Introduction to Aortic Regurgitation
- Issam A. Mikati, MD
- Robert O. Bonow, MD, MS
Aortic regurgitation (AR) is a common form of valvular heart disease. It frequently develops in patients with bicuspid aortic valves as well as patients with conditions that develop from degenerative processes related to aging or diseases of the aortic root and ascending aorta. AR places both a pressure and volume overload on the left ventricle. Natural history studies have shown that this results in left ventricular (LV) dilation and, ultimately, systolic dysfunction, which is transiently reversible but then becomes permanent. Because medical treatment with vasodilator therapy has met with mixed and limited success, surgical aortic valve replacement (AVR) remains the therapeutic option of choice despite the lack of randomized prospective studies demonstrating its efficacy. Aortic valve repair is only an option for a limited number of patients. Therefore the majority of patients will require AVR. There are no clinically established transcatheter valve–based options at this time, although they are actively being researched.
The fundamental issues in managing patients with AR include (1) identifying those with bicuspid aortic valves (often with an associated aortic aortopathy), (2) determining the mechanism responsible for the regurgitation (which is often not a primary valve pathology but instead disease of the aortic root and ascending aorta), (3) quantifying the severity of regurgitant flow to identify those with severe AR, (4) assessing LV systolic function and the degree of LV dilation, and (5) determining the optimal time for surgical intervention in those with severe AR. Patients with severe AR may remain asymptomatic for decades before the onset of symptoms, but when the symptoms are the only indication for surgical intervention, an important subset will have developed irreversible LV dysfunction that puts them at risk for postoperative heart failure (HF) and death despite a technically successful AVR. Early surgery prevents development of LV dysfunction; however, an aggressive early preemptive referral strategy, especially in young patients, places them at risk of long-term complications from prosthetic heart valves. Therefore the timing of surgery is a combination of the art and science of cardiovascular medicine: the goal is to send patients to surgery early enough to prevent irreversible LV dysfunction and late enough that patients derive long-term symptomatic and survival benefit from the procedure.
Cardiovascular imaging at each of the five steps mentioned previously is essential in managing patients with AR. Although a physical examination is important in the initial detection of patients with AR, it is inferior to Doppler echocardiography in determining its severity, its impact on LV function, and possible concomitant diseases of the aorta. The physical examination is also not effective for serial monitoring and detection of early LV dysfunction. Compounding this problem is the well-documented erosion in physical examination skills.
The ideal imaging test would provide the aortic valve anatomy and the ascending aortic morphology accurately, reproducibly, and efficiently. A classification scheme has been proposed based on mechanism of aortic regurgitation. This would help guide surgical treatment while also identifying patients who are candidates for repair versus replacement.
The ideal imaging test would also quantify the severity of AR and provide accurate measurements of LV size and function because progressive LV dilation or declining systolic function has been identified as a trigger for surgery. These measurements need to be accurate and reproducible, with little inter- and intraobserver variability. The issue of reproducibility is essential because patients will need to be followed over time, and comparison with previous data is key in making treatment decisions in patients with AR. Currently, these tests are often performed at different centers and interpreted by different readers.
Echocardiography is recognized as the primary imaging test for AR assessment because it meets many of the criteria of an ideal imaging test mentioned previously. In addition, it is noninvasive, and it is widely availability and relatively inexpensive.
Echocardiography, with its excellent spatial and temporal resolution, allows visualization of the necessary anatomic information and quantification of the severity of AR and its impact on LV function. Both quantitative and qualitative measures are used, and an integrated approach incorporating many parameters is recommended because reliance on any single measure may lead to errors in interpretation. Assessment of the LV chamber was initially performed using 1D M-mode measurements, and much of the natural history data are based on this methodology. However, such M-mode data may be less reliable than measurements guided by 2D imaging or quantification of LV volumes. Cutoff values for end-diastolic dimensions, end-systolic dimensions, and systolic function have been defined as potential indications for surgery, and newer thresholds based on LV volumes and regurgitant severity are emerging.
Because of the limitations related to the variability of traditional 2D imaging (inter- and intraobserver variability of 6% in older studies), 3D echocardiography has emerged, as it provides a more accurate assessment of LV volume. Multiple studies, including multicenter studies, have shown less variability in 3D echocardiographic measurements and a closer correlation with the gold standard of cardiac magnetic resonance (CMR) imaging, as discussed in the following. This has helped blunt the consistent underestimation of LV volumes reported with 2D echocardiography and has allowed meaningful serial measurement of LV volumes.
Serial CMR has several potential advantages compared with transthoracic echocardiography, including improved endocardial definition, fewer geometric assumptions, and less angle dependence for flow measurements. This results in better reproducibility of AR severity quantitation. However, CMR and other imaging modalities mainly complement echocardiography, which is the clinical workhorse for AR assessment.
Beyond assessment of LV volumes and ejection fraction (EF), there is a need for early identification of systolic dysfunction so that patients can either be followed closely or referred for intervention before the onset of permanent LV systolic dysfunction. Traditional measures of LV size and systolic function, such as EF, are not sufficiently sensitive. Biomarkers such as brain natriuretic peptide (BNP) have promise in bridging this gap. BNP levels are higher in symptomatic than asymptomatic patients, although there is a significant relationship between BNP levels and severity of LV dilatation in asymptomatic patients. BNP is an independent marker in asymptomatic patients with normal LV systolic function for subsequent symptoms and/or LV dysfunction, even after adjustment for LV volumes.
Advanced imaging methods to assess regional and global systolic function, such as longitudinal strain, may prove to be more sensitive than LVEF in identifying patients with chronic severe AR who are at higher risk. Quantification of myocardial interstitial connective tissue by CMR, which has been shown to have prognostic value in the hypertrophied ventricles of patients with aortic stenosis, may also prove valuable in patients who have combined pressure and volume overload caused by chronic AR. Thus advances in echocardiographic techniques combined with other technologies offer great hope in filling the clinical gaps that persist in the current strategies for management of patients with chronic AR.
Aortic Regurgitation: Etiologies and Left Ventricular Responses
- Nicole M. Bhave, MD
Etiology
Etiologies of aortic regurgitation (AR) fall into two broad categories: primary leaflet problems and abnormalities of the aortic root. Primary leaflet problems include both congenital and acquired abnormalities. The most common congenital cardiac anomaly is bicuspid aortic valve, which is seen in 0.5% to 2% of the population. Bicuspid aortic valves are predisposed to regurgitation and stenosis and are often associated with ascending aortic dilation, which can also contribute to AR. Quadricuspid aortic valves, which are a much rarer anatomic variant, are more commonly associated with AR than stenosis ( Fig. 103.1 and Video 103.1, A ). Acquired valvular lesions, such as degenerative and calcific changes, endocarditis, and rheumatic valvular disease, can also result in AR ( Fig. 103.2 and Video 103.2, A-G).


Dilation and distortion of the aortic annulus, the sinuses of Valsalva, and the sinotubular junction, often collectively called the aortic root, may lead to suboptimal aortic valve leaflet coaptation, resulting in AR. The most common cause of thoracic aortic aneurysm is degenerative change; risk factors include advanced age, hypertension, and smoking. Genetic disorders such as Marfan syndrome, Turner syndrome, and familial thoracic aortic aneurysm syndrome, among others, can cause the sinuses of Valsalva and the ascending aorta to become dilated. Stanford type A aortic dissection can cause AR via multiple mechanisms, including dilation of the aortic root, pressure of a false lumen on one cusp resulting in asymmetrical coaptation, flail caused by disruption of leaflet support at the level of the sinotubular junction and/or annulus, and prolapse of an intimal flap through the annulus ( Figs. 103.3 , A-C , and 103.4 and Video 103.3). Rarely, blunt chest wall trauma can cause a leaflet tear or disruption of leaflet support ( Fig. 103.5 and Video 103.5, A and B ). A more extensive list of AR etiologies is given in Table 103.1 .



General Category | Specific Category | Examples |
---|---|---|
Primary Leaflet Abnormality | Congenital | -Bicuspid valve -Quadricuspid valve -Unicuspid valve |
Degenerative | -Thickening and/or calcification due to aging -Myxomatous degeneration -Leaflet damage due to membranous subaortic stenosis | |
Infectious/inflammatory | -Bacterial endocarditis -Nonbacterial thrombotic endocarditis -Rheumatic valvular disease | |
Neoplastic | Papillary fibroelastoma | |
Traumatic | Iatrogenic (cardiac catheterization) | |
Toxic | Anorexigen exposure | |
Aortic Root Abnormality | Genetic | -Marfan syndrome -Loeys-Dietz syndrome -Ehlers-Danlos syndrome -Turner syndrome -Familial thoracic aortic aneurysm syndrome |
Degenerative | -Advanced age -Hypertension -Atherosclerosis | |
Infectious | -Syphilis -Salmonella -Staphylococci -Mycobacteria | |
Inflammatory | -Giant cell arteritis -Takayasu arteritis -Rheumatoid arthritis -Spondyloarthropathies | |
Traumatic | -Aortic dissection -Leaflet tear or disruption of leaflet support at the root | |
Other | -Aortic dissection (multiple potential mechanisms of AR) -Ventricular septal defect with cusp prolapse |
Left ventricular responses to aortic regurgitation
The response of the left ventricle (LV) to AR depends upon the time of AR onset. In acute, severe AR, the LV experiences a sudden increase in end-diastolic volume but is not compliant enough to dilate rapidly. That is, normal LV size in the presence of severe AR is an indication that the AR is acute. The sudden increase in end-diastolic pressure with acute AR usually results in elevated left atrial pressure and pulmonary edema. Afterload also increases, leading to a decrease in stroke volume; tachycardia develops to maintain sufficient cardiac output. As the LV end-diastolic pressure approaches aortic diastolic and coronary perfusion pressures, myocardial ischemia may result.
Echocardiography is indispensable in establishing the etiology of acute, severe AR. The most common causes are infective endocarditis and Stanford type A aortic dissection. Transthoracic echocardiography (TTE) may be able to distinguish between these entities, but it is often appropriate to proceed to transesophageal echocardiography (TEE) for optimal imaging of all valves and the aorta. Because Stanford type A aortic dissection is a surgical emergency, an expeditious diagnosis is critical, and TEE can rapidly establish the presence of a dissection, define its extent, and clarify the mechanism(s) by which it is causing AR. Echocardiography can also be very helpful in identifying two other features of acute, severe AR: premature mitral valve closure (best appreciated on M-mode echocardiography) and diastolic mitral regurgitation.
In chronic AR, the LV adapts to accommodate the increased end-diastolic volume. Chamber compliance increases, and eccentric and concentric hypertrophy occur so that filling pressures remain normal or near-normal. Chamber dilation allows for preserved stroke volume, even though afterload is also increased. Over time, often years, LV systolic function worsens and patients may begin to experience heart failure symptoms such as dyspnea and lower extremity edema.
Prognosis and guideline-based management of aortic regurgitation
The prognosis of chronic AR is closely associated with the degree and pace of LV remodeling, even in the absence of symptoms. Longitudinal studies have shown that initial end-systolic dimension, rate of increase of end-systolic dimension, LV ejection fraction (EF), and rate of decrease of LVEF are independently predictive of symptom development and mortality. Because asymptomatic patients with significant chronic AR can experience poor outcomes, including sudden death, serial echocardiography is indicated to assess for LV dilation and dysfunction. Other indications for echocardiography in patients with AR are listed in Box 103.1 .
To confirm the presence and severity of AR (acute or chronic)
To diagnose and assess the etiology of AR
To assess aortic root dimensions and AR severity in patients with aortic root dilation
To assess left ventricular dimensions and systolic function in chronic AR upon initial diagnosis
To reassess left ventricular dimensions and systolic function periodically in asymptomatic patients with chronic, severe AR
To reevaluate mild, moderate, or severe AR in patients with new or changing symptoms
AR, Aortic regurgitation.
All indications listed are Class I (i.e., there is evidence and/or general agreement upon the benefit or usefulness of echocardiography in these settings).
The definitive treatment of severe AR is generally surgery. Optimal timing of surgery may be determined by clinical assessment of the patient and echocardiographic evaluation of LV dimensions and systolic function. Indications for aortic valve surgery in AR are listed in Table 103.2 . The left ventricular end-diastolic diameter and end-diastolic volume are expected to decrease in the immediate postoperative period. The EF tends to increase postoperatively in patients with preexisting LV systolic dysfunction, but in those with normal systolic function, it may decrease following surgery because of decreased preload. Patients with preoperative LV systolic dysfunction may have continued improvement in the EF in the months to years following aortic valve surgery. The degree of increase in EF correlates with the decrease in end-diastolic diameter. Patients with severe preoperative symptoms or reduced exercise tolerance and those with severe or long-standing LV dysfunction tend to have higher operative mortality and worse recovery of LV systolic function.
Class I | Severe AR with symptoms |
Severe AR without symptoms, with LV systolic dysfunction (ejection fraction ≤ 50% at rest) | |
Severe AR in patients undergoing coronary artery bypass grafting, surgery on the aorta, or other valve surgery | |
Class IIa | Severe AR with normal LV systolic function but severe LV dilation (end-diastolic dimension > 75 mm or end-systolic dimension > 55 mm) |
Class IIb | Moderate AR in patients undergoing coronary artery bypass grafting or surgery on the ascending aorta |
Severe AR in asymptomatic patients with normal LV systolic function and any of the following: -LV end-diastolic diameter > 70 mm -LV end-systolic diameter > 50 mm -Progressive LV dilation -Declining exercise tolerance -Abnormal hemodynamic response to exercise |
Echocardiography is an invaluable tool for establishing the presence and etiology of AR and its effects on the left ventricle. A combination of transthoracic and transesophageal imaging is often warranted for accurate assessment of LV size and systolic function and optimal visualization of the aortic valve. Patients with AR often require serial echocardiograms to reevaluate the ventricle, as LV remodeling is associated with adverse clinical consequences.
Aortic Regurgitation: Pathophysiology
- Roy Beigel, MD
- Robert J. Siegel, MD
Aortic regurgitation pathophysiology
The pathophysiology of acute and chronic aortic regurgitation (AR) is quite different. The pathophysiology and hemodynamic presentation differs whether AR develops abruptly (acute AR) or over a prolonged time period (chronic AR). Table 104.1 lists the main differences between these two distinct processes.
Acute AR | Chronic AR | |
---|---|---|
Clinical presentation | Pulmonary edema, refractory heart failure | Often asymptomatic |
Left ventricular size | Normal to slightly enlarged | Markedly enlarged |
Left ventricular end-diastolic pressure | Markedly elevated | Normal to slightly elevated |
Systolic aortic pressure | Normal or slightly decreased | Elevated |
Diastolic aortic pressure | Normal or slightly decreased | Decreased |
Pulse pressure | Normal or slightly increased | Increased |
Cardiac output | Decreased | Normal |
Heart rate | Elevated | Normal or slightly elevated |
Acute aortic regurgitation
Acute severe AR is characterized by the abrupt development of severe left ventricular (LV) volume overload in a ventricle, which does not have time to adapt to this acute change. The LV is often normal in size and is consequently exposed to a very high preload as well as an excessive afterload (due to the combination of elevated blood pressure and increased LV wall stress). The excessive load on the left ventricle can cause depression in LV ejection fraction (LVEF) and then a drop in LV stroke volume despite a relatively maintained myocardial contractility. The rapid increase in the regurgitant flow into the LV results in a hemodynamically overloaded LV with an acute rise in the LV end-diastolic pressure as the LV volume is somewhat fixed. This results in a rapid and marked increase not only of the LV end-diastolic pressure but also of the left atrial end-diastolic pressure and pulmonary capillary wedge pressure. This acute volume load causes the ventricle to operate at the extreme of the pressure-volume curve ( Fig. 104.1 ). The development of reflex tachycardia helps maintain cardiac output. In severe cases, the LV end-diastolic pressure can exceed the left atrial pressure and cause premature, presystolic closure of the mitral valve with or without diastolic mitral regurgitation ( Fig. 104.2 ). Thus, patients often present with pulmonary edema and even cardiogenic shock. In patients with preexisting LV hypertrophy (due to pressure overload such as in hypertension and aortic stenosis), acute AR can cause “exaggerated” hemodynamic changes, as these patients often have a small LV cavity, concentric hypertrophy, and a noncompliant left ventricle with a reduced preload reserve. Further deterioration accompanied by depression in the LV function and stroke volume can occur when the LV end-diastolic pressure approaches the aortic diastolic pressure, causing a drop in the gradient from aorta to LV end-diastolic pressure (myocardial perfusion pressure). This may cause subendocardial hypoperfusion and signs and symptoms of myocardial ischemia. With distention and dilation of the left ventricle, there is distention/dilation of the mitral annulus, which can induce functional mitral regurgitation (MR), which then further elevates the left atrial and pulmonary capillary wedge pressures.


Chronic aortic regurgitation
Failure of adequate closure of the aortic cusps causes AR. Factors that affect regurgitant volume are mainly the effective regurgitant orifice and, to a lesser extent, the diastolic pressure gradient between the aorta and the LV. Bradycardia, by prolonging diastole, also increases the regurgitant load. As AR progresses over time, the LV end-diastolic volume rises. It may eventually attain an LV volume at which the preload reserve is reached, meaning that all the sarcomeres within the LV are maximally distended such that any further increase in the LV afterload results in a decreased LV stroke volume. Table 104.2 summarizes the differences in the pathophysiologic features affecting the LV during the chronic phase of aortic and mitral regurgitation. Both AR and MR are volume overload conditions. However, in MR the regurgitant stroke volume flows retrograde into the low-pressure left atrial chamber. Conversely, in AR the sum of the regurgitant volume and the forward stroke volume is ejected forward into the high-pressure aorta during systole. The increase in the ejected forward stroke volume causes systolic hypertension (increased afterload) along with a wide pulse pressure caused by the decrease in diastolic pressure due to a drop in the aortic diastolic pressure. Although MR and AR are both associated with mechanical overload of the left ventricle, the systolic wall stress is higher in patients with AR compared to MR. In fact, wall stress may reach a level similar to that found in patients with aortic stenosis. Systolic wall stress is expressed by the Laplace relation p × r /2 h ( p , LV pressure; r , LV radius; h , wall thickness). In patients with AR, both p and r are increased, and although h is increased as well, it is generally not enough to compensate for the combined increase of both p and r . Consequently, AR increases the volume-pumping requirements of the LV (preload) and it also results in a significant increase in systolic wall stress and LV afterload.
Aortic Regurgitation (AR) | Mitral Regurgitation (MR) | |
---|---|---|
Pathophysiological mechanism | Volume and pressure overload | Volume overload |
LV afterload | Increased | Decreased |
LV wall stress | Higher than MR | Lower than AR |
LV geometry | Eccentric hypertrophy with modest concentric hypertrophy | Enlarged LV |
LV mass to volume ratio | 1 | < 1 |
LV ejection fraction (EF) | Can be lower before myocyte damage and contractile dysfunction have developed | Greater than normal—in compensated phase Normal or lower—in decompensated phase or when contractile dysfunction has developed |
Mechanism of reduced LV contractility | Increased afterload | A reduction in EF signifies loss of contractile elements |
Mechanism of LV hypertrophy | Increase in protein synthesis | Reduced rate of protein degradation |
LV radius to thickness | Lower than MR | Higher than AR |
Reversibility with treatment | Mostly reversible | Mostly reversible |
The volume overload accompanying chronic AR causes a series of pathophysiologic events, which include an initial increase in LV end-diastolic volume and pressure that leads to increasing LV compliance, accommodating the increased volume with optimizing filling pressures (see Fig. 104.1; Fig. 104.3 ). The chronic LV volume overload results in chamber enlargement, an eccentric pattern of hypertrophy, and an increase in LV mass that permits the ventricle to eject a large stroke volume, increasing the systolic pressure (increased afterload) to maintain a normal forward stroke volume.

In the early phase of chronic AR, compensatory mechanisms keep LVEF and LV fractional shortening in the normal range. Over time, however, the LV dilates and hypertrophies, and the ratio of ventricular wall thickness to cavity radius is maintained. As a consequence, the LV end-diastolic wall stress remains normal. These adaptive mechanisms permit the LV to accommodate large stroke volumes, often with little increase in LV filling pressure. Patients with severe AR have the largest LV end-diastolic volumes that occur in patients with heart failure—also termed cor bovinum ( Fig. 104.4 ). In AR patients, LV end-diastolic volumes can be three or four times normal, which allows these patients to sustain high cardiac outputs. LV compliance is usually also increased, so that the LV end-diastolic pressure (LVEDP) may be only slightly elevated. These compensatory mechanisms allow patients to remain stable for years even in the presence of severe AR. However, this is a progressive condition, and the increased afterload results in an increase in end-systolic dimensions and eventually a reduction in LVEF. The LVEF is low normal in AR, and the end-systolic dimensions are higher when compared with MR patients in whom the LVEF is generally preserved or is even on the higher side. In chronic AR, if wall thickening fails to keep up with the volume overload, there is an increase in wall stress, which then further reduces LV systolic function and the LVEF associated with contractile dysfunction due to myocyte damage. As LV filling pressure rises, symptoms of fatigue and dyspnea appear. Angina develops even in the presence of normal coronary arteries because of the combination of reduced coronary blood flow reserve in the hypertrophied myocardium, lower than normal diastolic pressures, shorter diastolic time (due to increasing heart rate), and elevated LVEDP, which results in a decrease in the gradient (aortic diastolic pressure–LVEDP) for coronary blood flow and myocardial perfusion. These further contribute to progressive deterioration in LV function. Distention and dilation of the left ventricle can also lead to secondary (functional) MR. Subsequently, cardiac output falls and the LV end-diastolic pressure and volume rise. All these contribute to elevation of the left atrial and pulmonary artery wedge pressures, which can cause pulmonary edema and an increase in the pulmonary artery, right ventricular, and right atrial pressures, eventually compromising right ventricular function.

Quantitation of Aortic Regurgitation
- Hari P. Chaliki, MD
- Vuyisile T. Nkomo, MD, MPH
Aortic regurgitation (AR), either acute or chronic, can be due to valvular pathology or aortic root pathology or a combination. In developed countries, chronic isolated AR is predominantly due to aortic root disease, whereas rheumatic fever is still the leading cause of chronic AR in developing countries. Surgery is frequently needed for patients with acute AR, whereas the timing of surgery for chronic AR depends on the severity of the regurgitation, the patient’s symptoms, and left ventricular size and function. Therefore, accurate determination of etiology and severity of AR is crucial when managing patients with AR. Semiquantitative and quantitative techniques should be used in an integrated fashion to assess the severity of AR.
Quantitation of aortic regurgitation
Semiquantitative Methods
Currently, Doppler color flow imaging is widely used to determine the severity of aortic regurgitation despite its limitations and semiquantitative nature. Parasternal long-axis, short-axis, and apical views can nicely demonstrate AR via color Doppler recording. Maximal length and area of the aortic regurgitant jet correlate poorly with aortic angiography. Short-axis jet area at the high left ventricular outflow tract (LVOT) relative to the LVOT area correlates well with angiography. Proportion of either jet area or jet width compared with LVOT area or width in excess of 60% to 65% indicates severe AR. Although this method is appealing, note that it requires careful attention to gain settings and Nyquist limits to avoid over- or underestimating the severity of regurgitation. In general, this method only gives a rough estimate of the severity of AR. This method is of limited utility in patients with eccentric jets, diffuse jets, and jets originating along the entire coaptation line.
Both continuous and pulse wave Doppler techniques are useful for the semiquantitative assessment of AR. Dense continuous-wave Doppler signal implies greater quantity of regurgitation, whereas faint signals generally represent mild regurgitation. Pressure half-time, the time for the pressure gradient to drop by half of its original value, is another measure of severity of AR obtained by continuous-wave Doppler. It is expected that the pressure half-time will be shorter (< 200 msec) in patients with severe AR because of rapid rise in left ventricular pressure from a large amount of regurgitant volume, especially in patients with acute AR. Conversely, in patients with mild AR pressure, half-time is longer (> 500 msec) because of a more gradual rise in left ventricular pressure. Pressure half-time cannot be used alone to determine the severity of AR because it is dependent on chronicity of the regurgitation, systolic blood pressure, and left ventricular compliance. For example, someone may have a short pressure half-time because of underlying severe left ventricular diastolic dysfunction. Another frequently used Doppler technique involves pulse-wave Doppler of the proximal descending thoracic aorta. Significant holodiastolic flow reversals in the descending thoracic aorta, diastolic velocity time integral similar to systolic velocity time integral, and relatively high end-diastolic velocity (> 20 cm/sec) are indicative of moderate to severe AR ( Fig. 105.1 and Video 105.1). In some older patients, diastolic flow reversal is a less reliable indicator of the severity of AR because of a stiff aorta.

It has been proposed that hydrodynamically the smallest area of the aortic regurgitant jet represents vena contracta (VC). From parasternal views, using the zoom function, one can visualize the proximal flow convergence zone, flow at the aortic valve, and flow below the aortic valve. In early to mid-diastole, VC is then measured, defined as the width of the narrowest portion of the aortic regurgitant jet, which occurs immediately distal to the anatomic orifice. VC width greater than 6 mm is indicative of severe AR with a sensitivity of 81% and specificity of 94%. In some patients, a right parasternal transthoracic window or transesophageal echocardiography (TEE) may be required to obtain adequate VC measurement ( Fig. 105.2 and Video 105.2).

Quantitative Methods
Continuity method utilizes the principle of conservation of mass for determination of aortic regurgitation. Calculation of the regurgitant volume is based on the calculation of the stroke volume through the aortic valve and the stroke volume throughout a second and competent valve (e.g., the mitral valve). Assuming there is no or minimal mitral regurgitation, the volume of AR can be precisely calculated by subtracting the flow through the mitral valve from the LVOT flow. This method uses the combination of two-dimensional (2D) information and Doppler technique. Flow through the mitral annulus and LVOT can be calculated by multiplying mitral annular area and LVOT areas with their corresponding time-velocity integrals (TVI). Assuming a circular mitral annulus and LVOT, the following formulas are used to determine the mitral flow, LVOT flow, and aortic regurgitant volume (RVol) :
Mitral flow = Mitral annulus area × TVI at the mitral annulus level *
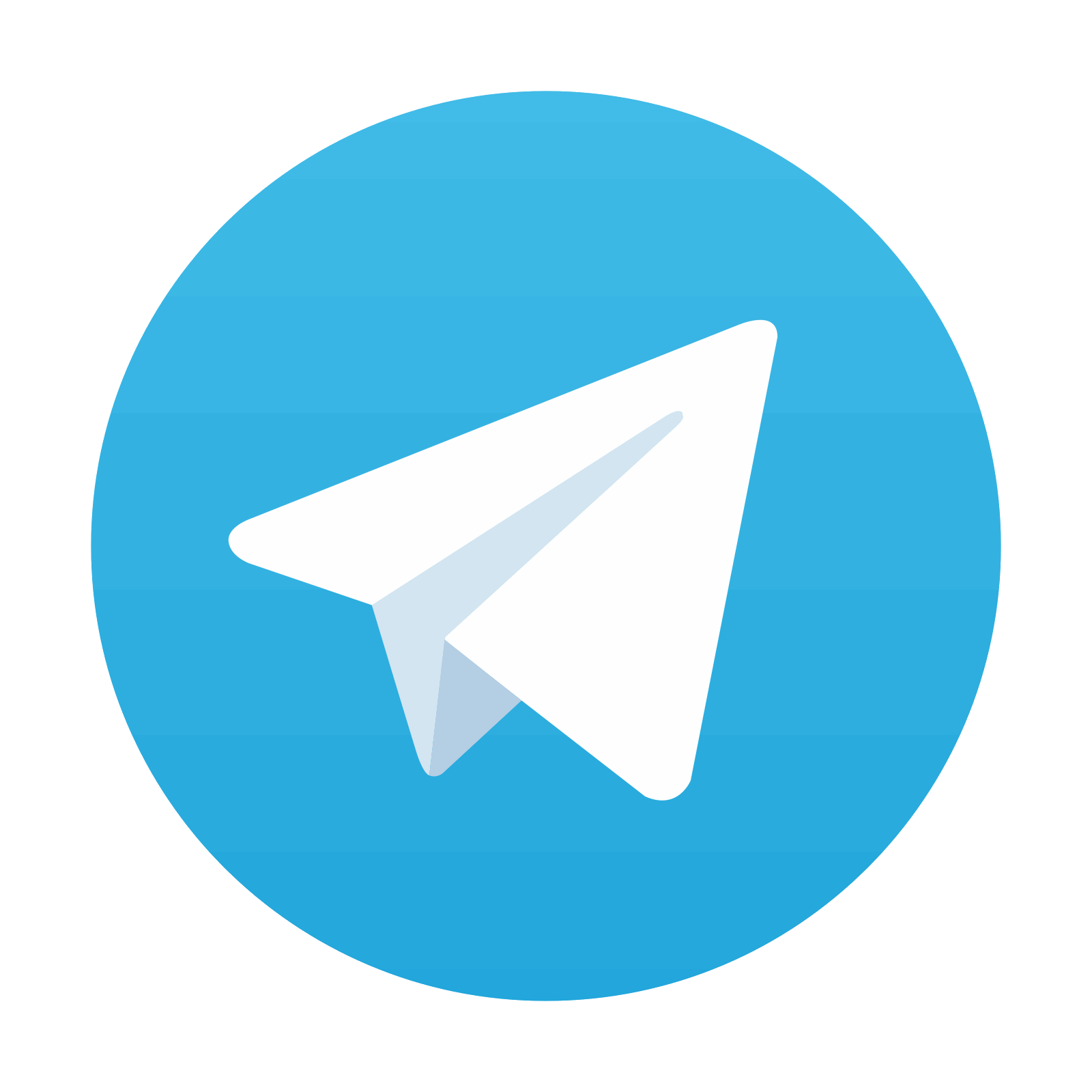
Stay updated, free articles. Join our Telegram channel

Full access? Get Clinical Tree
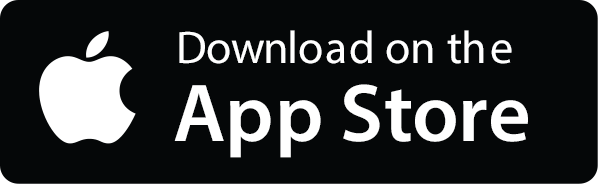
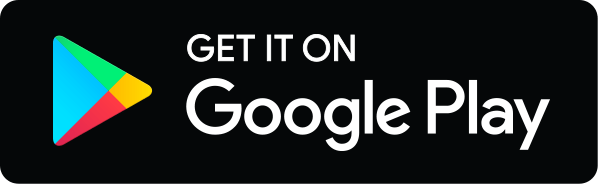