Chapter 16 Anesthesiology Principles, Pain Management, and Conscious Sedation
Pharmacologic Principles
Inhalational Agents
The original inhalational anesthetics—ether, nitrous oxide, and chloroform—had important limitations. Subsequent drug development has emphasized inhalational agents that facilitate rapid induction and emergence and are nontoxic; these include isoflurane, sevoflurane, and desflurane. Although halothane and enflurane were also commonly used in the past, the use of both agents has decreased dramatically during the last 5 to 10 years. The important aspects of each volatile anesthetic can be summarized in terms of their key clinical attributes (Table 16-1). Two of the most important characteristics of inhalational anesthetics are the blood-gas (B-G) solubility (partition) coefficient and the minimum alveolar concentration (MAC). The B-G solubility coefficient is a measure of the uptake of an agent by blood. In general, less soluble agents (lower B-G solubility coefficients), such as nitrous oxide and desflurane, are associated with more rapid induction of and emergence from anesthesia, whereas induction and emergence are slower with agents having high solubility in blood, such as halothane. MAC is a measure of potency and is defined as the concentration of an agent required to prevent movement in response to a skin incision in 50% of patients. A higher MAC represents a less potent volatile anesthetic. Among volatile agents, halothane is the most potent, with a MAC of 0.75%, whereas desflurane has a MAC of 6% and is the least potent of the hydrocarbon-based volatile agents. Nitrous oxide has a MAC of 104% at sea level, meaning that nitrous oxide alone is generally not suitable for the maintenance of general anesthesia. The pungency of anesthetic agents also has practical implications. Agents with low pungency, such as halothane and sevoflurane, do not cause significant airway irritation when delivered at commonly used concentrations and are useful for inhalation induction of anesthesia. Desflurane is highly irritating to the airways and is not useful for inhalation induction under most conditions.
Nitrous Oxide
Nitrous oxide provides only partial anesthesia at atmospheric pressure because its MAC is 104% of inspired gas at sea level. Nitrous oxide minimally influences respiration and hemodynamics. In addition, it has low solubility in blood. Therefore, it is often combined with one of the potent volatile agents to permit a lower dose of the potent volatile agent, thus limiting side effects, reducing cost, and facilitating rapid induction and emergence. The most important clinical problem with nitrous oxide is that it is 30 times more soluble than nitrogen and diffuses into closed gas spaces faster than nitrogen diffuses out. Because nitrous oxide increases the volume or pressure of these spaces, it is contraindicated in the presence of closed gas spaces such as pneumothorax, small bowel obstruction, and middle ear surgery, as well as in retinal surgery, in which an intraocular gas bubble is created. Because nitrous oxide gradually accumulates in the pneumoperitoneum, some clinicians prefer to avoid its use during laparoscopic procedures. However, periodic venting can prevent buildup, and some investigators have suggested that nitrous oxide might be preferable to carbon dioxide as the insufflated gas.1
Sevoflurane
Sevoflurane’s relatively low solubility facilitates rapid induction and emergence. Sevoflurane is associated with faster emergence than isoflurane, especially in longer cases, although its slightly faster emergence does not result in earlier discharge after outpatient surgery. Sevoflurane is associated with a lower incidence of postoperative somnolence and nausea in the postanesthesia care unit (PACU) and in the first 24 hours after discharge than isoflurane. Unlike isoflurane, sevoflurane is pleasant to inhale, thus making it suitable for inhalational induction in children. Sevoflurane is clinically suitable for outpatient surgery, mask induction of patients with potentially difficult airways, and maintenance of patients with bronchospastic disease. When sevoflurane, halothane, and isoflurane were compared, all these potent agents decreased respiratory resistance in endotracheally intubated nonasthmatics. However, sevoflurane reduced airway resistance more than halothane or isoflurane.2
Considerable metabolic transformation of sevoflurane takes place and results in increases in the serum fluoride ion concentration and, in the presence of soda lime, production of compound A, a metabolite that is nephrotoxic in experimental animals. However, β-lyase, the enzyme responsible for the formation of compound A,3 has 8 to 30 times greater activity in rat kidneys than in human kidney tissue. Therefore, the nephrotoxicity of compound A in humans appears to be theoretical and not clinically important.
Desflurane
Desflurane is rapidly taken up and eliminated. After anesthesia lasting more than 3 hours, desflurane was associated with more rapid recovery than isoflurane.4 Its pungent odor precludes inhalational induction. In addition, desflurane is associated with tachycardia and hypertension if the concentration is increased too rapidly. When exposed to dry carbon dioxide absorbent, volatile anesthetics are partially converted to carbon monoxide. Desflurane, enflurane, and isoflurane produce more carbon monoxide than halothane or sevoflurane does. Carbon monoxide production is greater with dry CO2 absorbent, with Baralyme more than with soda lime, at higher temperatures, and at higher anesthetic concentrations.5 Because continued gas flow in an unused machine will desiccate the CO2 absorbent, turning gas flow off in anesthesia machines when they are not in use can reduce carbon monoxide production. It is also prudent to run fresh gas through an anesthesia machine that has not been used for 1 or 2 days to wash out any carbon monoxide that might be present prior to patient contact.
Intravenous Agents
Induction Agents
Induction with thiopental, the oldest IV induction agent, is rapid and pleasant. Although the drug is remarkably well tolerated by a wide variety of patients, several clinical situations necessitate caution (Table 16-2). In hypovolemic patients and those with congestive heart failure, thiopental-induced vasodilation and cardiac depression can lead to severe hypotension unless doses are markedly reduced. In these patients, etomidate or ketamine is an alternative agent. Although thiopental does not directly precipitate bronchospasm, it does not blunt airway reactivity in response to the intense airway stimulation produced by endotracheal intubation as effectively as propofol or ketamine, which are attractive alternatives for patients with reactive airway disease.
Ketamine, which produces a dissociative state of anesthesia, is the only IV induction agent that increases blood pressure and heart rate and decreases bronchomotor tone. Usually associated with increased sympathetic tone, ketamine causes direct cardiac depression that may become evident if given to patients with high preanesthetic sympathetic tone, as in patients in hemorrhagic shock. In markedly reduced doses (15% to 20% of the usual induction dose), ketamine is an appropriate choice for IV induction of severely hypovolemic patients, in whom it causes the least fall in blood pressure of any of the induction agents. Ketamine is an appropriate agent for IV induction of asthmatic patients because it reduces bronchomotor tone and decreases the airway reactivity associated with endotracheal intubation. Among the IV induction agents, ketamine also causes the least amount of ventilatory depression and loss of airway reflexes. However, because of the induction of copious oropharyngeal secretions, an antisialogogue such as glycopyrrolate is generally administered with ketamine. Ketamine can be used as the sole anesthetic for brief superficial procedures because it produces profound amnesia and somatic analgesia. It is less useful, however, for abdominal cases or delicate surgery because it produces no muscular relaxation, does not control visceral pain, and may not completely control patient movement. The potent pain-relieving effects of ketamine have been exploited for preemptive analgesia. In patients in whom ketamine was infused continuously before incision and continued through wound closure, postoperative morphine consumption was significantly lower on postoperative days 1 and 2 than in patients who did not receive ketamine.6
Propofol also produces excellent bronchodilation. In asthmatic patients, 0% of those who received propofol wheezed at 2 or 5 minutes after intubation versus 45% of those who received a thiobarbiturate and 26% of those who received an oxybarbiturate.7 In nonasthmatic patients, 75% of whom smoked, airway resistance was less after induction with propofol than after induction with thiopental or etomidate. Brown and Wagner8 have demonstrated that the bronchodilatory effects of propofol and ketamine are mediated through the blockade of vagus nerve–mediated cholinergic bronchoconstriction.
Although not used as an induction agent, dexmedetomidine has gained increased value as a sedative agent in anesthetic care.9 Dexmedetomidine is a selective α2-adrenergic receptor agonist that has sedative, amnestic, and analgesic properties. It has value as a preoperative sedative, anesthetic adjunct and sedative-hypnotic for the management of sedation in critically ill patients. It causes minimal respiratory depression but can have significant cardiovascular effects, including bradycardia and hypotension, if given too rapidly or used for patients with significant hypovolemia.9
Opioids
Several reasons explain the universal popularity of opioids in anesthetic management. First, they reduce the MAC of potent inhalational agents. For example, fentanyl (3 ng/mL plasma concentration) decreased the MAC of sevoflurane by 59% and reduced MACawake (the alveolar concentration at which an emerging patient responds to commands) by 24%.10 Second, they blunt the hypertension and tachycardia associated with manipulations such as endotracheal intubation and surgical incision. Third, they provide analgesia that extends throughout the early postemergence interval and facilitates smoother awakening from anesthesia. Fourth, in doses 10 to 20 times the analgesic dose, opioids act as complete anesthetics in a high proportion of patients by providing not only analgesia but also hypnosis and amnesia. This characteristic has prompted their use for cardiac surgery patients, sometimes as sole anesthetic agents and more often as a major component of the anesthetic. Finally, they are now often added to local anesthetic solutions in epidural and intrathecal blocks to improve the quality of analgesia.
Neuromuscular Blockers
Succinylcholine, the only depolarizing agent still in clinical use, continues to be useful for endotracheal intubation because of its rapid onset and short duration of action. However, it is associated with serious hazards, including hyperkalemia and malignant hyperthermia, in a small proportion of patients. The drug can be administered in a relatively high dose for intubation because it is rapidly metabolized by plasma pseudocholinesterase, except in a small fraction of patients with atypical or absent pseudocholinesterase. Because its duration of action is only 5 minutes, a patient who cannot be successfully intubated can be ventilated by mask for a short time until spontaneous respiration resumes. However, a patient who cannot be ventilated by mask will not resume spontaneous breathing after an intubating dose of succinylcholine before the onset of life-threatening hypoxemia.11
Nondepolarizing relaxants are used when succinylcholine is contraindicated, as an alternative to succinylcholine for patients in whom easy endotracheal intubation is anticipated, and when prolonged intraoperative relaxation is required to facilitate surgical exposure. Knowledge of the side effects of individual agents, often related to vagolysis or release of histamine, and routes of metabolism plays a major role in the selection of specific agents for individual cases. Doses required to provide satisfactory operating conditions are summarized in Table 16-3. Dosing of nondepolarizing agents requires knowledge of several important characteristics. First, the use of neuromuscular blockers prevents movement in response to noxious stimuli. Therefore, chemical paralysis can mask the signs of inadequate anesthesia, or sedation or analgesia in postoperative patients. Medicolegal claims of intraoperative awareness during general anesthesia were more than twice as frequent in patients receiving intraoperative muscle relaxants.12 Second, higher doses are required to provide satisfactory conditions for intubation than for surgical relaxation. Therefore, if a nondepolarizer is used only after intubation, smaller doses are required. Third, other anesthetic drugs potentiate the actions of nondepolarizing agents. Potent inhalational agents potentiate the effects of competitive neuromuscular blockers in a dose-dependent fashion. The newer inhalational agent desflurane potentiates the effects of vecuronium approximately 20% more than isoflurane.13 Fourth, individual responses to muscle relaxants vary widely, with patients demonstrating markedly increased and decreased neuromuscular blockade in comparison to expected levels.
Fifth, and most important, subtle blockade can be difficult to detect and can be associated with postoperative weakness. The importance of subtle residual paralysis has been quantified by using the train-of-four (TOF) fade ratio, a semiquantitative monitoring technique used to assess the adequacy of neuromuscular blockade and the adequacy of pharmacologic reversal. A 1997 report characterized the symptoms of volunteers receiving graded doses of muscle relaxants at various TOF ratios.14 A sustained 5-second head lift, a commonly used clinical index of adequate reversal, was achieved if the TOF ratio was higher than 0.60. At TOF ratios higher than 0.70, all subjects maintained patent airways and oxygen saturation higher than 96%. However, in a 2003 study, at TOF ratios less than 0.90, subjects had diplopia and difficulty tracking objects in all directions. The ability to oppose the incisors strongly did not return until the TOF ratio was higher than 0.90. It was concluded that satisfactory return of neuromuscular function requires return of the TOF ratio to higher than 0.90 and, ideally, to 1.0.15 In patients who received the intermediate-acting neuromuscular blockers atracurium, vecuronium, or rocuronium only for endotracheal intubation, the TOF ratio was lower than 0.9 in 37% of patients 2 hours after receiving the muscle relaxant.15
With the longer acting muscle relaxants, such as pancuronium, residual blockade can potentially complicate postoperative recovery. In a clinical trial, 691 patients undergoing abdominal, gynecologic, or orthopedic surgery under general anesthesia were randomized to receive pancuronium, vecuronium, or atracurium. After reversal with neostigmine, a higher proportion (26%) of patients who had received pancuronium had residual neuromuscular blockade (TOF < 0.70) than patients who had received vecuronium or atracurium (5.3% combined).16 Patients who received pancuronium and had a TOF ratio less than 0.70 had a higher incidence of atelectasis or pneumonia on postoperative chest radiographs (16.9% of 59 patients in that category). There was no association between postoperative pulmonary complications and residual blockade with the other two muscle relaxants, with intermediate durations of action.
Anesthesia Equipment
Anesthesia equipment has undergone rapid development over the past several decades. The central piece of equipment for delivery of anesthesia is the modern anesthesia machine, which functions primarily to deliver oxygen and volatile anesthetics to the patient. In addition, modern anesthetic machines have sophisticated ventilators that allow for effective respiratory support and integrated monitors that accurately measure oxygen delivery, inspired and end-tidal gas concentrations, airway pressures, minute ventilation, and fresh gas flows. Despite many years of improving design, the hazards of gas delivery systems must still be considered. The primary concern is inadvertent delivery of a hypoxic gas mixture. Adverse anesthetic outcomes were associated with gas delivery equipment in 72 of 3791 cases in the American Society of Anesthesiologists (ASA) closed claims database. Misuse of equipment occurred in 75% of incidents, and 78% could have been detected with monitoring of pulse oximetry or capnography.17
Patient Monitoring During and after Anesthesia
The indications, risks, and benefits associated with the use of noninvasive and invasive electronic monitors must be assessed for each individual patient (Box 16-1). These decisions are guided by the patient’s medical condition, type of surgery, and potential complications associated with invasive monitoring. However, the proliferation of electronic monitoring devices does not circumvent the need for clinical skills such as observation, inspection, auscultation, and palpation. The ASA has established standards for basic anesthetic monitoring.18 These have been designed to integrate clinical skills and electronic monitoring with the goal of enhancing patient safety.
Box 16-1 Electronic Monitors Used for Anesthesia and Their Indications
Neuromuscular Blockade Monitoring
Because of variability in sensitivity to neuromuscular blockers among patients, it is essential to monitor neuromuscular function in patients receiving intermediate- and long-acting muscle relaxants. The most common sites of monitoring are at the ulnar or orbicularis oculi muscles. The basis of neuromuscular monitoring is assessment of muscle activity after proximal nerve stimulation (Box 16-2). This evaluation indicates acetylcholine receptor blockade at the neuromuscular junction. The degree of neuromuscular blockade is indicated by a decreased evoked response to twitch stimulation.
Box 16-2 Techniques for Assessing Neuromuscular Blockade
Train-of-Four Fade Ratio (Four Successive 200-µsec Stimuli Over 2-Sec Period)
Twitch height progressively fades with increasing blockade:
Presence of four twitches without fade suggests adequate reversal of neuromuscular blockade.
Double-Burst Stimulation: Two Successive Sets of 50-Hz Bursts (Three Stimuli/Burst) Separated by 750 µsec (Appears as Two Twitches)
Easier to detect fade visually with this technique than with train-of-four fade ratio
Loss of second twitch indicates 80% receptor blockade
Presence of twitches without fade suggests adequate reversal of neuromuscular blockade
Central Nervous System Monitoring
Awareness during anesthesia is an uncommon but disturbing complication. Many years of experience with intraoperative electroencephalographic signal processing has resulted in development of the bispectral array (BIS), which is thought to monitor awareness during anesthesia. The monitor is essentially a modified electroencephalogram (EEG) that assesses brain wave activity and reports numbers from 0 to 100, which correlate with the level of awareness. A value of 100 represents complete awareness and 0 represents complete suppression of brain wave activity. Evidence has suggested that BIS is an accurate indicator of the depth of anesthesia.19 Monitoring the depth of anesthesia may improve time to awakening and discharge in the outpatient setting. Furthermore, some reports have indicated that BIS values lower than 40 for more than 5 minutes during general anesthesia may be associated with increased perioperative morbidity, including myocardial infarction (MI) and stroke in high-risk patients.20 In addition, BIS monitors are gaining acceptance as a means of assessing awareness in locations such as emergency departments and intensive care units.
Preoperative Evaluation
The ASA has established basic standards for preanesthetic care in which an anesthesiologist is required to evaluate the medical status of the patient, derive a plan for anesthetic care, and discuss the plan with the patient.21 The Joint Commission (TJC) requires that all patients receiving anesthesia undergo a preanesthetic evaluation. Because a decreasing percentage of patients are admitted to the hospital on the day before surgery, preoperative testing clinics have been developed to facilitate preoperative evaluation. The advent of preoperative clinics has facilitated the efficient use of operating room resources. Ferschel and colleagues22 have reported that development of an anesthesia preoperative evaluation clinic in a teaching hospital reduced day of surgery cancellations and delays. Optimally, preoperative clinics need to be efficient, predictable, and thorough. In modern practice, many patients without complicated medical problems who are scheduled for elective, low-risk procedures can be interviewed by telephone or online teleconference prior to surgery and given preoperative instructions.
Airway Examination
Assessing the airway is a crucial step in developing an anesthetic plan. Even if regional anesthesia is planned, general anesthesia and the need to maintain a patent airway might be necessary. The goal of the airway examination is to identify characteristics that could hinder assisted mask ventilation or tracheal intubation. A history of diseases or conditions that are associated with airway closure or difficult laryngoscopy will alert the physician to potential airway difficulties. Review of previous anesthetic records can provide invaluable information regarding previous airway management. The airway examination is completed by systematic inspection of the mouth opening, thyromental distance, neck mobility, and size of the tongue in relation to the oral cavity (Box 16-3). The patient is observed in frontal and profile views because many airway abnormalities, such as a receding mandible, will not be evident from a frontal view. The size of the tongue in relation to the oral cavity can be graded by using the Mallampati classification (Fig. 16-1). The Mallampati examination is performed with the patient sitting and the head in a neutral position, mouth opened as wide as possible, and tongue protruded maximally. The observer views the oral and pharyngeal structures that are evident. In general, a patient in whom the uvula, tonsillar pillars, and soft palate are visible (class I) will be easy to mask ventilate and intubate. Patients in whom only the hard palate is visible, a class IV airway, have a higher likelihood of being difficult to mask-ventilate and intubate. However, the Mallampati classification is only one component of the airway examination; it must be used in conjunction with other aspects of the airway examination and the patient’s history to provide a complete airway assessment. Other physical factors associated with uncomplicated airway management are adequate mouth opening, neck extension, and thyromental distance. In a meta-analysis examining more than 50,000 patients, Shiga and associates23 have reported that individual physical characteristics, by themselves, have poor predictive value for identifying airway difficulties. However, the combined presence of two or more physical end points that predict difficult airway management increasingly improves sensitivity and specificity.
Box 16-3 Important Factors When Performing an Airway Examination
Patient History
Medical history (e.g., history of oropharyngeal mass, pharyngeal disease)
Review of chart to assess prior airway management during previous anesthesia
Cardiovascular Disease
The risk for perioperative myocardial ischemia and infarction and the risk for cardiac death have become important issues as progressively more complex surgery has been offered to patients with increasingly severe systemic disease. The apparent incidence of perioperative myocardial ischemia depends on the perspective of the study, prospective or retrospective, sensitivity of the markers used, and type of surgical procedure. Based on review of the available literature, the American College of Cardiology (ACC) and the American Heart Association (AHA) have published guidelines for the evaluation and treatment of CAD in noncardiac surgical patients.24 These guidelines focus on the patient’s history of CAD, exercise tolerance and type of surgery proposed. A detailed history and physical examination are required to assess the presence of underlying cardiovascular disease. Assessment of functional status and the ability to perform common daily tasks is a critical part of the assessment. Patients with active major cardiovascular conditions require evaluation and treatment before undergoing noncardiac surgery. The following are considered active cardiac conditions:
In the 2007 revision of the ACC/AHA guidelines, the previously used intermediate-risk category was replaced with clinical risk factors from the Revised Cardiac Risk Index, with the exclusion of the type of surgery, which is included elsewhere in the approach to the patient.24 These clinical risk factors are incorporated into the overall evaluation of the patient (Fig. 16-2). The clinical risk factors that are part of the Revised Cardiac Risk Index include the following:
Figure 16-2 presents a framework for determining which patients are candidates for preoperative cardiac testing. The clinician must consider several interacting variables and give them appropriate weight. Since publication of the perioperative cardiovascular evaluation guidelines in 2002, several new randomized trials and cohort studies have led to modification of the original algorithm. The following stepwise approach is recommended24:
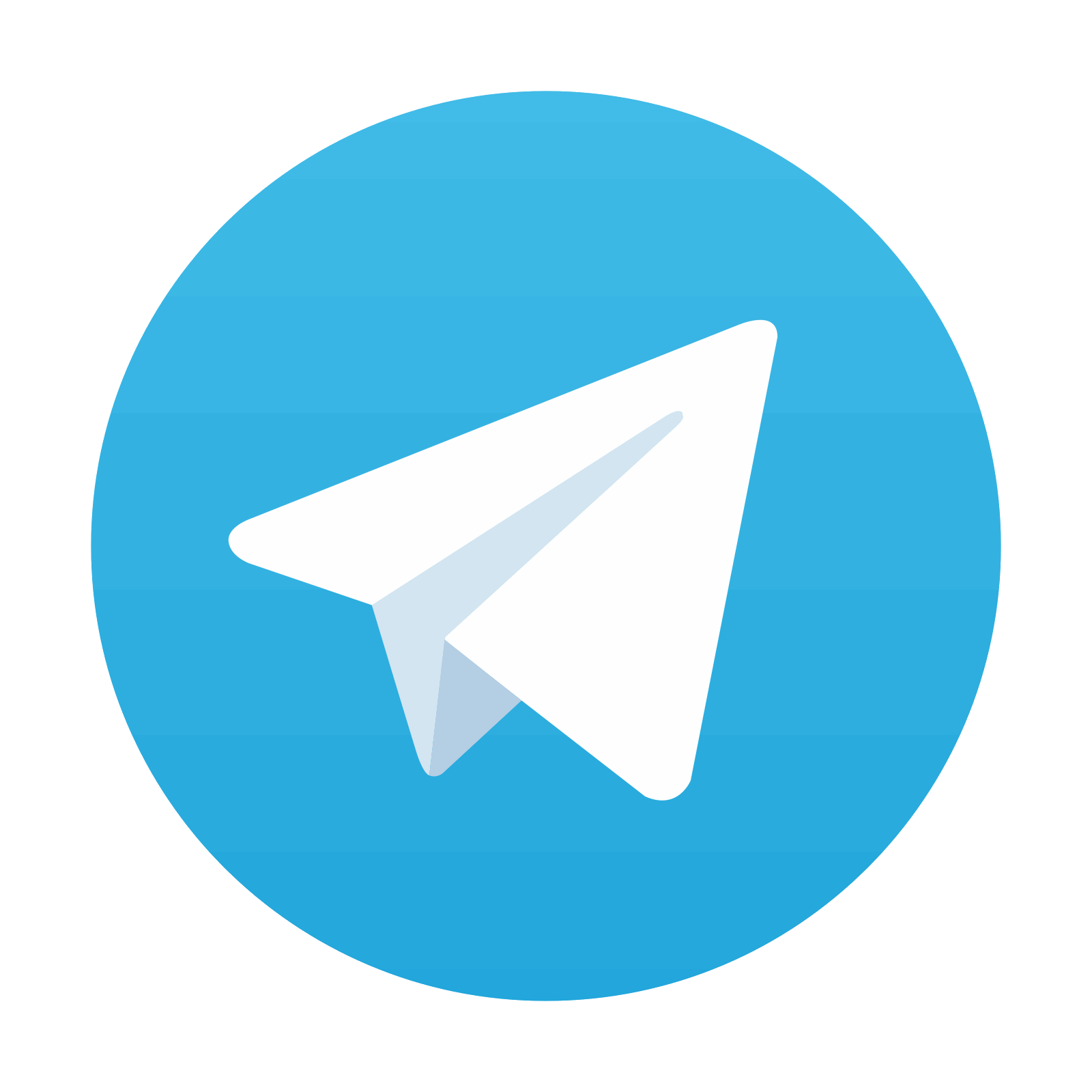
Stay updated, free articles. Join our Telegram channel

Full access? Get Clinical Tree
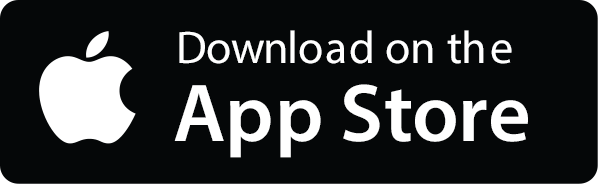
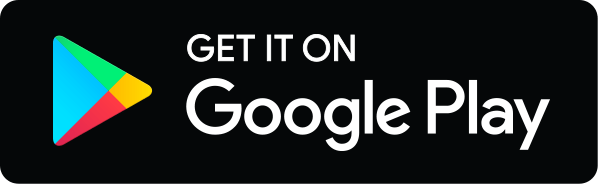