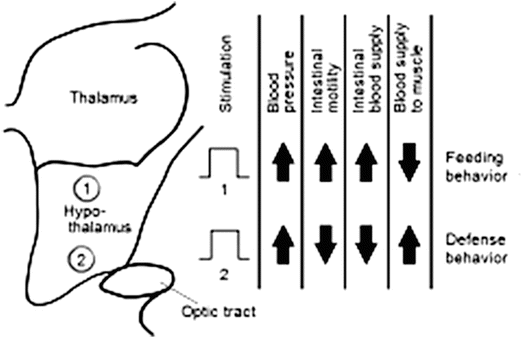
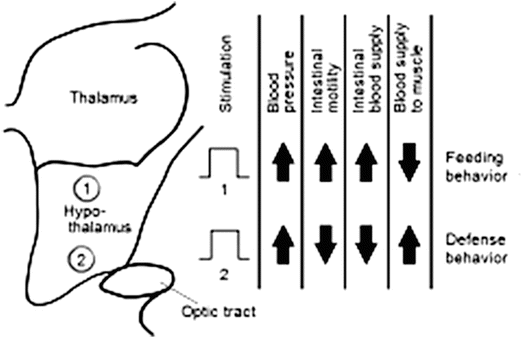
Fig. 14.6
The body’s cardiovascular responses are more or less under involuntary control and are thus regulated by the autonomic nervous system (ANS). For example, stimulation of any region of the medial hypothalamus will induce characteristic changes in cardiovascular responses. These patterned responses are commonly associated with innate behavior responses that are also attributed to the hypothalamus, such as feeding or defensive behaviors, which in turn appropriately modulate other body systems under ANS control
As noted above, afferent axons from the aortic and carotid baroreceptors principally travel to the medullary cardiovascular centers, with neural pathways continuing onward to the hypothalamus [6]. Temperature regulation of the body is also centered within the hypothalamus. Thus, during exposure to cold, the hypothalamus initiates appropriate autonomic responses to maintain body temperature, such as vasoconstriction and shivering. The contraction of the peripheral vasculature motivates a redistribution of blood flow to vital organs like the heart and brain, in order to maintain their suitable function [2]. The shiver reflex induced by the hypothalamus increases heat production which, in turn, causes additional adjustments in blood flow and cardiac activity. The opposite outcome occurs during exposure to high degrees of heat, such that sweating is initiated via postganglionic sympathetic neurons and vasodilation of the vasculature supplying the skin is amplified. The regulation of bodily processes is an important responsibility of the hypothalamus, and it performs such tasks via the autonomic pathways, hence regulating countless systems within the body simultaneously. Keeping this relative state of constancy throughout the body regardless of extreme external changes is referred to as homeostasis; in nearly all cases, there are direct effects on cardiac performance.
< div class='tao-gold-member'>
Only gold members can continue reading. Log In or Register a > to continue
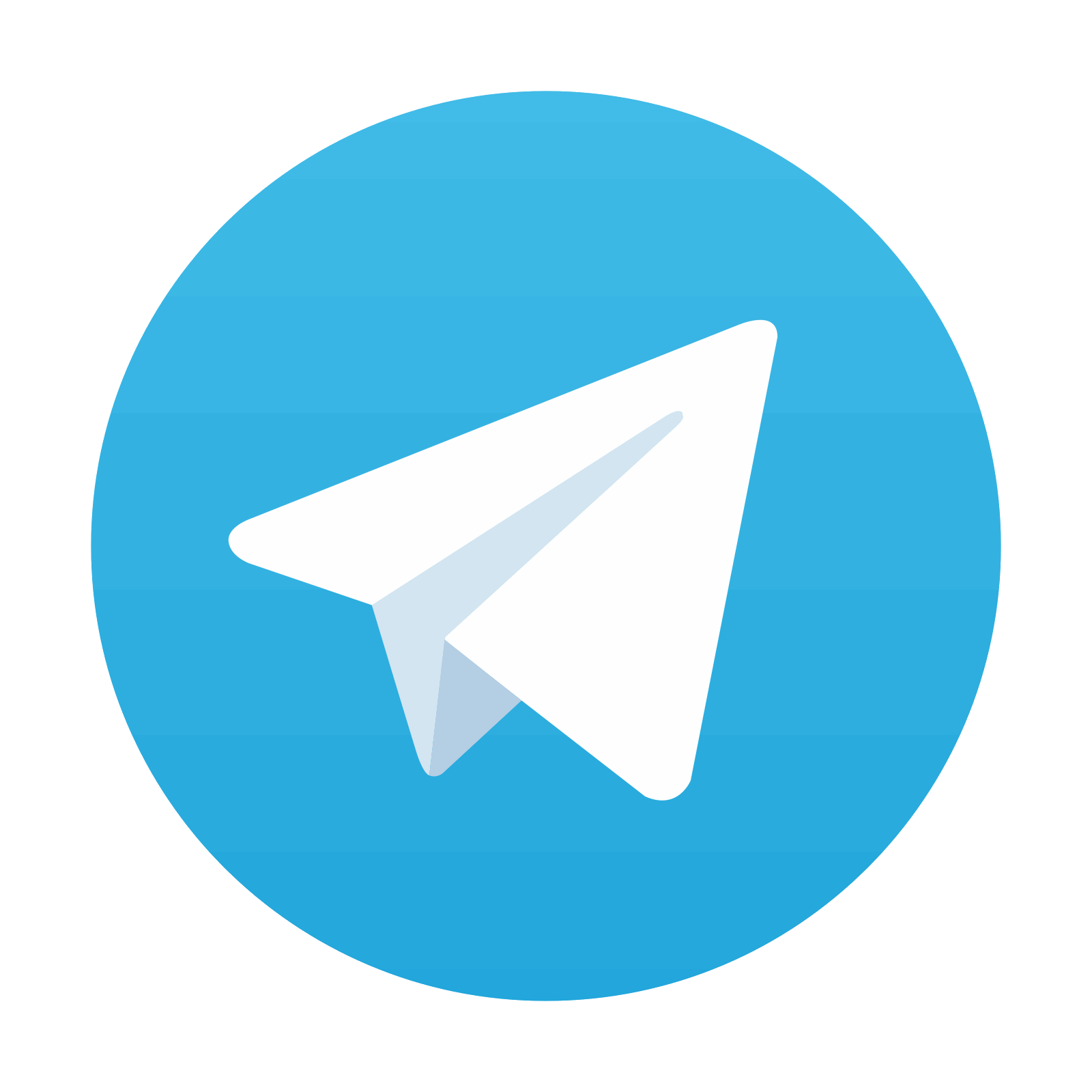
Stay updated, free articles. Join our Telegram channel

Full access? Get Clinical Tree
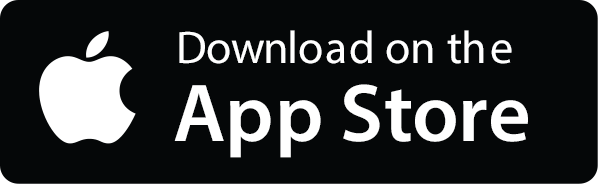
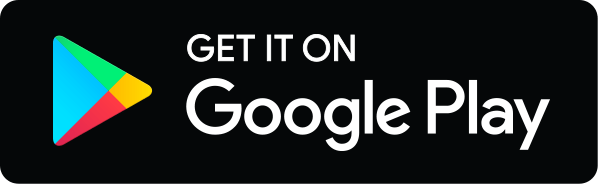