H. Jay Przybylo Feinberg School of Medicine, Northwestern University, Chicago, IL, USA The contemporary era of surgical intervention to correct congenital heart defects began in 1938 when Robert E. Gross, assisted by the anesthesia team at Boston Children’s Hospital, performed an innovative surgery to successfully ligate a patent arterial duct in a 7‐year‐old child [1]. At that time, medications for pediatric anesthesia consisted of volatile general anesthetic agents and narcotic analgesics. Today, 80 years after Gross’s landmark operation, some of these anesthetics and narcotics remain an integral part of the anesthetic regimen for pediatric patients undergoing cardiac surgical procedures. In the modern era pediatric anesthesiologists continually strive to provide safer operating conditions for the patient. In addition to improved anesthetic agents and narcotics, monitoring techniques and therapies that limit or prevent complications from the underlying cardiac disease have allowed more patients with complex cardiac defects to undergo interventional and surgical repair. Currently, we are working on strategies to prevent complications resulting from the inflammatory response to cardiopulmonary bypass (CPB) and to provide better cerebral protection during artificial or altered circulation [2]. Pediatric cardiac anesthesia care has expanded outside of the conventional operating room and now also includes the cardiac catheterization suite, interventional radiology, computed tomography (CT) scanner, and magnetic resonance imaging (MRI) center [3]. Perhaps the greatest changes we are experiencing are the collection, analysis, and distribution of data. Improvements in the speed and storage capability of information technology allow studies of large samples to be easily collected, evaluated, and transmitted worldwide. In recent years a trend has emerged that shows a decrease in overall mortality for patients who have undergone repair of a congenital cardiac defect in infancy or childhood. This has created a growing population of adult patients presenting for anesthesia care for all types of procedures, cardiac and noncardiac [4]. As more children with congenital heart disease survive, many of them will require revision of previous surgical interventions or further diagnostic and therapeutic procedures, including those that are unrelated to the heart defect such as appendectomies, fractures, and pregnancy. The American Heart Association estimates that there are approximately 1.4 million people alive in the United States who have had a procedure for a congenital cardiac malformation [5]. We are now anesthetizing patients with congenital heart disease who are in their fifth and sixth decades of life and have the health concerns of adulthood complicating the normal pediatric cardiac anesthesia care [6]. In order to provide care for this growing patient population, anesthesiologists must be knowledgeable in all aspects of congenital heart disease and repair. The development and improvement of ultrasound technology made it possible to not only diagnose congenital heart disease in utero, but also provide a greater insight into fetal pathophysiology and neonatal cardiac anatomy [7]. The various imaging modalities available today aid cardiologists and congenital heart surgeons in determining the optimal timing for and the best type of early intervention. As heart malformations are the most common congenital defect requiring anesthesia care today, it is expected that members of the cardiac anesthesia team will anesthetize more neonates than any other group of patients who require cardiac procedures. The cardiac anesthesia team therefore will be responsible for the care of patients from extreme prematurity to 50 plus years of age [8]. Our experience has demonstrated that approximately one‐third of patients who require congenital cardiac anesthesia are less than 1 year of age, one‐third are 1–15 years of age, and one‐third are adults (some of them in the sixth decade of life). Neonates and infants tend to have malformations that result in abnormal blood flow, while older patients are more likely to suffer from heart rhythm disturbances and valve dysfunction. This knowledge is important in developing cardiac anesthesia teams, educational programs, and anesthetic protocols [9]. In addition to young age, other variables related to increased morbidity and mortality in anesthesia include increased severity of the illness as measured by physical status and emergency procedures [10, 11]. Overall mortality with improved interventional and anesthetic techniques has decreased to less than 5% for all patients with congenital heart disease [12]. Vigilance is ever important for the anesthetist, as anesthesia care provided to children with congenital heart defects entails even greater risk over other children [13, 14]. The demographic considerations clearly demonstrate the wide scope of patient care required by the cardiac anesthetist and the American Academy of Pediatrics has recommended that a pediatric cardiovascular center use a team approach for all aspects of care and intervention of these patients [15]. The quality of life in children after repair of congenital heart disease is regarded as lower than in other children; the more severe the lesion, the lower the quality of life [16]. The anesthesia team will be charged with developing new modalities of care that will improve on this finding. These complex patients require a thorough evaluation by the anesthesiologist before the procedure. The evaluation will include the present and past history, including previous anesthetic experience, family history, prenatal evaluation when available, a physical examination, and review of all studies performed leading to the intended procedure. Our task in anesthesia has become easier with the electronic medical record. Before the patient visit, it is now possible to review all previous encounters and interventions, be aware of the diagnosis and medical history to date, have a list of all medications, obtain all test results, and review previous anesthetic exposures. In addition, information regarding the specifics of the congenital heart lesion and therapy can be quickly researched via internet access, searching both surgery and anesthetic databases [9, 17]. A note of caution: this should be done prior to examining the patient, because motivated parents will have this information in hand and issues and questions can be handled in a timely fashion, preventing unnecessary delays searching for pertinent information and building the confidence of parents. The history should include information specific to the malformation present and the age of the patient, including gestational age for newborns. The prenatal and maternal history should be obtained to balance confounding issues such as prematurity and maternal diabetes. The presence of one congenital defect should always initiate the search for others and the possible presence of a syndrome that could alter the anesthetic management. Cardiac lesions are in the midline groups of congenital defects and are associated with neurologic, renal, and intestinal defects in addition to other organ systems, the VACTERL association (vertebral defects, anal atresia, cardiac defects, tracheo‐esophageal fistula, renal anomalies, and limb abnormalities). Altered neurologic outcome in children with congenital heart disease is now well recognized, and the history should include specifics of the birth and other indicators of preexisting neurologic impairment [18]. Children can be uncooperative with diagnostic testing and exercise tolerance might not be easily quantitated. Probing the level of physical activity by history can give an indication of cardiac function. Frequent respiratory infections are associated with congestive heart failure. Other more subtle indicators include the feeding history and the frequency of respiratory infections. A child with low cardiac reserve may have difficulty eating and be smaller in stature and weigh less than anticipated. Continuing debate regarding the management of children with upper respiratory tract infections remains. If multiple symptoms are present the airway might be reactive, provoking hypoxemic episodes surrounding the time of anesthesia. The patient’s temperature might fluctuate during the administration of anesthetics and airway secretions may increase, leading to the possibility of mechanical obstruction of the endotracheal tube. If it is possible the case should be delayed until resolution of the infection [19]. Adult patients, in addition to a complete history of the cardiac malformation and previous interventions, are questioned regarding disease process related to age. The patient’s internist and all other adult specialists caring for the patient should be consulted. The history may help determine cardiac function, but this can also be measured by exercise testing. Some patients with prior repairs develop heart rhythm disturbances over time and this should be investigated. Patients with functionally univentricular hearts require special attention. These patients live with passive pulmonary blood flow. Worsening cyanosis can be an indicator of collateral vessel formation or intracardiac shunting. Previous Fontan procedures are associated with protein‐losing enteropathy, thromboembolic events, hepatic dysfunction, and thyroid dysfunction as a result of amiodarone therapy for rhythm disturbances [20]. The presence of single left ventricle (versus right) is beneficial information. The physical examination by the anesthesiologist is a tool to confirm information obtained by the history and the review of previous records. The physical examination also concentrates on the anatomy of the airway, the ability to gain vascular access, and an examination of pulses to determine the feasibility of arterial line placement. Preanesthetic blood testing includes electrolyte levels, with special attention to potassium levels potentially altered by or associated with diuretic therapy. Patients with cyanotic heart disease compensate for hypoxemia with polycythemia. There is no agreement at present regarding the upper limit of acceptable hematocrit and the decision to decrease the red cell mass by phlebotomy is generally guided by the presence of associated complications such as thrombosis. Platelet count and coagulation studies should be obtained in patients undergoing CPB. The American Society of Anesthesiologists has developed guidelines for recommended fasting before the induction of anesthesia [21]. These guidelines are intended to provide safe conditions for the induction of anesthesia with the minimum risk of aspiration of gastric contents as the airway reflexes are ablated. The recommendations for fasting balance the concern for preventing dehydration and hypoglycemia while limiting the amount of gastric volume and acid content. Special considerations regarding fasting include the administration of routine medications as prescribed with a small amount of clear nonfat‐containing fluid. Patients with univentricular physiology are encouraged to maintain normovolemia through oral intake of clear fluids, optimizing pulmonary blood flow at the time of anesthesia induction. It is generally safe to allow clear liquids up to two hours prior to the scheduled start of anesthesia. Most outpatient elective cardiac patients benefit from premedication before being taken to the operating room. Several studies have demonstrated that midazolam and dexmedetomidine decrease anxiety and improve the process of parental separation [22]. Parental presence in the operating room at induction has also been used. No reliable evidence has proven this to be beneficial over other means of anxiolysis. More recently tablet‐based interactive devices have been shown to help reduce perioperative anxiety when used in the operating room [23]. Individual preferences, parental cooperation, and the ability to administer and monitor the effects of these medications are determinants of best practice for the anesthesiologist. In the child with poor or indeterminate cardiac function, the use of ketamine may be considered. Concerns of increased pulmonary artery pressures and worsening of cyanosis have not been proven [24]. Ketamine can be administered by virtually all routes. Ketofol, a combination of ketamine and propofol, is currently a popular choice for procedural sedation as patients are noted to maintain stable hemodynamics with an appropriate level of sedation. In a double‐blind randomized clinical study, 84 adult patients with ischemic left ventricular dysfunction (ejection fraction <40%) were randomly assigned to two groups: etomidate versus ketofol. A decrease in all hemodynamic parameters (baseline systolic and diastolic arterial blood pressure, mean arterial pressure, and heart rate) from induction time to laryngoscopy was greater in the ketofol group than in the etomidate group. In light of this, etomidate remains the sole anesthetic induction agent maintaining cardiac function [25]. In children, ketofol is often used in the emergency department for emergent intubation and procedural sedation. However, further studies are warranted to assess the efficacy and safety of using ketofol in critically ill patients such as those with congenital cardiac defects [26]. Aside from the standard equipment for the administration of anesthesia, special considerations for congenital cardiac anesthesia include the availability of intravenous (IV) fluids and various fluid and blood administration sets. Our standard practice for the majority of open‐heart cases is two peripheral IV lines, a double‐lumen central line, and a peripheral arterial line. If excessive or brisk blood loss is a potential problem, as in reoperations for patients with coagulopathies, rapid infusion devices and infusion pumps should be prepared and determined to be functioning appropriately. Distal infusion and low‐volume injection ports are used to allow prompt administration of medications to the child’s circulation. This will also avoid fluid overload in extremely small infants and speed the effects of changes in dosing of medications by continuous infusions. Equipment for airway management should be prepared and checked for function with all necessary devices (especially fiberoptic, both flexible and rigid capability) to ensure that safe securing of the airway is readily available for patients with potentially difficult airways. Resuscitation medications should be prepared based on the patient’s weight, accurately labeled, and placed in locations that are easily available and will not cause confusion with other anesthetic medications. For example, heparin for anticoagulation before CPB is prepared on a weight basis and placed in a position unique for this drug and away from other medications. Methods for heart rhythm control including the defibrillator should be prepared. Medications used in the process of anesthesia, such as antifibrinolytic, inotrope, vasodilator, and sedation infusions, should be prepared and checked for accuracy. The complexity of caring for these patients is nowhere more obvious than in the number of drugs administered and the different routes by which this is accomplished. The continuous infusion of medications is particularly prone to error. Great care must be taken to assure the concentrations are correct, the infusion pump is programmed appropriately, and the delivery system is connected without error. This is even more critical when caring for extremely small infants. The availability of blood and blood products should be confirmed prior to the induction of anesthesia. The responsibilities for all members of the team should be delineated prior to starting the induction of anesthesia. Airway management, monitor placement, and venous and arterial access are designated to individual members to ensure expedient preparation of the patient for the procedure. The operation of the defibrillator is also clarified before the beginning of the case. The induction of anesthesia is primarily directed by the cardiac function. In patients with normal ventricular function, any of the standard anesthetic agents can be safely used for induction. In patients with decreased ventricular contractility, ketamine remains a popular choice and can be used by either the intramuscular or IV route. Etomidate administered by the IV route is useful in older patients, potentially avoiding the psychic phenomena associated with ketamine. The addition of a benzodiazepine to ketamine also avoids psychic phenomena. Anesthesiologists often face challenges in establishing vascular access in patients with congenital heart defects. The high number of newborn infants and patients with multiple previous procedures limits available sites for both venous and arterial access. Consideration of these issues before inducing anesthesia allows alternate plans to be developed, adds efficiency to the process, and minimizes anesthesia time. Experience in ultrasound‐guided vascular access should be part of the cardiac anesthesiologist’s skill set, as evidence exists proving the success of utilizing ultrasound for guided vascular access. The use of ultrasound‐guided placement has been well demonstrated in accessing the central venous compartment via the internal jugular vein. However, in our experience we find that children do not tolerate catheters in the neck well and object to the tethering effect of IV tubing at this site during the recovery period of surgery. Hence, our preference has been for subclavian vein central venous access. Ultrasound‐guided access has been shown to decrease the time to successful placement of catheters for venous access [27]. Improved first and second attempt rates decreased the rate of complications using ultrasonography in gaining arterial access [28, 29]. Guided technology may be a beneficial tool to the anesthesiologist, in part because of the improvement, growing familiarity with, and availability of ultrasonography. Another paradigm shift in approaching the patient with difficult vascular access involves a change in technique. In anesthesia we learn to gain venous access using catheter over needle devices. Micropuncture technique and equipment utilize a guide wire through a narrow‐gauge needle, dilators are used as necessary, and the catheter is then placed over the guide wire. Halothane, previously associated with a high incidence of cardiac complications, is no longer available for use. The remaining volatile agents provide essentially equal cardiac stability and all can be used successfully. Of the volatile anesthetic agents currently used in pediatric anesthesia, sevoflurane is more easily accepted by children. During induction care must be taken to observe vital signs. There is evidence that all the volatile agents are cardioprotective and decrease mortality in patients anesthetized for heart surgery [30, 31]. An anesthetic technique of high‐dose narcotics was previously shown to be the best technique to prevent the stress response during and after anesthesia for the repair of congenital heart defects in infants [32]. Halothane was the volatile agent used in comparison. More recently, 50 μg/kg total fentanyl dose has been demonstrated to be a sufficient amount for anesthesia; higher doses of 100 μg/kg were noted to increase the frequency of hypotension without noticeable improvement in the state of anesthesia [33]. In more recent studies the focus has been on the benefits of cerebral protection and preconditioning of the heart [34]. These studies have demonstrated the benefits of using volatile anesthetic agents during and after CPB to maintain cardiac function after intervention [31, 35]. Sevoflurane might depress cardiac function to a greater extent than isoflurane following CPB and the use of all volatile agents in this period should be carefully monitored [36]. Current literature suggests that IV anesthetics such as ketamine and dexmedetomidine may induce perioperative neuroprotective properties [37–39]. Presently a combination of moderate‐dose fentanyl up to 50 μg/kg with sevoflurane before initiating CPB and either desflurane or isoflurane during and after CPB achieves successful results. Patient recall is possible with narcotic technique, and amnesic agents should be administered, either benzodiazepines or volatile agents at a concentration of one‐half minimum alveolar concentration. Rapid cooling and rewarming are associated with impaired neurologic outcome, and weaning from CPB at lower core temperatures utilizing prolonged hypothermic neuroprotection may be warranted [36, 40]. It is well recognized that CPB results in an inflammatory response. The impact of all facets of anesthesia care on the inflammatory response remains unclear. This includes the use of steroids and volatile agents and the use of ultrafiltration following CPB [41, 42]. A method for intraoperative monitoring and clinical correlation after repair entails the use of transesophageal echocardiography (TEE). Care should be exercised after the probe is placed in infants, as the immature airway lacks cartilage support and is prone to collapse. Carbon dioxide retention may occur without a concomitant increase in end‐tidal levels [43]. In patients with a retroesophageal subclavian artery, passage of the TEE probe may affect the waveform of the arterial line if it is placed in the affected arm. Three specific cardiac lesions deserve special attention. First, the newborn with hypoplastic left heart syndrome (HLHS) remains a particular clinical challenge. Risk is entailed when these babies are transported from the neonatal intensive care unit to the operating room. These newborns require a high pulmonary vascular resistance to promote systemic perfusion. Extreme caution must be exercised in transporting and caring for these patients before the initiation of CPB. Low oxygen concentration, normo‐ to hypercapnia, and aggressive systemic afterload reduction are necessary to maintain the delicate balance of pulmonary to systemic blood flow [44–46]. Oxygen saturation should be kept at 70–80%; excessive oxygen saturations result in a decrease in the pulmonary vascular resistance and excessive pulmonary blood flow at the expense of systemic perfusion. The second condition, the patient with Fontan physiology, also requires careful attention to ventilation parameters. Passive pulmonary blood flow is optimized with ventilation that allows negative intrathoracic pressures [47]. Positive‐pressure ventilation decreases pulmonary blood flow, and continuous positive airway pressure can have deleterious effects on perfusion, both pulmonary and systemic. Early extubation, or at least spontaneous ventilation, of these patients is a consideration [48]. The third condition that requires particular attention is the patient who has a bidirectional superior cavopulmonary anastomosis. These patients rely on sufficient cerebral blood flow to provide venous return to the pulmonary arteries for optimal arterial oxygen saturation. In these patients hyperventilation is actually detrimental and hypoventilation (pCO2 = 50–55 mmHg) improves oxygenation. Because this is counterintuitive, the anesthesiologist must be aware of this curious physiology [49, 50]. Neurologic issues can occur in infants and young children with congenital cardiac defects [51]. A sign of neurologic involvement is evidenced by periventricular leukomalacia, which has been found to be present in 20% of infants presenting for surgery. The presence of periventricular leukomalacia increases to 50% after surgery requiring CPB [52]. Signs and symptoms in the child may not be evident early in life, but become more apparent on the entrance to school. Neuroprotection during CPB is a consideration for the anesthesiologist. Variables noted to possibly affect neurologic outcome include the use and length of time of deep hypothermic circulatory arrest (DHCA), the level of hemodilution, acid–base management at deep hypothermia, cerebral blood flow optimization, and the speed of rewarming [35]. Near‐infrared spectroscopy (NIRS) has recently been used to measure cerebral and somatic regional oxygen saturation. The NIRS probe uses a light source that directs light through bone and tissue, which is then sensed by a shallow and deep detector. The absorption data returned to the detectors reflect deoxygenated hemoglobin and total hemoglobin, from which regional (cerebral or somatic) oxygenation is calculated. Kussman and colleagues reported that perioperative periods of diminished cerebral oxygen delivery, as indicated by rSO(2) <45%, are associated with one‐year Psychomotor Development Index and brain MRI abnormalities among infants undergoing reparative heart surgery [53, 54]. At present methods believed to prevent brain injury include limited use of DHCA, monitoring and responding to regional cerebral perfusion (NIRS), achieving uniform cooling, utilizing pH stat arterial blood gas strategy, and recognizing that hyperglycemia is preferred over hypoglycemia. Raising the hematocrit to 30% during CPB appears to result in less neurologic injury [55, 56]. Pharmacologic intervention includes using volatile agents to increase cerebral blood flow and achieve more uniform cooling and rewarming. Neuroprotective pharmacotherapy that has been studied in an effort to reduce neurologic injury includes free radical scavengers, antiepileptics, erythropoietin, and anti‐inflammatory agents [57]. None has been proven unequivocally beneficial to date. An important challenge to the clinical management of potential adverse neurologic outcomes is the lack of accurate and specific biomarkers of neurologic injury. Development of these markers could potentially assist in the timely assessment of neurologic deficits and several studies are underway [58]. Blood loss after CPB continues to be a challenge and subject of debate. Before induction of anesthesia, a clear understanding of the desired hematocrit at the end of the procedure will allow adequate preparation of blood and blood products. In general terms for the patient having a two‐ventricle repair a hematocrit of 30% is adequate. For the patient with a functionally univentricular heart that is still mixing (e.g., Norwood, bidirectional Glenn) a hematocrit of 45% is optimal. After the Fontan procedure a hematocrit of 30–35% is acceptable. In younger patients it is more likely that blood loss will be greater and therefore a higher‐end hematocrit will be desired [59]. Consideration must be paid to the number of donor exposures each patient will experience. The use of blood products is associated with the potential transfer of pathogens and alloimmunization, both of which may affect the long‐term health of the patient [60]. Platelet count and function decreases are frequently encountered [61]. A rational plan for post‐CPB includes the use of an antifibrinolytic, followed by platelet infusion, cryoprecipitate to increase fibrinogen, and factor VII, in increasing order of problematic bleeding [62].
CHAPTER 6
Anesthesia for the Patient with Congenital Heart Disease
Demographics
Anesthetic Assessment
Anesthetic Management
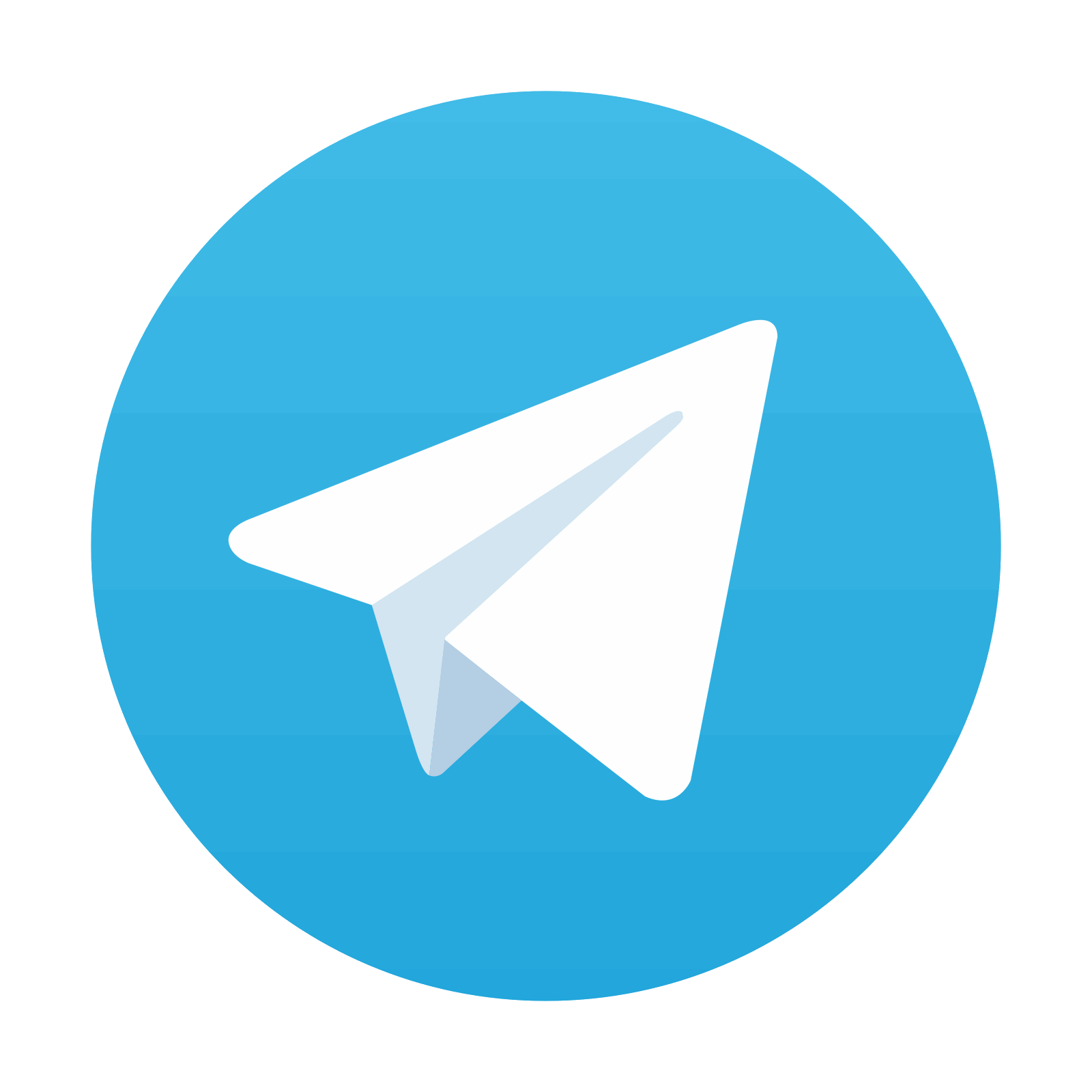
Stay updated, free articles. Join our Telegram channel

Full access? Get Clinical Tree
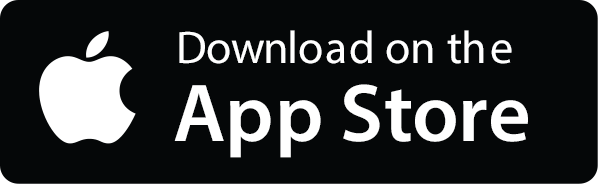
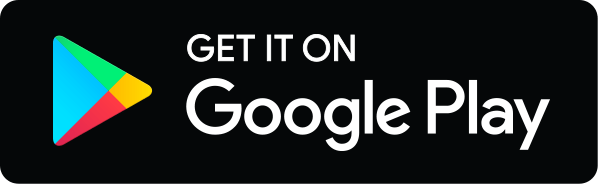