In the previous chapter, methods of estimation of cardiac output and organ blood flow indices were discussed. These are essential components of the echocardiographic assessment of adequacy of cardiovascular function. The current chapter focuses upon the noninvasive assessment of systolic and diastolic cardiac function.
Assessment of systolic function is a basic component of functional echocardiography. Shortening fraction (SF), also known as fractional shortening, is the most commonly used index of left ventricular systolic function in children. This index reflects the degree of myocardial fiber shortening during systole. As the loading condition affects the initial myocardial fiber length (preload effect) and the degree of shortening (afterload effect), this index of cardiac systolic function is considered to be load-dependent. Therefore, changes in loading condition impact the myocardial contractility as assessed by SF without necessarily a change in the inherent myocardial function. In addition, the most commonly used method to measure SF has significant limitations (see below). Nevertheless, SF provides the clinician with a simple and quick way to assess myocardial systolic function. Assessment of SF is generally performed with M-mode echocardiography (see also Chapter 5).
The normal value of SF for all gestational and postnatal ages is considered to be in range between 27% and 42%. In hemodynamically stable preterm infants ≤30 weeks’ gestation, average SF was 34%±5% (range 23–48%) in the first 3 postnatal days and 38%±6% (range 25–51%) from 4–14 days after birth.1
The patient should be placed in a supine position.
For better resolution, a high-frequency (10–12 MHz) probe is preferable.
Optimal visualization of the left ventricle (LV) can be achieved using standard parasternal short- or long-axis views. In neonates it is preferable to use a parasternal short-axis view.
Using the parasternal short-axis view, obtain a cross section of the LV at the level of the papillary muscles or the tip of mitral valve leaflet (Figure 8-1). The latter is preferred in neonates. Care should be taken to obtain a two-dimensional (2D) image of the cross section of the LV in such a way as to have mitral valve closure as a horizontal line. Next, place the M-mode line to be perpendicular to the septum, the above-mentioned horizontal line, and the posterior wall, with about equal distance between the two papillary muscles. Initiating the M-mode setting on the ultrasound machine will yield the motion of the various structures along the M-mode line.
FIGURE 8-1.
A schematic drawing of the short-axis view of the heart at the level of mitral valve tip. The dotted line on the drawing depicts where the M-mode cursor line is placed. The motion of various structures along this dotted line is displayed in the M-mode tracing. For the purpose of measuring the shortening fraction, an M-mode tracing of the short-axis view of the heart at the level of papillary muscles or tip of the mitral valve is obtained. In neonates, it is preferable to obtain M-mode at the level of the tips of mitral valve. Left ventricular internal diameter in diastole (LVIDD) and systole (LVIDS) are measured as shown.

It is customary to start by measuring the right ventricle internal diameter in diastole followed in sequence by measuring the ventricular septal thickness, LV internal diameter (LVIDD), and posterior wall thickness in diastole. Subsequently, in systole the septal thickness, LV internal diameter (LVIDS), and posterior wall thickness are measured. Normal data for the above measurements varies for different gestational ages and weights.2–4 For the purpose of estimating SF, only LVIDD and LVIDS are needed. The echo system will automatically calculate SF, provided the above measurements are assigned using the analysis package of the system. SF is calculated as [(LVIDD−LVIDS)/LVIDD]×100. The above measurements can also be performed using 2D images. However, M-mode has better temporal resolution and is preferable in the neonatal population.5
The main limitation of SF in estimating contractility and systolic function is generalizability of relative linear movement of the two points along the M-mode cursor (the endocardial borders of the ventricular septum and the LV posterior wall) to the entire LV myocardium. While in normal myocardium this is not an issue, in pathological conditions, where accurate estimate of systolic function is even more important, SF may not reflect the overall contractile function of the LV. In the presence of myocardial dysfunction, different parts of the myocardium can have different degrees of fiber shortening. For example, the LV might have greater shortening in the lateral direction compared to the anteroposterior direction. In such a case, the SF (which measures shortening in anteroposterior direction) would underestimate overall LV systolic function (Figures and Videos 8-2 to Figure 8-4). In the case of elevated pulmonary artery pressure, the septal wall may be flattened or bowed into the LV, or the motion might be paradoxical (Figure and Video 8-5). Similarly, the septal and posterior wall motion might be dyskinetic in dilated cardiomyopathy, rendering SF much less useful as a measure of myocardial function (Figure and Video 8-6). In such cases, measuring changes in area can be more useful than SF. To measure fractional change in area, a 2D cross section of the LV at the level of the tips of the mitral valve is obtained using parasternal short-axis view (similar to SF measurement). Fractional area change is calculated using the formula [(LVAD−LVAS)/LVAD]×100, where LVAD and LVAS are LV area (traced by planimetry) at the end of diastole and systole, respectively. There are limited data on the normal ranges in neonates; in adults, a value > 36% is considered normal.6 Alternatively, volumetric changes (ejection fraction) can be used when septal motion is abnormal, as will be discussed below. Another limitation of SF is load dependency; however, most echocardiographic indices of systolic function share this limitation.
FIGURE and VIDEO 8-2.
Short-axis view of the left ventricle in an extremely preterm infant. In the video, note the greater change in left ventricular diameter in the lateral direction compared to the anteroposterior direction. (Also see Figures 8-3 and 8-4.)

FIGURE 8-3.
On the left, a short-axis view of the left ventricle at the level of the tip of the mitral valve is shown. Note the dotted line representing the thin section of the heart where the M-mode tracing is obtained. On the right, the M-mode tracing with the measurements of left ventricular internal diameter in diastole (LVIDD) and systole (LVIDS) is displayed. Using these measurements, the shortening fraction (SF) is 19%.

FIGURE 8-4.
Two-dimensional views of the left ventricle in diastole (left) and systole (right). Shortening fraction (SF) is calculated based on 2D measurements of left ventricular internal diameter in diastole (LVIDD) and systole (LVIDS) in two different orientations (the solid and dotted lines). Limitation of SF in estimating systolic function is evident in this example, as using different orientations yield very different results.

Ejection fraction (EF) is another measure of the LV systolic function. This index is an estimate of the proportion of LV volume that is ejected during each cardiac cycle. There are several ways to estimate EF. Two echocardiographic methods that can be used to calculate EF include (1) methods based upon linear LV M-mode minor-axis dimensions and (2) modified Simpson’s biplane. The area-length, or bullet, method is another way to estimate EF.7 This method is more laborious, as it involves measurements in both minor and major axis and is not further discussed.
This method is essentially the same as the method of measuring SF. The parasternal short axis view of heart and M-mode are used to measure LVIDD and LVIDS. EF is calculated by [(LVIDD3−LVIDS3)/LVIDD3]×100, and the normal value ranges from 56% to 78%. Given the fact that one is cubing the same diameter used in the calculation of SF, the limitations of this index are also the same as those for SF mentioned above. Therefore, this method of calculation of EF is not useful when the same conditions exist that interfere with the utility of SF (discussed above).
The patient should be placed in a supine or in a slight left lateral decubitus position.
For better resolution, a high frequency (10–12 MHz) probe is preferable
Optimal visualization of the LV for this purpose can be achieved using an apical four- or two-chamber views (Figure and Video 8-7).
Using the apical view, obtain a longitudinal section of the LV through the mitral valve. Adjust the image gains and contrast (compression) so that there is good endocardial border definition and discrimination between the blood/tissue interface. Care should be taken to obtain a 2D image where the endocardial border is clearly visible. In addition, one must be aware of potential of foreshortening the LV.
First, the endocardium and mitral valve plane are traced during diastole, and then the longitudinal axis is determined by measuring the distance from the apex to the midpoint of mitral valve plane (Figure 8-8). The mitral valve plane is determined by a line connecting the lateral and medial hinge points. The echocardiography machine built-in software calculates the volume based on the assumption that the LV is cone shaped and consists of a series of discs. The calculated volume is the end-diastolic volume (EDV). The same process is then repeated at end-systole to determine the end-systolic volume (ESV). The EF (expressed as a percentage) is calculated as [(EDV−ESV)/EDV]×100, with a normal value being 56–78%. This method can also be used to estimate stroke volume (EDV-ESV) and ventricular output ([EDV−ESV]×heart rate). Of note, when one is tracing the blood-endocardium interface, it is customary to include the papillary muscles in the blood pool. Newer echocardiography systems have the autotrace capability.
FIGURE 8-8.
An example of measurement of ejection fraction (EF) using the modified Simpson method. Some echocardiography system can autotrace the endocardium as in this example. The end-diastolic volume (EDV) and end-systolic volume (ESV) are 6 and 2 ml, respectively. Using [(EDV−ESV)/EDV]×100, the EF is 67%.

The main limitation of the modified Simpson’s method in estimating EF is the difficulty in obtaining high-quality images with adequate enough resolution to visualize clearly the endocardial border. The procedure requires slightly more time to perform than SF, which is another shortcoming of the method. Finally, load dependency is another limitation of this method.
Velocity of circumferential fiber shortening (VCF) is another measure of contractility and systolic function. Like SF, VCF is also load-dependent, but it is thought to be less preload-dependent and less sensitive to abnormal septal motion. VCF is significantly affected by changes in heart rate; as such, it is often corrected for heart rate (VCFC). This measure of contractility is not currently part of routine assessment of cardiac function. However, as discussed below, one needs to calculate VCFC in order to assess the stress-velocity index, a load-independent index of contractility.
The patient should be placed in a supine position.
For better resolution, a high-frequency (10–12 MHz) probe is preferable
To measure VCF, two views of the heart are needed. The first view can be a parasternal short-axis (preferable) or standard parasternal long-axis. This is the same view used for measuring SF. The second view is apical five-chamber.
The first step of the procedure is the same as the one used for measuring SF (see above). In this step, the relative motion of interventricular septum and posterior wall are assessed by M-mode. The second step involves Doppler interrogation of the aortic flow at the valve level from the apical five-chamber view. After obtaining a four-chamber apical view of the heart, turn the transducer slightly counterclockwise and anteriorly to visualize the LV outflow track and proximal part of ascending aorta. Take care to obtain an upright 2D image of heart. Next, place the Doppler gate at the aortic valve level. Activating the PW-Doppler button will produce the spectral Doppler envelope of aortic flow velocity. From this tracing, the LV ejection time (LVET) can be calculated using the duration of aortic flow. Alternatively, LVET can be calculated from the duration of aortic valve opening using M-mode tracing of aortic valve in parasternal long-axis view.
The first step in measuring VCF is the same as for SF. In this step, LVIDD and LVIDS are measured. Next, LVET can be measured using the duration of the aortic flow spectral Doppler envelope obtained in apical five-chamber view (discussed above). Care must be taken to ensure that the heart rate is similar during the M-mode and Doppler image acquisitions. Alternatively, using the M-mode at the level of aortic valve in parasternal long-axis view, LVET can be calculated as the time span from opening to closure of aortic valve. The echo system will automatically calculate VCF, provided the above measurements are assigned using analysis package of the system. VCF is calculated as [(LVIDD−LVIDS)/LVIDD]/LVET. However, VCF is affected by changes in heart rate (because LVET is heart rate-dependent). Therefore, VCF is often corrected for heart rate to yield VCFC. To calculate the VCFC, the duration of the cardiac cycle (R-R interval) is measured. This can be obtained from the M-mode image used for measurement of LVIDD and LVIDS; in this view, two identical points from either the electrocardiogram (ECG) or the M-mode tracing can be used to measure the R-R interval. Alternatively, the spectral Doppler tracing of the aortic valve can be used (provided the heart rate is similar to the M-mode); the time span between two identical points on the ECG or from the start of one Doppler envelope to the next is used to derive the R-R value. Once the R-R interval is obtained, the echocardiography machine software calculates the VCFC using the following formula: VCFC=VCF×RR. The normal VCFC is 1.28±0.22 circ/s in full-term neonates and 1.08±0.14 circ/s in the older pediatric population.8 In hemodynamically stable preterm infants, we have found an average VCFC to be 1.22±0.26 circ/s (range 0.78–1.77) in the first 3 postnatal days and 1.45±0.27 circ/s (range 0.89–2.21) from 4–14 days after birth.1
In general, the limitations of VCFC are similar to SF except that it is less sensitive to abnormal geometry of the ventricle (see above).
The stress-velocity index is a measure of myocardial contractility that is largely load-independent. In other words, the stress-velocity index represents true contractile function of the myocardium, whereas SF and VCFC represent systolic function of the myocardium for a given preload and afterload. Both load-dependent and load-independent measures of contractility provide important and clinically relevant information. SF and VCFC provide information on the myocardial systolic function with the current loading conditions. Conversely, in the case of the stress-velocity index, one can gain insight into the myocardial contractility capability relative to the normal heart, independent of any loading conditions. The stress-velocity index is defined as the relationship between VCFC and wall stress (WS). Prior to any further discussion of the index, it is important to first describe the derivation of WS, which is given in the subsequent section.
Wall stress (WS) is a measure of afterload; afterload is the force that the LV has to overcome to pump blood out of the ventricle into the aorta during systole. This force can be estimated, as it exerts its effect per unit area in the direction of apex-base (meridional) or in the direction of minor axis (circumferential). Because meridional WS is easier to estimate and correlates well with circumferential WS, meridional WS is more commonly used. In this book, all further comments and discussions on WS are made with reference to meridional WS.
While the concept of preload in terms of end-diastolic volume is relatively easy to grasp, afterload can be more difficult. Indeed, afterload is often confused with systemic vascular resistance (SVR). While related, WS and SVR are two different indices of cardiovascular function. SVR is resistance to flow generated by characteristics of vasculature, vascular tone, and viscosity of the blood, and according to Ohm’s law, SVR=ΔP/Q, where ΔP is the pressure gradient (Mean blood pressure – Central venous pressure) and Q is the cardiac output. On the other hand, WS depends on the LV cavity pressure and diameter and LV wall thickness, according to the law of Laplace. The law of Laplace states that surface tension (ie, WS) is directly related to LV cavity pressure and diameter and inversely related to wall thickness. Because the pressure, diameter, and wall thickness change during the cardiac cycle, WS also varies during the cardiac cycle. The best indicator of afterload as a limiting factor for myocardial contractility is the end-systolic WS. While LV cavity diameter and wall thickness can be measured noninvasively by echocardiography, end-systolic ventricular pressure can only be measured invasively. Tracing the arterial pressure waveform may be helpful, as the arterial pressure at the time of the dicrotic notch on the pressure waveform corresponds to the ventricular pressure at the end of systole. However, this type of tracing, which can be obtained noninvasively, is cumbersome to perform routinely. Fortunately, several investigators have shown that the mean arterial blood pressure correlates well with end-systolic ventricular pressure. Because this value is much easier to measure, it can be used for the calculation of end-systolic WS.9
The patient should be placed in a supine position.
For better resolution, a high-frequency (10–12 MHz) probe is preferable.
To measure wall stress, the parasternal short axis view is used.
The procedure is the same as the one used for measuring SF (see above).
The LVIDS and posterior wall thickness in systole (PWS) are measured using M-mode tracing image. Blood pressure at the time of M-mode tracing acquisition is recorded (as discussed above, mean blood pressure is an adequate surrogate for end-systolic ventricular pressure). The echo system will automatically calculate the WS, provided the above measurements are assigned using analysis package of the system. WS is calculated as (0.334 P×LVIDS) / (PWS [1+ (PWS/LVIDS)]). The normal WS is 30.2±8.7 g/cm2 in full-term neonates and 37.3±8.8 g/cm2 in the older pediatric population.8 In hemodynamically stable preterm infants, we have found an average WS to be 28.4±12.3 g/cm2 (range 8.2–86.6) in the first 3 postnatal days and 20.1±8 g/cm2 (range 7.4–43.6) from 4–14 days after birth.1
Inaccuracy in estimating LVIDS due to septal motion abnormalities can affect the accuracy of WS.
Now that VCFC and WS have been defined, the discussion on the stress-velocity index can continue. When one plots the VCFC obtained for any given WS value, the graph that is produced is known as the stress-velocity index. There is an inverse relationship between the two parameters, such that the graph that is produced is negative and linear: the higher the wall stress, the lower the VCFC (Figure 8-9). However, in neonates the y-intercept of this regression line is higher and the slope is steeper than in older children. In other words, the myocardium functions at a higher baseline contractile state and is more sensitive to increase in afterload than older children. Some studies have found an even higher y-intercept and steeper line in preterm compared to full-term neonates,10,11 while others found no such a difference.12
FIGURE 8-9.
The blue line represents the normal inverse relationship that exists between the heart rate-corrected velocity of circumferential shortening (VCFC, myocardial contractility) and the wall stress (WS, afterload) in children. In neonates, the y-intercept of the regression line (red) is higher and the slope is steeper than in older children. In other words, the myocardium functions at a higher baseline contractile state and with an increase in afterload the contractility of immature myocardium decreases more than that of the mature heart of older children. (Modified with permission from Rowland DG and Gutgesell HP. Noninvasive assessment of myocardial contractility, preload, and afterload in healthy newborn infants. Am J Cardiol. 1995;75(12):818–821.)

The stress-velocity index is usually presented as a z-score. Z-score is based upon population normative data, with one z-score equivalent to one standard deviation. Normal contractility is considered to be with ±2 standard deviations; therefore, normal is considered a z-score of ± 2, with >+2 and <−2 representing a hyperdynamic state and poor contractility, respectively. For a given end-systolic WS, the z-score is estimated as follows: [VCFC (actual)−VCFC(calc)] /SD, where VCFC (actual) is the measured heart rate-corrected velocity of circumferential fiber shortening, VCFC(calc) is the calculated VCFC based on measured WS and the normal relationship between VCFC and WS (based upon a normal population), and SD is the standard deviation of VCFC of the normal population. Based upon normative data in full-term neonates,8 VCFC(calc) is calculated as −0.020 mWS + 1.88. Standard deviation (SD) of VCFC in normal population is 0.22. For example, if measured VCFC and WS are 0.91 circ/s and 10 g/cm2, respectively, the VCFC(calc) is (−0.020×10)+1.88 or 1.68 circ/s. The z-score would be [0.91–1.68]/0.22 or – 3.5. In this example the contractility is reduced (3.5 SD below the mean for normal population). In hemodynamically stable preterm infants, VCFC (calc) can be calculated using −0.0123 mWS + 1.58, with SD 0.23 for the transitional period (first 3 days) and −0.0241 mWS +1.93 with SD 0.27 for postnatal day 4–14.1
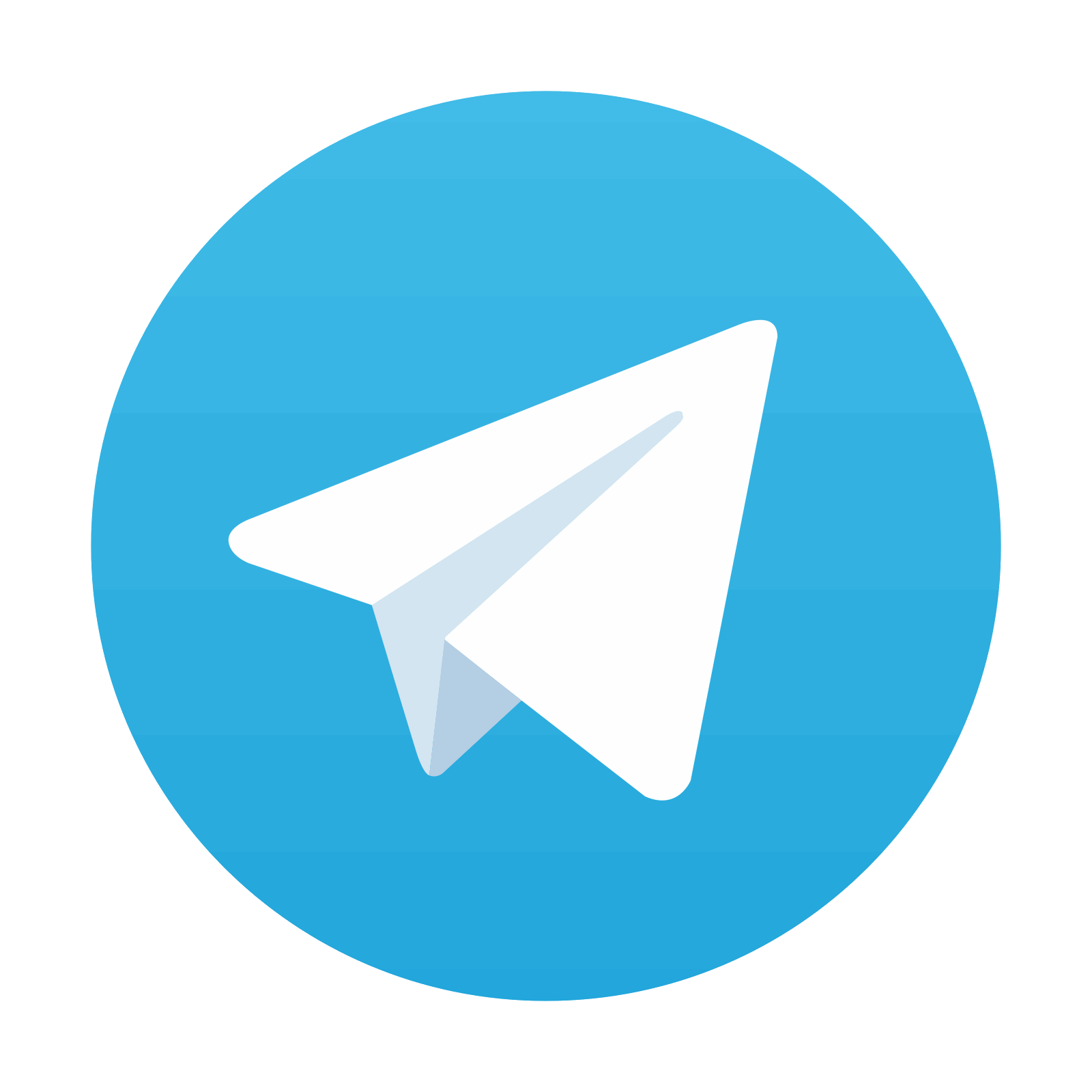
Stay updated, free articles. Join our Telegram channel

Full access? Get Clinical Tree
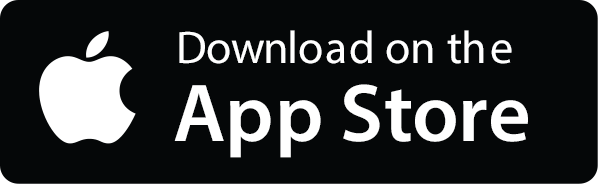
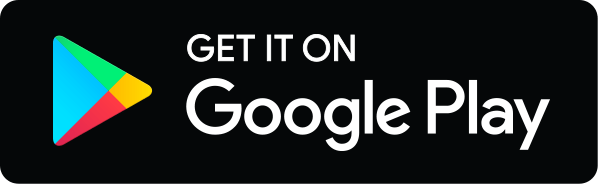