Chapter 1
- 1.
The alveolar–capillary unit has a large surface area for gas exchange. In addition, there is a short distance between the alveolus and the red blood cell, and the unit as a whole takes the entire cardiac output. These features make it ideal to function as the gas-exchanging unit.
- 2.
Type II cells cover almost the entire alveolar surface (93%) with their broad, thin extensions. They do not divide and are especially susceptible to injury. Type II cells are cuboidal and located in the corners of alveoli. They are more numerous than type I cells. The hallmark of type I cells are the osmiophilic lamellar inclusion bodies that contain surfactant. Upon injury, type II cells multiply and eventually become type I cells.
- 3.
The pulmonary circulation is a low-pressure, high-compliance system. At rest, many of the vessels are either compressed or not open. Increases in pulmonary flow either universally, as would occur during exercise, or locally, as would occur if one of the main pulmonary arteries were occluded, are associated with maintenance of low pressure, as compressed or occluded vessels open and open vessels distend. Thus transient occlusion of the left main pulmonary artery would double the blood flow through the right pulmonary artery, but this increase in flow would produce only a small change in the pressure in the right pulmonary artery and would certainly not double it.
- 4.
The barrier between gas in the alveolus and the red blood cell consists of the type I alveolar epithelial cell, the capillary endothelial cell, and their respective basement membranes. The distance of the barrier is approximately 1 to 2 µm.
- 5.
The principal function of the lung is gas exchange. The anatomic features that make the lung ideally suited for this function include the large surface area for gas exchange, with millions of alveoli and capillaries; the close proximity between gas in the alveolus and the red blood cell; the dual circulation of the lung, resulting in the entire cardiac output going through the lung; the structure of the type I cell, with its thin, elongated cytoplasm that reduces the distance between alveolar gas and the pulmonary capillary; and the highly distensible, low-pressure pulmonary circulation that allows for accommodation over a wide range of cardiac outputs without a significant change in pressure.
Chapter 2
- 1.
Factors that determine total lung capacity (TLC) include lung elastic recoil and the properties of the muscles of the chest wall. TLC occurs when the forces of inspiration decrease because of chest wall muscle lengthening and are insufficient to overcome the lung’s elastic recoil.
- 2.
Residual volume occurs when the forces exerted by expiratory muscle shortening decrease and are insufficient to overcome the outward recoil of the chest wall.
- 3.
Acute hypersensitivity pneumonitis is associated with an increased number of cells and material in the alveoli and interstitium and is an example of a restrictive lung disease. Thus lung volumes will be reduced. Specifically, TLC and, to a lesser extent, functional residual capacity (FRC) and residual volume (RV) are decreased, resulting in an increase in the RV/TLC ratio.
- 4.
Surfactant increases lung compliance overall. In addition, surfactant stabilizes individual alveoli by increasing surface tension at high alveolar volumes and decreasing surface tension as lung volume decreases.
Chapter 3
- 1.
Increasing lung volume increases the length and diameter of the airways. As a result, resistance to airflow decreases with increases in lung volume, but the relationship is curvilinear. At lung volumes greater than FRC, there is little effect on total airway resistance. Below FRC, resistance increases rapidly and approaches infinity at RV.
- 2.
The cross-sectional area of a conducting airway is determined by the forces of contraction (i.e., the elastic forces in the airways and the tension of the smooth muscle surrounding the airways) and the forces of dilation or outward traction (i.e., a positive transpulmonary pressure or the interdependence of alveoli and terminal bronchioles).
- 3.
A reduction in elastic recoil pressure, which is present in individuals with emphysema, will result in a decrease in the driving pressure for expiratory gas flow and in premature airway closure in association with movement of the equal pressure point closer to the alveoli.
- 4.
Airway resistance is determined by flow rate (the more laminar the airflow, the lower the resistance), and airflow velocity becomes very low as the effective cross-sectional area increases. Furthermore, the airways exist in parallel, and as a result the many small airways contribute little to the resistance.
Chapter 4
- 1.
A–D.
- A.
The test appears to be performed in a satisfactory way with a rapid rise to peak flow and a gradual, smooth decline to residual volume. Compared with the normal curve (dotted line), peak flow and expiratory flow rates are decreased. Forced vital capacity (FVC) is normal, with a decreased forced expiratory volume after 1 second (FEV 1 ); this would result in a decrease in the FEV 1 /FVC ratio. These changes are consistent with obstructive pulmonary disease (OPD); the magnitude is approximately 50%, and thus this is moderate OPD.
- B.
The test demonstrates a rapid rise and a smooth decline to the baseline compatible with acceptable quality. The FVC and FEV 1 are reduced, with a normal FEV 1 /FVC ratio. Expiratory flow rates are normal. These changes are consistent with restrictive pulmonary disease; the magnitude is reduced 25% to 50%; thus this is mild-to-moderate restrictive lung disease.
- C.
The test demonstrates a rapid rise to peak flow, a saw-toothed decline, and an abrupt drop in flow to the baseline. The effort as demonstrated by the initial rise is good, but the subject either coughed (sawtooth) or periodically closed the glottis when exhaling. In addition, the subject did not exhale to residual volume (abrupt drop in flow). Thus this flow volume curve is uninterpretable.
- D.
There is a rapid rise to peak flow followed by a gradual, smooth decline to zero flow or baseline. This is an acceptable test. The FVC and FEV 1 are normal and have a normal ratio. Expiratory flow rates at high lung volume (i.e., early in the maneuver or close to TLC) are normal; flow rates at low lung volume (i.e., late in the maneuver or close to RV) are reduced. This is an example of mild obstructive pulmonary disease (OPD) involving the small airways. It is what you might find in an asymptomatic 20 pack-per-year cigarette smoker.
- A.
- 2.
Early in chronic OPD, the spirogram and flow volume curve are normal, but evidence of air trapping (i.e., an elevated RV/TLC ratio) may be present. As the disease progresses, there is further air trapping in association with decreased expiratory flow rates, particularly involving the small airways (see Answer 1D). With further progression, peak flow and airway resistance (Raw) are affected and FEV 1 becomes decreased. When the FVC begins to decrease, the individual has moderate-to-severe obstructive pulmonary disease; when the FVC is below 50% of predicted, the individual has severe obstructive pulmonary disease. Emphysema and chronic bronchitis can both produce pulmonary function abnormalities as already described. The two diseases can be distinguished by the diffusion capacity for carbon monoxide (D lco ). The D lco is normal in chronic bronchitis, whereas it is decreased in emphysema.
- 3.
In individuals with restrictive pulmonary disease, both the FVC and the FEV 1 are decreased but proportionately. This occurs in association with normal (and even supernormal) expiratory flow rates (see Answer 1B) and with decreases in TLC, and less so in RV (resulting in an elevated RV/TLC ratio due to a decrease in TLC). Compliance is also reduced in many (but not all) restrictive lung diseases. As the disease progresses, there is further reduction in these pulmonary function measurements with eventual decreases in expiratory flow rates.
- 4.
A normal D lco requires a normal surface area for gas diffusion. This is the major requirement. However, decreased perfusion secondary to emboli or decreased cardiac output or decreased capillary blood volume such as in anemia also contributes to an abnormal D lco .
Chapter 5
- 1.
As tidal volume (V t ) increases, there is a decrease in dead space ventilation (V ds ) for the same minute ventilation (V e ):
V T = 500 mL If V DS is 150 mL then V E = V T − V DS = 350 mL When V T is 600 mL for the same V E V DS = 600 mL − 350 mL = 250 mL
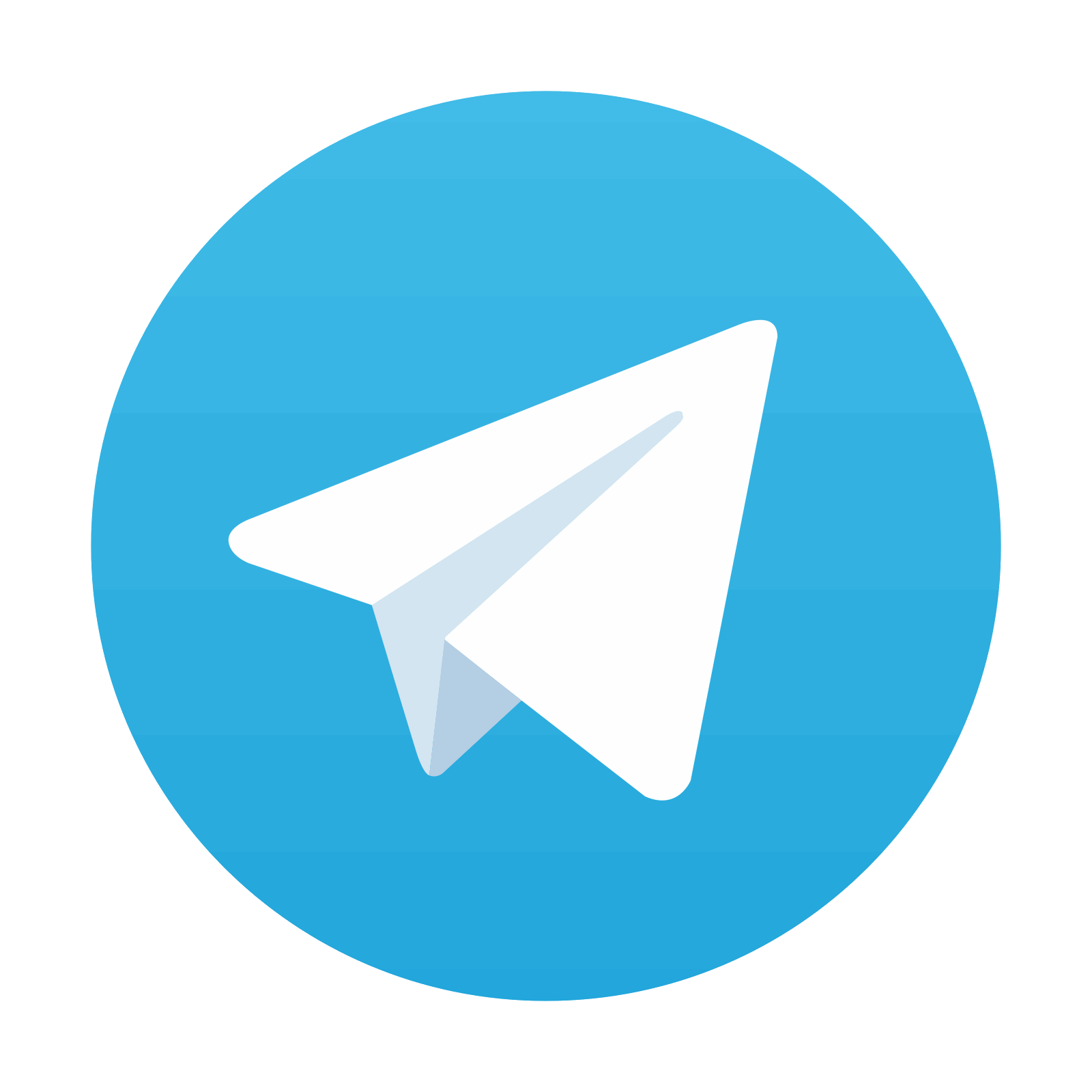
Stay updated, free articles. Join our Telegram channel

Full access? Get Clinical Tree
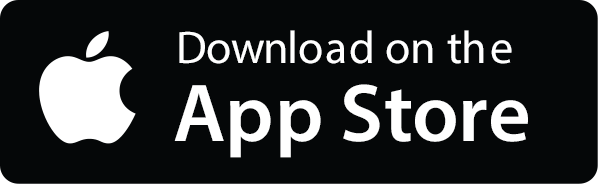
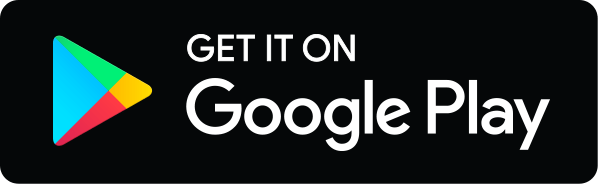
