The measurement of blood flow through any vascular structure using ultrasound requires two basic pieces of information: the velocity of blood flow and the cross-sectional area of the vessel. The velocity of blood flow can be measured using spectral Doppler. As pulsed wave (PW) Doppler allows for measurement of velocity at a specific point in the vessel (ie, range resolution), it is the preferred mode of spectral Doppler. When measuring the velocity, the echocardiographer must take care to use an angle of insonation as parallel as possible to the direction of flow. If the ultrasound beam is intercepting the blood flow at an angle, the velocity will be underestimated (see Chapter 6). In clinical practice, an angle of less than 20° is generally considered acceptable, as the effect on velocity is negligible. An angle greater than 20° will require angle correction, which often produces inconsistent results. Another important point in measuring the velocity is that, in the presence of a turbulent flow, the measured velocity by PW Doppler cannot be used to estimate the flow because of aliasing that occurs with higher velocities (see Chapters 1 and 4). Therefore, the operator should assess the flow by color Doppler first to ensure that the flow is laminar before attempting to obtain the velocity by PW Doppler.
In the measurement of the cross section of the vessel, it is assumed that the vessel is cylindrical in shape and therefore the vessel cross section is a circle. By measuring the diameter of the vessel, one can calculate the area using the following formula:
Blood flow can be calculated using either of these two formulas:
Velocity time integral (VTI) is the area under the curve of the Doppler envelope for each cardiac cycle. Mean velocity is the time-averaged velocity over the entire cardiac cycle (see below).
Important: The assumption that the vessel in cross section is circular is thought to be acceptable for clinical use of flow estimation in the arterial system. However, for the venous system (such as the superior vena cava, due to collapsibility of the veins), this assumption is more problematic. This will be discussed below.
In this chapter, estimation of left and right ventricular output and superior vena cava flow will be discussed. This will be followed by a brief description of mitral and tricuspid flow Doppler. Use of mitral and tricuspid flow Doppler in the assessment of diastolic function will be discussed in Chapter 8. Finally, the assessment of organ blood flow using Doppler flow indices will be reviewed.
When the ductus arteriosus is closed, left ventricular output (LVO) represents systemic blood flow. Conversely, in the presence of a patent ductus arteriosus (PDA), the systemic blood flow can be over- or underestimated by LVO, depending upon whether the shunt through the PDA is predominantly left to right (overestimate) or right to left (underestimate). While there are different techniques (eg, thermodilution and magnetic resonance imaging) available to estimate cardiac output, echocardiography is the only noninvasive bedside modality that can be used clinically. Recent reports suggest that electrical velocimetry, which is based on thoracic bioimpedance, has the potential for noninvasive and continuous cardiac output monitoring.1–3 As for echocardiography, given the percentage error of up to 30%, except for the extreme values, the absolute LVO should be used with caution in assessing the systemic blood flow. Rather, serial measurements used to assess changes in LVO would be a more reliable indicator of the hemodynamics status.
The normal value of LVO varies in different studies, with mean values ranging from 100 ml/kg/min to as high as 300 ml/kg/min.4–15 There are several reasons for this apparent discrepancy in the literature. The timing of measurement appears to be of significant importance. Immediately after birth, LVO is relatively low and then increases as the pulmonary vascular resistance drops and the left-to-right shunt through the PDA increases.8,15 Subsequently, the LVO decreases with the closure of ductus arteriosus8,15 and remains stable or mildly increases during the first year.6,10,13 Moreover, there is more variability in estimated LVO in preterm infants, especially during the early postnatal period.9,12 Another reason for variability of LVO is the variation in methodology. This is especially true for the measurement of aortic diameter: the measured diameter is smallest when the aortic annulus is measured at the valve hinge points and progressively increases when ascending aorta and sinus of Valsalva are used. Furthermore, the potential to overestimate the aortic diameter is greater when M-mode rather than two-dimensional (2D) ultrasound is used. For practical purposes, mean LVO is considered to be about 200 ml/kg/min with a range of 150–300 ml/kg/min.4–15
The patient should be placed in a supine or a slight left lateral decubitus position.
A high-frequency (10–12 MHz) probe is preferable to maximize spatial resolution. However, in some cases (eg, in larger infants or when a subcostal approach is used), a lower-frequency probe can be used for better penetration (see details on transducer frequency in Chapters 1 and 2).
The apical five-chamber view is usually the best way to ensure a good angle of insonation (<20°) (Figure and Video 7-1). Alternatively, the suprasternal view can be used, especially if flow from the ascending aorta is assessed. When significant lung disease such as air leak or fibrosis is present, the apical and suprasternal acoustic windows might not be available. In such cases, the subcostal view can be helpful, although the angle of insonation across the aortic valve might not be optimal.
For the apical view, place the transducer slightly lateral to the left midclavicular line at the level of 6th or 7th intercostal space (also see the standard views in Chapter 3). The transducer’s marker should be pointing toward the patient’s left. Once a 2D image of the left ventricular outflow tract is obtained, place the PW Doppler cursor at the level of aortic valve to obtain the velocity profile (Figure 7-2). Next, trace the spectral Doppler envelope to determine the VTI (Figure 7-2). It is preferable to use the machine’s analysis system by designating each measurement appropriately (eg, aorta VTI); this will save time because the machine’s software package will perform the necessary calculations. Depending upon the echocardiography system, the heart rate might be automatically acquired through the EKG recording obtained via the bedside monitor, or by manual placement of the leads. Otherwise, the heart rate can be calculated by measuring the time between identical points on the EKG or flow Doppler envelope (RR interval on most echo system).
The patient should be placed in a supine position.
A high-frequency (10-12 MHz) probe is preferable to maximize spatial resolution. However, in some cases (eg, in larger infants or when a subcostal approach is used) a lower-frequency probe can be used for better penetration (see details on transducer frequency in Chapters 1 and 2).
The parasternal long-axis view is the preferred approach for measuring the aortic valve diameter (Figure and Video 7-3).
Place the transducer slightly medial to the left midclavicular line at the level of the 4th or 5th intercostal space (also see the standard views in Chapter 3). The transducer’s marker should be pointing toward the patient’s right shoulder. As the valve structure is less distensible with a change in volume or pressure, measurement of the diameter at the valve is preferable (Figure 7-4). Care must be taken to obtain a standard long-axis view of the heart in order to have the echo reflection of aortic valve cusps (during diastole) in the center. This will ensure that the measured distance is the true valve diameter. The diameter is measured when the valve is open in systole. Alternatively, for the calculation of the surface area, the diameter at the ascending aorta (distal to the sinuses of Valsalva) can be used. This is easier to measure but might be less reliable for serial measurements if there is a significant change in cardiac output. Regardless of whether the valve or ascending aorta is used, it is more important that the diameter and Doppler measurements are performed in the same location.
As mentioned above, in the presence of a PDA, LVO does not represent systemic blood flow. This poses a significant limitation on utility of LVO measurements during the transitional period when a PDA is common. In such circumstances, estimation of right ventricular output (RVO) can be useful in the assessment of systemic flow. In the absence of significant flow through the patent foramen ovale (PFO), blood returning to the right side of the heart and flowing through the pulmonary artery should be equal to the systemic output. Although PFO is a common finding during the transition, usually the flow is not significant. However, it is important to note that in general, the estimated RVO (when measured noninvasively) is higher than LVO.9,11,13,15,16 This is in contradistinction to the fact that the circulation of the neonate is in series, thus LVO and RVO should be equal. The likely reasons for this apparent discrepancy are the difficulty in visualizing the pulmonary valve hinge points (producing an error in measurement of the diameter) and the presence of a PFO. The difference between LVO and RVO in most studies is about 20-50 ml/kg/min,9,15,16 but some authors reported a difference as high as 120-130 ml/kg/min.11,13 In addition, the difference appears to be greater in preterm infants. Therefore, similar to LVO, serial measurements would be a more reliable indicator of a patient’s hemodynamics status than a single RVO measurement.
The patient should be placed in a supine position.
Same as for LVO measurement.
Pulmonary artery flow velocity can be assessed from the modified apical (Figure and Video 7-5), modified parasternal long-axis (Figure and Video 7-6), parasternal short-axis (Figure and Video 7-7), and subcostal views. When significant lung disease exists (such as an air leak or fibrosis) that renders other views impossible, the subcostal view might be the only option.
FIGURE and VIDEO 7-5.
Pulmonary artery flow velocity can be assessed from different views. This video clip shows visualization of the pulmonary artery from the modified apical view. As both aortic and pulmonary flow can be assessed from the apical view, this view is preferable for hemodynamic assessment. The close temporal proximity of acquisition of the two flow Doppler tracings from this view along with minimal disturbance of the patient can be advantageous compared to assessment of aortic and pulmonary flow from separate echo windows and views.

When the apical view is used to acquire a Doppler tracing at the aortic valve, the view can be modified to acquire pulmonary flow Doppler tracing as well. In this approach, after obtaining the aortic Doppler tracing, slide the transducer horizontally toward the midline by 1-2 cm, rotate it clockwise slightly, and tilt it anteriorly to visualize the right ventricular outflow tract, pulmonary valve, and pulmonary artery. The Doppler cursor is placed at the pulmonary valve and a Doppler tracing is obtained (Figure 7-8). For the modified parasternal long-axis view, place the transducer slightly medial to the left mid clavicular line at the level of the 4th intercostal space (also see the standard views in Chapter 3). The transducer’s marker should be pointing toward the right shoulder. This should reveal standard long axis of the heart. Rotating the probe slightly clockwise and tilting it anteriorly will yield the pulmonary valve and artery. Place the PW Doppler cursor at the level of pulmonary valve to obtain the velocity profile. Tracing the spectral Doppler envelope and determining the heart rate are performed in the same manner as for LVO measurement (see above).
The patient should be placed in a supine position.
Same as for LVO measurement.
All the above four views can be used to measure the diameter. The preferred method is the parasternal long-axis view.
The view and the procedure are the same as the ones used for measuring the velocity. The pulmonary valve annulus should be measured during systole. However, since the plane of pulmonary valve attachment is parallel to the transthoracic ultrasound beam, the cusps attachments are poorly visualized in all the views (Figure 7-9). This makes estimation of valve diameter less reliable compared to the aortic valve. Alternatively, the main pulmonary artery (pulmonary trunk) diameter distal to the sinus of Valsalva can be measured (Figure 7-10). However, using this diameter to calculate the RVO often overestimates the actual output. In addition, the repeatability of this measurement is worse than that of pulmonary valve.16
As mentioned above, application of LVO and RVO in assessing systemic flow can be limited by the presence of a significant PDA and PFO, respectively. Kluckow and Evans proposed SVC flow measurement as a surrogate for cerebral and systemic blood flow.17 There are several advantages of SVC flow over ventricular output. SVC flow measurement is not affected by a PDA or PFO. As regional blood flow, in general, and cerebral blood flow, in particular, is of more interest than the entire systemic cardiac output during the early postnatal transition, SVC flow measurement is advantageous. However, SVC flow measurement also has its own set of limitations. Given the collapsibility of the vein, the assumption of a circular cross section (as used for an artery) is not valid. The contribution of brain blood flow to SVC flow in the preterm infant is unknown. Nevertheless, SVC flow, when used in conjunction with other indicators of systemic flow or in a research setting, can be useful. Table 7-1 shows the range of normal SVC flows in preterm and full-term infants.11,17,18 In the very preterm infant, SVC flow <30–45 mL/kg/min is considered low during the first postnatal day and the low SVC flow is associated with increased risk for development of intraventricular hemorrhage and poor neurodevelopmental outcome.19–22
Authors | n | VTI | max D | min D | Flow | |
5 hr | ||||||
Kluckow & Evans17 | 25 | 9.7 (6.3–13.0) | 3.0 (2.1–4.5) | 2.4 (1.6–3.5) | 62 (30–140) | |
Groves et al11 | 80 | 10.0 (5.5–12.2) | 90 (41–132) | |||
12 hr | ||||||
Kluckow & Evans17 | 25 | 9.3 (5.4–15.5) | 3.4 (2.0–4.1) | 2.6 (1.6–3.6) | 75 (34–117) | |
Groves et al11 | 80 | 9.0 (5.0–15.4) | 101 (40–183) | |||
Holberton et al18 | 165 | 114 (82, 150)a | ||||
24 hr | ||||||
Kluckow & Evans17 | 24 | 11.0 (4.3–17.6) | 3.5 (2.4–4.3) | 2.5 (1.6–3.3) | 82 (42–150) | |
Groves et al11 | 80 | 11.0 (7.6–16.6) | 112 (64–193) | |||
48 hr | ||||||
Kluckow & Evans17 | 21 | 10.6 (5.7–17.2) | 3.5 (2.4–4.3) | 2.5 (1.6–3.3) | 86 (46–140) | |
Groves et al11 | 80 | 12.6 (8.0–17.3) | 113 (82–179) |
The patient should be placed in a supine position.
Same as for LVO measurement.
Subcostal (Figure and Video 7-11)
Place the transducer on the abdomen between the xiphoid process and the umbilicus (also see the standard views in Chapter 3). With the marker pointing toward the patient’s left, gently tilt the transducer posteriorly. After visualization of the right atrium in 2D, the SVC can be identified using color Doppler. Place the PW Doppler cursor in the SVC as it joins the right atrium to obtain the velocity profile. Identify the typical triphasic flow pattern (Figure 7-12). Tracing the flow Doppler envelope and determining the heart rate is done in the same manner as for LVO (see above). It is important to trace at least 3-5 cardiac cycles to capture the variation in Doppler envelopes, which at times can be quite significant, as shown in Figure 7-13. Simultaneous acquisition of the EKG tracing helps in identifying the cardiac cycle when the determination of the cardiac cycle by flow pattern is not feasible (Figure 7-13D).
The patient should be placed in a supine position.
Same as for LVO measurement
SVC diameter can be measured in modified suprasternal or parasternal long-axis view (Figure and Video 7-14).
In suprasternal view, first the brachiocephalic (innominate) vein is identified by counterclockwise rotation, and moving the transducer downward using color Doppler, the vein can be traced as it drains to SVC and right atrium (also see the standard views in Chapter 3). The diameter can be measured using 2D or M-mode. Due to significant diameter change over the cardiac cycle, for flow measurement, the average of maximum and minimum diameter is used. The change in diameter over the cardiac cycle is better visualized by the M-mode than 2D. However, placing the M-mode line perpendicular to the SVC can be challenging. An oblique section of SVC by the M-mode leads to overestimation of cross-sectional area and hence overestimation of SVC flow. Therefore, the 2D measurement of diameter is generally preferable (Figure 7-15).
FIGURE 7-15.
Two-dimensional and color Doppler images of superior vena cava (SVC) obtained from high parasternal long-axis view (A and B). Measurement of SVC diameter is shown by the double arrow. The average of maximum (A) and minimum (B) diameters is used for calculation of SVC flow. Abbreviation: RA, right atrium.

Estimation of blood flow through mitral and tricuspid valves is inaccurate due to the inability of measuring accurately the surface area of the valves. PW Doppler is used to evaluate the flow pattern rather than the flow. The characteristics of flow pattern can be useful for assessment of diastolic function, which is discussed in detail in Chapter 8.
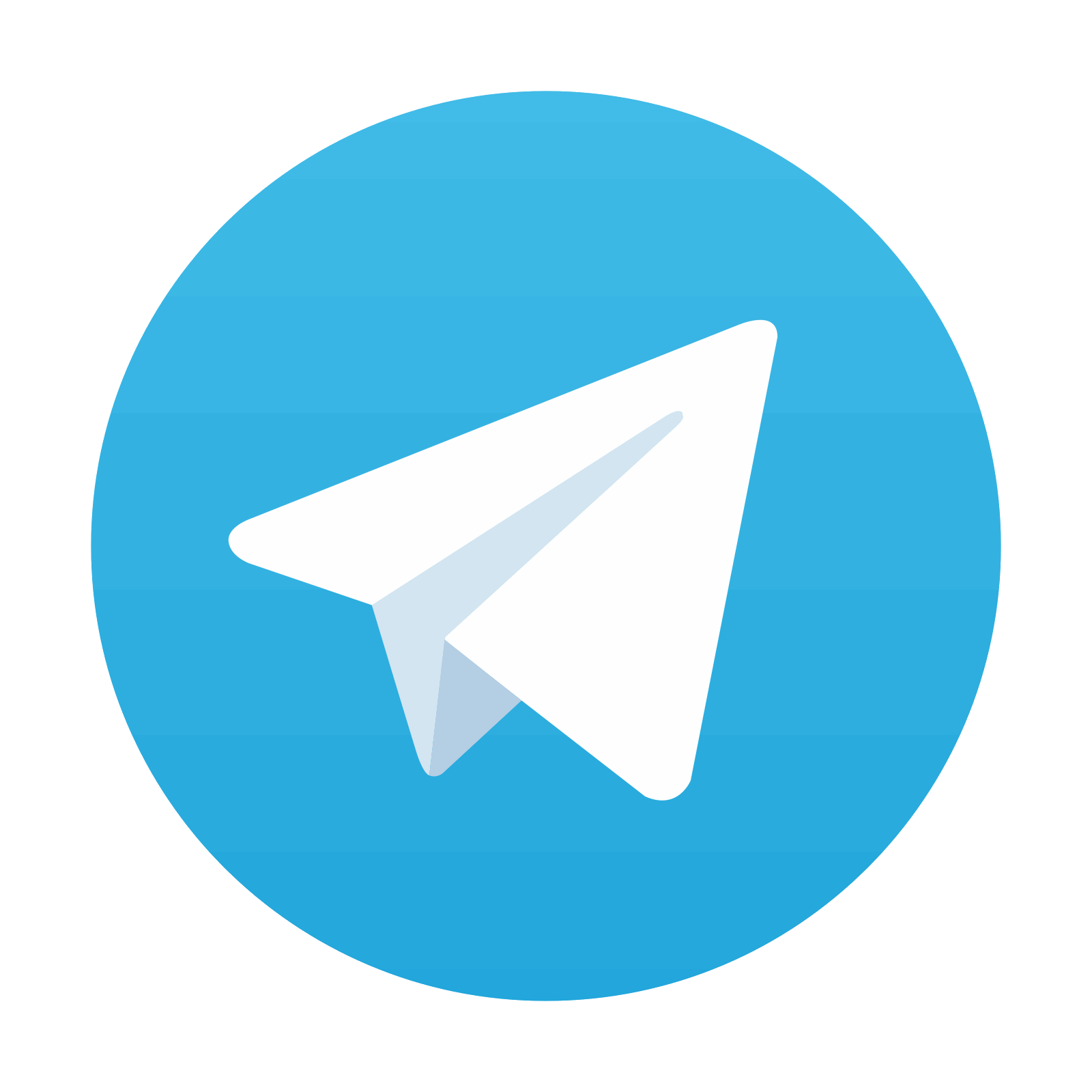
Stay updated, free articles. Join our Telegram channel

Full access? Get Clinical Tree
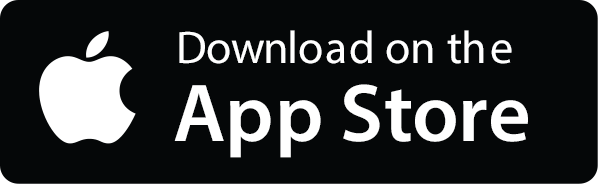
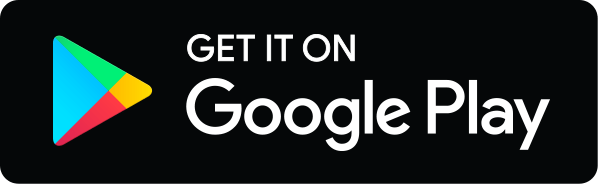