Mjaye L. Mazwi1, Carl L. Backer2, John M. Costello3, and Constantine Mavroudis4 1The Hospital for Sick Children, Toronto, Ontario, Canada 2UK HealthCare Kentucky Children’s Hospital, Lexington, KY, USA 3Medical University of South Carolina Children’s Hospital, Charleston, SC, USA 4Peyton Manning Children’s Hospital, Indianapolis, IN, USA Improved results after surgical treatment of congenital heart defects in recent years may in part be attributed to recognition of the importance of perioperative patient preparation and stabilization. Specific issues that warrant attention and management strategies used in the perioperative care of the infant and child differ considerably from those applied to adults in many important ways. Most importantly, the unique cardiac anatomy and physiology present in infants and children with complex congenital heart disease and the operations used to treat these lesions warrant nuanced and at times esoteric critical care management strategies. Additionally, noncardiac comorbidities present in children differ vastly from those encountered in adults [1]. The circulatory response to cardiopulmonary bypass (CPB) and the anatomy and physiology of organ systems vary with maturational change [2]. As a result of higher complication rates associated with invasive monitoring devices, the postoperative monitoring of infants and children emphasizes noninvasive techniques (e.g., physical examination, regional saturation monitors) when compared to the more routine invasive monitoring of adult cardiac surgery patients [3]. Perioperative care ideally involves a multidisciplinary team to facilitate optimal outcomes [4]. Iterations of the team typically include cardiovascular surgeons, cardiac intensivists, cardiologists, cardiac nurses, cardiac anesthesiologists, child life specialists, social workers, and respiratory therapists [4, 5]. Consultation should be available from other pediatric subspecialties to assist in the management of comorbidities and complications. The cumulative experience of the entire team likely contributes to the improved outcomes achieved by centers performing higher volumes of pediatric cardiac surgical procedures [6–8]. The experience of the bedside nurse is of particular importance [9, 10]. To consolidate this experience, many programs organize the care of all critically ill children with cardiac disease in dedicated cardiac intensive care units (ICUs) [11]. In this chapter, we have summarized general strategies for the critical care of infants and children undergoing cardiac surgery. Postoperative management strategies that may be useful following specific operations are discussed in other chapters dedicated to those operations. Optimizing operative outcomes begins with adequate preparation of the patient for surgery. For fetuses with critical congenital heart disease, antenatal diagnosis has been shown to reduce preoperative morbidity and mortality by facilitating delivery at a center with the ability to provide early, appropriate resuscitation and stabilization [12–15]. Additionally, antenatal diagnosis may facilitate fetal interventions in carefully selected cases, which have the potential to beneficially modify aspects of the anatomy and subsequent development of the heart [16, 17]. Children undergoing elective surgery should have a careful history and physical examination before the procedure. Operative approaches are determined by the nature of the cardiac defect, but are also influenced by the patient’s age, size, and preoperative clinical condition. The anatomic variability and complexity of congenital heart lesions require careful preoperative evaluation. All patients being considered for cardiac surgery should ideally undergo a multidisciplinary preoperative assessment (e.g., cardiac surgery, cardiology, anesthesia, and cardiac nursing) that involves a careful review of available data to ensure that they are adequate and that additional testing – such as echocardiography, computed tomography (CT), magnetic resonance imaging (MRI), or catheterization) – is not indicated. This assessment facilitates a careful review of indications, timing, and operative plan as well as a discussion of patient‐specific issues or potential complications. Any chronic noncardiac medical problems that require special attention or any acute medical illnesses that would warrant consideration for modification or cancellation of elective surgery should be identified [18]. Many programs perform this evaluation by means of a weekly combined medical–surgical conference. This conference structure has been identified as one of 21 important quality measures for pediatric cardiac surgery programs by the Society of Thoracic Surgeons and the Congenital Heart Surgeons’ Society (see Table 7.1) [19]. Most patients also undergo a routine preoperative screening evaluation that involves a chest x‐ray and laboratory testing (e.g., complete blood count, serum electrolytes, and when indicated coagulation panel). These tests are obtained to screen for abnormalities that could possibly require correction prior to surgery or special attention during surgery, such as anemia, hypokalemia, or bleeding disorders. In select cases, additional preoperative screening may be indicated in patients with underlying comorbidities. Elective cases in older children may not require such intensive preoperative laboratory evaluation [20, 21]. The psychological and emotional effect of intensive care on the child warrants attention. The foreign environment and limited availability of stimuli that help foster normal development may be challenging. Mental health and psychological wellbeing play a significant role in recovery from the acute insult of surgery. Emotional preparation of the child for surgery may facilitate a smooth recovery. Practical examples of this include providing the child with factual information concerning the surgery, predictions of what is likely to occur, and reassurance. In the postoperative period, keeping the parents involved in bedside care as much as feasible is likely to help attenuate some of this stress. Children undergoing cardiac surgery are at risk for developing posttraumatic stress disorder, especially if the ICU stay is prolonged [22]. In contrast to older children, the vast majority of neonates who are awaiting cardiac surgery are hospitalized in an ICU. Critical congenital heart defects may be broadly categorized as lesions with ductal‐dependent systemic blood flow, ductal‐dependent pulmonary blood flow, transposition physiology, or obstructed pulmonary venous return [23]. Historically, many neonates with critical congenital heart defects who presented with shock or severe cyanosis underwent emergent surgical intervention. However, the introduction of prostaglandin E1 (PGE1) infusion to maintain the patent arterial duct (thereby perpetuating the fetal circulation) in the 1970s has largely eliminated the need for emergency surgery [24]. With the exception of severely obstructed pulmonary venous return, the administration of PGE1 along with other supportive measures (e.g., judicious use of mechanical ventilation, diuretics, and inotropic support) typically allows surgery to be deferred for a few days while awaiting recovery of myocardial and other end‐organ function and a drop in the pulmonary vascular resistance (PVR). Side effects of PGE1 include apnea, hypotension, fever, and rash [24, 25]. When compared with spontaneous breathing, the elective initiation of mechanical ventilation for interhospital transport of neonates on PGE1 infusions is associated with a higher incidence of complications [25]. Thus, it is reasonable in many cases to transport neonates receiving PGE without securing the airway, provided that other indications aside from risk of apnea do not exist. A PGE1 dose of 0.01 μg/kg/min is typically effective for maintaining ductal patency, although higher doses are used in select situations, such as the neonate with critical congenital heart disease presenting in extremis after ductal closure. Aminophylline or caffeine may be safely used to minimize the occurrence of apnea in neonates receiving PGE1 [26]. Table 7.1 Quality measures for congenital and pediatric cardiac surgery. CPS, centrifugal pump system; EACTS, European Association for Cardio‐thoracic Surgery; ECMO, extracorporeal membrane oxygenation; IABP, intra‐aortic balloon pump; STS, Society of Thoracic Surgeons; VAD, ventricular assist device. Source: [19] / with permission of Sage Publication. Examples of lesions with ductal‐dependent systemic blood flow include hypoplastic left heart syndrome and interrupted aortic arch (Table 7.2). Neonates with these lesions develop shock, including myocardial dysfunction, metabolic acidosis, tachypnea, and hepatic and renal dysfunction as the arterial duct constricts. Systemic perfusion is further compromised as PVR falls, leading to preferential shunting to the pulmonary circulation and volume overload of the systemic ventricle. Preoperative management in neonates with ductal‐dependent systemic blood flow who present in shock is aimed at improving systemic perfusion, minimizing further declines in PVR, and supporting myocardial function. In addition to a PGE1 infusion, the judicious use of inotropes, afterload reduction with vasodilator therapy, and diuretics may be beneficial to support the volume‐overloaded systemic ventricle and augment systemic oxygen delivery (DO2). As shown by Barnea et al., augmentation of cardiac output allows a greater degree of imbalance between systemic (Qs) and pulmonary (Qp) blood flow to be tolerated with maintenance of adequate DO2 [27]. Augmentation of the hematocrit also increases arterial oxygen content (CaO2), which is one of the determinants of systemic oxygen delivery (DO2). Formulas commonly used in critical care, including those for DO2 and CaO2, may be found in Table 7.3. Therapies that further lower PVR, such as excessive supplemental oxygen or hyperventilation, should be avoided. Generous positive end‐expiratory pressure and relative hypoventilation may be employed with the goal of increasing PVR [28]. As an increase in blood viscosity results in a greater increase in PVR (Rp) when compared to systemic vascular resistance (Rs), transfusion to augment hematocrit is another strategy that may be useful in the overcirculated neonate [29]. The use of subambient inspired concentrations of oxygen (FiO2) may increase PVR and improve the Qp : Qs ratio. However, the use of subambient FiO2 has fallen out of favor as the associated diminished CaO2 results in a lack of improvement in systemic DO2 [30, 31]. Use of pharmacologic paralysis to minimize oxygen consumption (VO2) is also an option if the above measures fail to adequately improve DO2. Table 7.2 Ductal‐dependent congenital heart defects. Table 7.3 Commonly used formulae in cardiac critical care. BSA, body surface area; cl, chloride; ΔP, change in pressure; Hgb, hemoglobin; HCO3, bicarbonate; Na, sodium; Patm, atmospheric pressure; PH2O, vapor pressure; Qp : Qs, pulmonary flow : systemic flow; RQ, respiratory quotient (usually assumed to be ∼0.8). Neonates with hypoplastic left heart syndrome and a severely restrictive or intact atrial septum are at high risk for mortality due to the severity of the heart defect, abnormalities in pulmonary vascular and lymphatic development, and late development of pulmonary vein stenosis [32]. Acute stabilization may be achieved by fetal intervention or emergent creation of an atrial septal defect in the catheterization laboratory postnatally, followed by stage 1 Norwood palliation once pulmonary congestion has improved. Neonates with ductal‐dependent systemic blood flow are at risk for developing necrotizing enterocolitis (NEC) [33]. Historically, many clinicians were reluctant to provide enteral nutrition before surgery. However, evolving data suggest that many neonates with ductal‐dependent systemic blood flow may safely receive and potentially benefit from cautious enteral feeding prior to surgery [34, 35]. Ductal‐dependent pulmonary blood flow is present in neonates with severe or complete right ventricular outflow tract obstruction (Table 7.2). These patients present with progressive cyanosis as the arterial duct constricts. A PGE1 infusion will reliably reopen the arterial duct and improve systemic oxygen saturation levels [36]. In comparison to the ductal morphology in neonates with ductal‐dependent systemic blood flow, the arterial duct in neonates with ductal‐dependent pulmonary blood flow is typically longer and more tortuous, providing increased resistance that usually limits pulmonary overcirculation. Such patients generally do not require interventions to balance the circulation and augment DO2, and are more likely to tolerate enteral nutrition while awaiting surgery [34]. In patients with d‐transposition of the great vessels, the aorta arises from the right ventricle and the pulmonary artery arises from the left ventricle. Neonates with transposition physiology are dependent on mixing of oxygenated and deoxygenated blood at the arterial duct and through any atrial or ventricular septal defects (Table 7.2). Such mixing represents the “effective” systemic and pulmonary blood flow. In patients with restrictive septal communications, marked cyanosis may be present at birth or develop with constriction of the arterial duct. PGE1 is initiated when this diagnosis is suspected. An emergent balloon atrial septostomy (BAS) facilitates mixing at the atrial level and typically improves systemic oxygenation [37]. Additional benefits of a BAS include a reduction in left atrial pressure, which may facilitate a drop in PVR and the option to discontinue the PGE1 infusion and thus avoid its associated side effects. For these reasons, unless a sizable secundum atrial septal defect is present, some centers perform an elective BAS in nearly all patients with d‐transposition of the great vessels. In neonates with total anomalous pulmonary venous return, the pulmonary veins typically drain to the innominate vein, the coronary sinus, or the inferior caval vein, resulting in complete mixing of systemic and pulmonary venous return in the right atrium. A subset of patients with total anomalous pulmonary venous return have obstruction of the pulmonary venous pathway and may present with profound cyanosis within minutes to hours of birth. Neonates with severely obstructed total anomalous pulmonary venous return do not have sustained improvement with medical therapies and should undergo emergent surgical repair. Neonates with critical congenital heart defects are at increased risk for noncardiac comorbidities. Major noncardiac structural anomalies (e.g., omphalocele, tracheoesophageal fistula, renal anomalies) are found in approximately 15% of neonates with congenital heart disease [38, 39]. A recent report from the Society of Thoracic Surgeons Congenital Heart Surgery Database indicates that nearly 20% of neonates undergoing cardiac surgery have a major noncardiac anomaly or genetic syndrome, and that rates of these anomalies vary widely among patients with different types of heart defects [1]. While some clinicians advocate for universal screening, knowledge of associations between specific heart defects and various noncardiac anomalies and genetic syndromes may allow for a more parsimonious approach to testing [40]. Neonates with specific congenital heart defects that are associated with syndromes warrant genetic evaluation and counseling (e.g., interrupted aortic arch and DiGeorge syndrome; atrioventricular septal defect and trisomy 21) [1]. Genetic testing is not done to exclude patients from surgery, but rather to help anticipate comorbidities, plan the postoperative care, and facilitate counseling for future pregnancies [41]. Children with complex congenital heart disease who undergo cardiac surgery in infancy are at increased risk for adverse neurodevelopmental outcomes. Recent literature indicates that the prevalence of central nervous system abnormalities before surgery in newborns with congenital heart defects has been underappreciated [42, 43]. Preoperative brain MRI examinations obtained in research protocols in term newborns with transposition or single‐ventricle physiology reveal anatomic and functional features seen in premature neonates, suggesting abnormal brain development in utero [43, 44]. Licht and colleagues have shown that preoperative cerebral blood flow is diminished in neonates with severe congenital heart defects and progressively decreases until surgical correction, re‐emphasizing the importance of the assessment of the optimal time to perform surgery [45, 46]. Microcephaly, periventricular leukomalacia, or strokes are seen in approximately one‐third of preoperative neonates with complex congenital heart defects [47]. The long‐term implications of these findings are the subject of ongoing investigations, but they likely contribute to the suboptimal late neurodevelopmental outcomes experienced by some neonates with complex congenital heart disease [48]. In neonates who were premature, had a difficult delivery, or presented in shock or with severe cyanosis, an ultrasound of the brain should be obtained to evaluate for intraventricular hemorrhage or other intracranial abnormalities. In stable neonates who lack these comorbid‐presenting factors, however, the yield of a screening brain ultrasound is extremely low [49]. Neonates with congenital heart defects are nearly twice as likely as babies without birth defects to be born prematurely, at low birth weight, and small for gestational age [50–52]. Neonates with critical congenital heart disease who are born prior to 39 weeks’ gestation are at increased risk for morbidity, mortality, and longer hospitalization [53–55]. The care of these patients may be complicated by any of the problems associated with prematurity, including respiratory distress syndrome, NEC, and intraventricular hemorrhage. The musculature in the pulmonary arterioles is less well developed, predisposing patients with left‐to‐right shunting to a rapid decline in PVR and overcirculation. In premature and/or low birth weight neonates, the timing of surgical or transcatheter intervention is controversial. Some clinicians advocate for early intervention rather than a strategy of growth and delayed intervention, although convincing data demonstrating that either approach leads to improved outcomes are lacking [56–58]. The monitoring devices used in the postoperative period are selected based upon the patient’s age, heart defect, and anticipated postoperative course. Information derived from monitoring devices is extremely useful, but is only reliable when obtained from appropriately calibrated instruments. In addition, the information obtained must be interpreted in the context of known normal values for patient age and what is known about the patient’s physiology (see Table 7.4) [59]. Table 7.4 Ranges of heart rate during sinus rhythm in normally convalescing patients after open‐heart surgery. Source: Adapted from [59]. The electrocardiogram (ECG) leads should be placed on the chest and limbs so that the patient’s heart rate, rhythm, and ST segments can be monitored. The leads should be placed away from the area to be prepped and not too close to the electrocautery‐grounding pad. Perioperative monitoring of a child’s temperature is crucially important to ensure adequate end‐organ protection and avoid hyperthermia and associated increased VO2. The high systemic vascular resistance of a very hypothermic child can prevent successful weaning from CPB. Typically both the esophageal and rectal or bladder temperatures are monitored in all open‐heart cases. The use of forced‐air warming blankets in the operating room has been very successful in warming patients after hypothermic CPB and keeping them warm in a cold operating room [60]. Monitoring the patient’s oxygen saturation on a continuous basis is the “standard of care” for all infants and children undergoing cardiac surgery [61]. Pulse oximetry is simple to use, noninvasive, and relays continuous extraordinarily vital and reliable information. The LED readout displays the child’s peripheral oxygen saturation at the same time as audible tones are emitted that are synchronized to the heart rate and saturation percentage. The saturation monitor also gives some information about the patient’s cardiac output. If the saturation monitor cannot pick up the pulse, the child is either cold and peripherally vasoconstricted or the cardiac output is not sufficient to generate a recognizable pulse waveform – a grave sign. The saturation probe should be used pre‐, intra‐, and postoperatively, and is especially valuable during transport. An indwelling arterial catheter is necessary for all open‐heart operations and most major closed‐heart cases. It permits continuous monitoring of the systemic arterial pressure and easy sampling for determination of arterial blood gases and other laboratory parameters. An appropriately sized plastic angiocath can be inserted into the artery being used for monitoring percutaneously or by a surgical cutdown. In the newborn, the umbilical artery is an alternative site for monitoring using an umbilical arterial line. Adequate intravenous (IV) access should be obtained before beginning any major cardiac procedure. Central venous access is needed for complex procedures and in most neonates to monitor central venous pressure and administer medications (see Table 7.5) [62]. Percutaneous catheters may be placed in the subclavian, internal jugular, or femoral veins. Complications occasionally occur during central venous line insertion and maintenance including bleeding, inadvertent arterial injury, thrombosis, and infection [63]. Adult studies have found that the use of ultrasound guidance may increase the rate of successful line placement and minimize complications during placement of central lines, although findings from well‐designed pediatric studies are less convincing [64, 65]. Although thrombotic complications related to central venous lines occur in up to one‐third of pediatric cardiac patients, the use of empiric low‐dose heparin infusion has not been shown to be beneficial [66, 67]. Table 7.5 Normal ranges of intracardiac and intravascular pressures (in mmHg). A, a‐wave; v, v‐wave. Source: Adapted from [62]. Measurement of venous O2 saturation in the superior caval vein approximates mixed O2 venous saturation (SvO2) and is useful for estimating cardiac output. In neonates recovering from single‐ventricle palliation, venous O2 saturation will also aid in the calculation of the ratio of pulmonary to systemic blood flow. Postoperative management centered on maximizing SvO2 has been associated with improved survival following stage 1 Norwood palliation in some centers [68]. Thus, care should be taken to select the length of a percutaneous interval jugular or subclavian vein catheter such that the tip will be positioned in the superior caval vein. Continuous assessment of SVO2 saturation is now feasible following congenital heart surgery using oximetric catheters [69]. Right and left atrial lines may be placed directly at the conclusion of CPB and are useful for measuring central venous pressure, left atrial pressure, and as a reliable long‐term source of venous access following complex procedures [70–72]. Left atrial lines may be particularly helpful in the assessment of the circulatory adequacy of small left‐sided structures after attempts at rehabilitation of the left heart. A specific complication when using these lines is unrecognized migration of the catheter out of the atrium and into the pericardial space, which causes infusion of IV fluids and/or hyperalimentation into the pericardium and an insidious progressive cardiac tamponade. Bleeding from the atrium at the time of catheter removal may also occur, which can cause cardiac tamponade that may warrant emergency mediastinal exploration. With left atrial lines (or any IV line in a patient with residual right‐to‐left shunting), meticulous attention must be paid to avoiding the introduction of any air or particulate matter into the line that could cause coronary or cerebral arterial embolization. The left atrial line should not be used for routine administration of fluid or medications and, if obstructed, should not be flushed. The atrial lines should be removed with precautions for bleeding, including consideration for sedation so that the child does not cough or cry, ensuring there are no clotting abnormalities, consideration for having blood available, and timing the line removal when surgeons are available in the event that mediastinal exploration is needed. For patients with marginal postoperative cardiac output we have often left the sternum open, sealing the chest with a silastic patch. A left atrial line can be used until the time of chest closure. Pulmonary artery catheters are rarely used in the current era following congenital heart surgery. Instead, transthoracic echocardiograms are more commonly used to obtain an intermittent, noninvasive assessment of pulmonary artery pressures. Potential relative indications for placement of pulmonary artery catheters include patients with an elevated preoperative PVR or those in whom residual left‐to‐right shunting is anticipated (e.g., multiple muscular ventricular septal defects). A flow‐directed Swan–Ganz catheter may be passed through either the subclavian vein or internal jugular vein into the pulmonary artery. Alternatively, the catheter can be placed directly into the pulmonary artery through the right ventricular outflow tract or through the right atrium at the time of surgery. The pulmonary artery catheter can be used for measurement of pulmonary capillary wedge pressure (PCWp), pulmonary artery pressure (Pap) and cardiac output (CO), or for quantification of residual left‐to‐right shunting. If placed directly through the right ventricle free wall, the pulmonary artery catheter is often removed 48–72 hours after the procedure, while the mediastinal chest tubes are still in place and while observing similar precautions for bleeding as with the atrial lines. Near‐infrared spectroscopy (NIRS) is a noninvasive monitoring technique providing real‐time venous‐weighted regional oxygen saturation levels. Used as a trend monitor, NIRS values provide validated, surrogate measures of cardiac output in pediatric patients during and after cardiac surgery [73]. The specific value of NIRS monitoring is the ability to obtain continuous, noninvasive, real‐time information on the regional oxygen content that reflects both oxygen delivery and consumption. Alterations in regional somatic oxygen saturation reflect local perfusion changes and may be an earlier, more sensitive indicator of evolving low cardiac output [74]. Figure 7.1 Real‐time near‐infrared spectroscopy (NIRS) graph showing 2.5 hours of data that demonstrate acute decrease in rSO2 associated with chest closure in a newborn patient with hypoplastic left heart syndrome and resultant improvement with fluid resuscitation and increased inotropic support. Source: [75] / with permission of Elsevier. Early recognition of changes in NIRS values may facilitate timely, critical interventions in the care of patients in both the operating room and the ICU. Changes in the NIRS monitoring trends often occur before or in the absence of any other physiologic changes (Figure 7.1) and responding to the trend data can have a positive impact on patient outcomes [75]. Most centers now use two‐site NIRS monitoring – cerebral and somatic – routinely while the patient is critically ill. Temporary pacing wires have diagnostic and therapeutic utility in complex pediatric cardiac surgical patients [76]. The routine placement of temporary pacing wires is controversial and practice varies among centers [77–79]. Atrial electrograms may be directly recorded in patients in whom traditional surface electrocardiograms are nondiagnostic. Temporary pacing may be readily employed in patients with sinus node dysfunction or to restore atrioventricular synchrony in patients with junctional ectopic tachycardia or complete heart block. Burst pacing may be used to terminate re‐entrant arrhythmias. Temporary pacemaker wires are anchored to the epicardium with fine sutures that are placed superficially to facilitate their subsequent removal with minimal risk of hemorrhage. Wires can be placed with either a unipolar configuration or a bipolar configuration (two separate wires on the atrium or a single wire with two electrodes). The sensitivity and capture threshold of temporary pacing wires should be assessed daily in patients being paced, as wires that sense poorly increase the likelihood of inappropriate pacing and pacemaker‐induced arrhythmia. A high capture threshold warns of impending wire failure. Complications associated with these wires are rare, but include tamponade with removal and retention [77]. Drainage catheters, appropriate to patient size, are left in place after all open and some closed procedures. Chest tubes are not necessarily placed after simple patent arterial duct ligation or vascular ring division. There is a trend of centers moving away from using rigid thoracotomy tubes with pleuravac drainage to using Blake drains with soft bulb suction [80]. For a larger child, in whom neither pleural space has been entered, a single mediastinal drainage tube is sufficient. When the pleural cavities are entered, they are drained separately with pleural drains. In small infants, or when there is myocardial swelling, care should be taken to insure that the mediastinal drainage tube does not cause coronary artery or cardiac compression. The amount of blood lost through the chest tube(s) should be carefully recorded on an hourly basis. The stomach should be routinely decompressed postoperatively with a nasogastric tube in intubated patients. Gastric decompression will minimize respiratory embarrassment secondary to abdominal competition from gastric distention as well as vomiting and potential tracheobronchial aspiration. The nasogastric tube is generally removed when the endotracheal tube is removed. A urinary catheter is typically placed in infants and children undergoing an open cardiac procedure or a complicated closed‐heart procedure. Hourly urine output is an excellent indicator of the adequacy of renal perfusion and cardiac output. The urinary output should generally be at least 1 mL/kg/h in the child with an adequate cardiac output and preload, although lower volumes are sometimes seen during the first night after a complex operation. The use of urinary catheters with a temperature sensor allows avoidance of a separate temperature probe. CPB is associated with a systemic inflammatory response that contributes to dysfunction of multiple organ systems and prolongs postoperative recovery. The routine use of empiric corticosteroids during pediatric CPB cases is controversial. A number of studies have found that corticosteroids attenuate the post‐bypass inflammatory response, as assessed by cytokine levels and other inflammatory markers [81, 82]. Given these data, some form of empiric corticosteroid administration before CPB became commonplace in most pediatric cardiac surgical centers [83]. However, in a recent observational study of children undergoing congenital heart surgery whose data were in the Pediatric Health Information Systems Database, investigators were unable to demonstrate a significant benefit associated with empiric corticosteroids and found that their use may be associated with increased morbidity, particularly in lower‐risk patients [84]. Another observational study using data from the Society of Thoracic Surgeons Congenital Heart Surgery Database found that empiric corticosteroids are not associated with improved clinical outcomes and may increase the risk of infection in neonates [85]. The results of an ongoing placebo‐controlled trial of empiric corticosteroids in neonates undergoing cardiac surgery requiring CPB may be informative (Corticosteroid Therapy in Neonates undergoing Cardiopulmonary Bypass, NCT01579513; http://clinicaltrials.gov). Following the procedure, the patient must be carefully monitored and transported to the ICU. Transportation is facilitated with a portable ECG and arterial blood pressure monitor along with an oxygen saturation monitor. Oxygen and emergency airway equipment are required for all patients. It is important to avoid hypothermia during transport. Upon arrival to the ICU, the sequence of subsequent care for the child is important and should begin with attention to the airway and ventilation, followed by the cardiovascular system, and then the various subsystems. While the child is being attached to the ICU’s monitoring systems, all staff should observe the patient constantly for signs of difficulties that might require alteration in the normal sequence of events. Utilization of a formal transition process, derived from Formula One pit stop and aviation models, has been associated with a reduction in technical errors and handover omissions [86, 87]. We have used a formal handoff template delivered in a “sterile cockpit” environment [88]. The surgeon provides a detailed description of the operative findings and repair, the CPB, cross‐clamp and circulatory arrest times, an assessment of myocardial function coming off bypass, vital signs, loading conditions, and any pressure or oxygen saturation measurements or transesophageal echocardiogram findings. The anesthesia team conveys relevant data about vascular access, the airway, and lung function, the anesthetic, and important laboratory values. Maintaining airway patency and ensuring adequate oxygenation and ventilation are priorities for all patients. Physical examination of the patient immediately upon arrival in the ICU can reveal clinical signs of desaturation, including perioral and/or peripheral cyanosis. Careful inspection for symmetry and adequacy of chest wall expansion as well as auscultation for bilateral breath sounds should be performed. Other signs of respiratory insufficiency in spontaneously breathing patients include tachypnea, retractions, nasal flaring, grunting, and apnea. A portable chest x‐ray should be obtained to evaluate for the presence of a pneumothorax, hemothorax, and/or atelectasis, as well as to confirm the position of the endotracheal tube (ideally in the middle third of the trachea), intravascular lines, and nasogastric tube. It is common practice to obtain daily portable chest radiographs for all intubated patients [89]. Continuous pulse oximetry is used in all patients along with continuous end‐tidal CO2 monitoring. An arterial blood gas is obtained to assess adequacy of gas exchange. Patients who are extubated prior to leaving the operating room are usually placed on supplemental oxygen via nasal cannula or face mask. The initial arterial blood gas of the child who is extubated frequently shows mild CO2 retention. In the usual case, as the residual effects of anesthesia wear off and the patient is less hypopneic, the PaCO2 will normalize. In the event that hypercarbia worsens, or if the child should start to develop signs of respiratory insufficiency, noninvasive ventilation (e.g., continuous positive airway pressure [CPAP] or bilevel positive airway pressure) or reintubation should be considered. Inadequate reversal of muscle relaxants and/or the sustained effects of narcotics are two situations that are easily treated. Evidence of acute upper‐airway edema may be treated with nebulized racemic epinephrine and dexamethasone. The care of patients who return to the ICU intubated is more complicated. Ensuring a smooth postoperative course requires a thorough understanding of cardiopulmonary interactions. Positive‐pressure ventilation and alterations in respiratory mechanics can have a profound effect on hemodynamics, particularly following repair of complex congenital heart defects (see Figure 7.2) [90]. Changes in intrathoracic pressure affect systemic and pulmonary venous return, right ventricular ejection, and left ventricular afterload. Appreciation of the unique effects of cardiopulmonary interactions on circulatory physiology following complex congenital heart procedures is essential when selecting ventilator strategy [91]. It is important that the ICU physician be present at the time of initiation of ventilator support. “Routine” settings do not exist and an assessment should be undertaken with specific gas exchange targets in mind for each individual patient. Operating room ventilator and tubing characteristics may differ from those used in the ICU, and thus different settings may be needed in the ICU when compared with those used in the operating room to obtain similar parameters of gas exchange. The presence of a large leak around the endotracheal tube, pleural effusions, chest wall edema, or abdominal distention can dramatically affect the adequacy of delivered breaths. Careful observation and monitoring are critical during this transition phase. The usual starting tidal volumes are approximately 8–10 cc/kg, and the ventilator rate varies with age. A pressure‐ or volume‐limited mode of ventilation may be used; with either mode, serial blood gases are obtained to monitor the effect of ventilation along with pulse oximetry and an end‐tidal CO2 monitor. Sedation and analgesia, as well as avoidance of patient/ventilator asynchrony and prevention of pulmonary hypertensive crisis, may all be achieved by continuous or intermittent IV infusion of dissociative agents and narcotics. The importance of patient/ventilator synchrony cannot be overemphasized. Patient ventilator dysynchrony may be associated with ventilator‐induced lung‐injury (VILI), longer mechanical ventilator duration, adverse cardiopulmonary interactions, and patient discomfort requiring escalation of sedatives [92]. Figure 7.2 Illustration of the left ventricle, thoracic cavity, and aorta. Similar changes in the left ventricular systolic transmural pressure (PTM) can be generated by manipulating either aortic pressure or intrathoracic pressure (ITP). When negative ITPs are exaggerated, as occurs in respiratory disease, PTM increases significantly. PPV, positive‐pressure ventilation. Source: [90] / with permission of Wolters Kluwer Health Inc. The ventilator strategy should be customized based on each patient’s preoperative state and anticipated postoperative physiology. For example, in children who have or are at risk for postoperative pulmonary hypertension, ventilator settings are selected to achieve a pH of 7.40–7.45 and avoid hypoxia. Strong consideration should be given to the early use of inhaled nitric oxide for the treatment of pulmonary hypertension [93]. In contrast, after a superior cavopulmonary anastomosis (bidirectional Glenn), hyperventilation may decrease cerebral blood flow, with subsequent decreased blood flow through the superior caval vein to the pulmonary arteries leading to hypoxia, and inhaled nitric oxide is generally not effective [94, 95]. Therefore, in such patients hyperventilation is avoided and mild hypoventilation (PCO2 48–55 mmHg) may result in optimal pulmonary blood flow and systemic oxygenation [96]. Following both the Glenn and Fontan procedures, the goal is to achieve target gas exchange at the lowest mean airway pressure (MAWp) possible to facilitate passive pulmonary blood flow and aim for early extubation [97]. Most centers are now successfully using protocols that allow early extubation in carefully selected patients [98–100]. Multicenter studies indicate that early extubation following some operations is feasible, relatively safe, and may be associated with a reduction in postoperative length of stay [101, 102]. Interventions of this sort are important in an era where new metrics like value‐based care (i.e., cost : outcome ratio) are beginning to reshape healthcare [103]. Considerations around extubation include postextubation airway edema and potential vocal cord dysfunction, both of which may cause upper‐airway obstruction. The high negative intrathoracic pressure generated during spontaneous breathing with upper‐airway obstruction creates increased afterload that may be poorly tolerated by the patient with marginal ventricular function. To minimize airway swelling in infants, IV dexamethasone may be administered prior to extubation, although published data do not completely support the efficacy of this practice [104]. Treatment with nebulized racemic epinephrine is also transiently effective in reducing airway edema. Patients should be closely observed for tachyarrhythmias during these treatments and for rebound subglottic edema. Assessment and management of factors that impact systemic DO2 and VO2 are essential in the immediate postoperative period. Close attention to optimal myocardial loading conditions, particularly after complex cases, is important. Hypovolemia may occur secondary to changes in the venous capacitance or through protein and fluid leakage into the interstitial, peritoneal, or pleural spaces. Blood loss through the chest tubes may warrant replacement during the initial several hours to prevent anemia, hypovolemia, and inadequate preload. Treatment includes replacement of the sequestered volume by appropriate amounts of crystalloid, blood, or colloid solution. The standard fluid bolus that is administered is 5–10 mL/kg over 5–10 minutes. Patients who have had single‐ventricle palliation and are still cyanotic typically require a more generous hematocrit than biventricular circulations for adequate systemic DO2. Care should be taken to avoid overtransfusion, as severe polycythemia may increase systemic and PVR and interfere with capillary perfusion. Ongoing assessment and management of temperature are important given its impact on VO2 and systemic vascular resistance. Patients who are hypothermic upon admission to the ICU may be peripherally vasoconstricted, and may be hypovolemic in spite of normal systemic arterial and atrial filling pressures. In addition, hypothermia impairs platelet function and can promote bleeding [105]. In these patients arterial hypotension should be prevented by adequate volume replacement as the body temperature returns to normal and the peripheral vascular bed dilates. Mild postoperative hypothermia is readily corrected in older children by use of warm blankets and in infants by means of overhead radiant heaters. Hyperthermia increases cardiac workload and oxygen consumption and may lower the threshold for seizure activity and junctional ectopic tachycardia (JET) in vulnerable patients. Therefore, hyperthermia should be treated early and aggressively with antipyretics (acetaminophen, ibuprofen) and/or surface cooling. An initial 30–40 mg/kg loading dose of acetaminophen is more effective in reducing fever than a 15 mg/kg maintenance dose [106]. For children who do not follow the anticipated course following cardiac surgery, early consideration must be given to the completeness of the preoperative diagnosis and the adequacy of the surgical repair. Data from the Society of Thoracic Surgeons Congenital Heart Surgery Database indicate that unplanned cardiac reinterventions during the same hospitalization as the index operation are performed in 11.8% of neonates, 5.2% of infants, and 2.8% of children [107]. These unplanned reinterventions are associated with substantial mortality and resource utilization [107, 108]. Evaluation begins with an echocardiogram. Cardiac computed tomography angiogram (CTA) and/or catheterization may be safely performed early after surgery and often will identify residual lesions that are amenable to intervention [109, 110]. The assessment and management of fluid balance are an essential component of early postoperative management [111, 112]. Although there is a tendency for sodium and free water retention following CPB, it is routine to administer fluid therapy after cardiac surgery to help prevent intravascular hypovolemia. There is significant variation among centers in postoperative fluid management strategy from restrictive protocols (50 mL/kg/24 h) up to standard maintenance. The amount and composition of IV fluids may vary depending on the clinical situation and the serum electrolyte levels. Increasingly, with the exception of neonates, there is a trend in critical care medicine toward more restrictive use of isotonic fluids as morbidity associated with fluid overload is more widely appreciated [113]. Diuretics are typically prescribed postoperatively to assist in fluid removal. Plasma electrolyte levels should be closely monitored and corrected when needed. Although hypokalemia is known to contribute to ventricular irritability, especially for patients receiving digoxin, this is not as significant a problem in pediatric patients as in adults. Hypokalemia should be treated with supplemental IV potassium (0.5–1.0 mEq/kg over 2 h) to keep the serum potassium at a normal level. However, care should be taken not to administer the potassium too fast or in too concentrated an infusion, or to administer liquid enteral potassium in individual doses exceeding ∼1 mEq/kg, as either may cause a hyperkalemic cardiac arrest. Hyperkalemia can also occur, usually in the context of postoperative renal dysfunction. Hyperkalemia should be treated by removing all potassium from the IV fluids, and may require oral or rectal sodium polystyrene sulfonate, 1 g/kg every 4 h. Should hyperkalemia persist, the patient may be treated with either peritoneal or hemodialysis. Serum magnesium levels should also be monitored and corrected when needed to decrease arrhythmia risk. Sodium disturbances occasionally occur postoperatively. Mild dilutional hyponatremia is often present and usually requires no treatment other than diuretic administration and mild restriction of free water intake. Serum sodium levels below 125 mEq/L may be associated with neurologic symptoms and seizures and should be treated by water restriction and cautious administration of normal saline or 3% normal saline. Hypernatremia is much less common and usually occurs in patients in renal failure or in infants who have received large amounts of sodium bicarbonate. Hypernatremia should be treated by sodium restriction and liberalization of free water intake. Maintaining normocalcemia is important for cardiovascular function. Hypocalcemia is seen mainly in neonates and after transfusion of large amounts of blood products. Myocardial function in neonates is particularly dependent on adequate calcium levels as a result of immaturity of the sarcoplasmic reticulum [114]. Serum calcium is measured frequently and maintained within normal limits by IV administration of calcium gluconate or calcium chloride. Metabolic acidosis is occasionally seen postoperatively, particularly after complex cases. Metabolic acidosis frequently results from hyperchloremia as a result of fluid administration and ultrafiltration during surgery. However, the most clinically concerning etiology of metabolic acidosis is decreased cardiac output and impaired tissue perfusion resulting in lactic acid production [115, 116]. Treatment often involves simultaneous correction of the underlying hemodynamic disorder and IV administration of sodium bicarbonate (1 mEq/kg per dose). Rapid infusion of sodium bicarbonate has been associated with intraventricular hemorrhage in neonates. Since the buffering action of bicarbonate will result in the formation of carbon dioxide, it is important that the patient’s ventilator status be optimized prior to administration. Other causes of metabolic acidosis include renal tubular acidosis, NEC, sepsis, hepatic injury, metabolic syndromes, and intraventricular hemorrhage [116]. The management of hyperglycemia after cardiac surgery in adults and children has been the subject of intense investigation in recent years. In critically ill adults, including those recovering from cardiac surgery, multiple studies have found associations between hyperglycemia and adverse outcomes. In a single‐center randomized clinical trial in critically ill adults, the majority of whom were recovering from cardiac surgery, Van den Berghe and colleagues found that strict glycemic control reduced mortality and improved end‐organ function [117]. However, recent multicenter trials designed to replicate these findings have either been stopped early due to excessive hypoglycemia or have found that glycemic control was associated with increased mortality [118]. In pediatric cardiac surgical patients, observational studies have found that hyperglycemia is common after cardiac surgery and associated with adverse early postoperative outcomes [119–122]. In a single‐center randomized trial involving 700 critically ill children, the majority of whom were recovering from cardiac surgery, patients assigned to the strict glycemic control group had reduced markers of inflammation, shorter ICU length of stay, and lower mortality (3% vs. 6%) [123]. However, two recent, large multicenter randomized trials of tight glycemic control failed to replicate these findings [124, 125]. Current data do not support tight glycemic control following pediatric cardiac surgery.
CHAPTER 7
Perioperative Care
Preoperative Care
Specific Considerations for the Neonate
Ductal‐Dependent Systemic Blood Flow
For pulmonary blood flow
For mixing
For systemic blood flow
Poiseuille’s equation
Flow = [(ΔP) × π × radius4] / [8 × viscosity × length]
Resistance to flow
= viscosity × length/radius4
Cardiac output (CO)
= stroke volume (SV) × heart rate (HR)
Arterial oxygen content (CaO2)
= (Hgb × 1.36 × SaO2) + (0.003 × PaO2)
Oxygen delivery (DO2)
= CO × CaO2
Oxygen consumption (VO2)
= (CaO2 – CvO2) × CO
Qp : Qs
= [Sat(aorta) – Sat(mixed venous)] / [Sat(pulmonary venous) – Sat(pulmonary artery)]
Mean arterial pressure (MAP)
= [Systolic blood pressure + 2(diastolic blood pressure)] / 3
Oxygenation index (OI)
= [Mean airway pressure × FiO2 ]/ [PaO2 × 100]
Glucose infusion rate (GIR)
= [% dextrose × rate (mL/h)] / [6 × weight (kg)]
Anion gap
= Na – Cl – HCO3
Creatinine clearance
= [urine Cr (mg/dL) × urine volume (mL) × 1.73] / [serum Cr (mg/dL) × duration of collection (minutes) × BSA]
Ductal‐Dependent Pulmonary Blood Flow
Transposition Physiology
Total Anomalous Pulmonary Venous Return
Noncardiac Anomalies
Operative Care
Age
Heart rate (beats/min)
0–1 month
120–190
1–6 months
110–180
6–12 months
100–170
1–3 years
90–160
3–6 years
80–150
6–15 years
80–140
>15 years
70–130
Electrocardiogram Leads
Temperature Monitoring
Oxygen Saturation Probe
Arterial Catheter
Percutaneous Venous Catheters
Infants and children
Newborn period
Right atrium
a = 3–7; v = 2–5; mean = 1–5
mean = 0–3
Right ventricle
15–30/2–5
35–65/1–5
Pulmonary artery
15–30/5–10 (mean = 10–20)
35–65/20–40 (mean = 25–40)
Pulmonary wedge
a = 3–7; v = 5–15; mean = 5–12
Left atrium
a = 3–7; v = 5–15; mean = 5–10
mean = 1–4
Left ventricle
80–130/5–10
Atrial Catheters
Pulmonary Artery Catheter
Near‐Infrared Spectroscopy
Pacemaker Wires
Chest Tubes
Nasogastric Tube
Urinary Catheter
Empiric Corticosteroids
Postoperative Care
Transport to the Intensive Care Unit and Handoff
Airway and Breathing
Cardiovascular System
Fluid and Electrolyte Management
Hyperglycemia
Renal Function
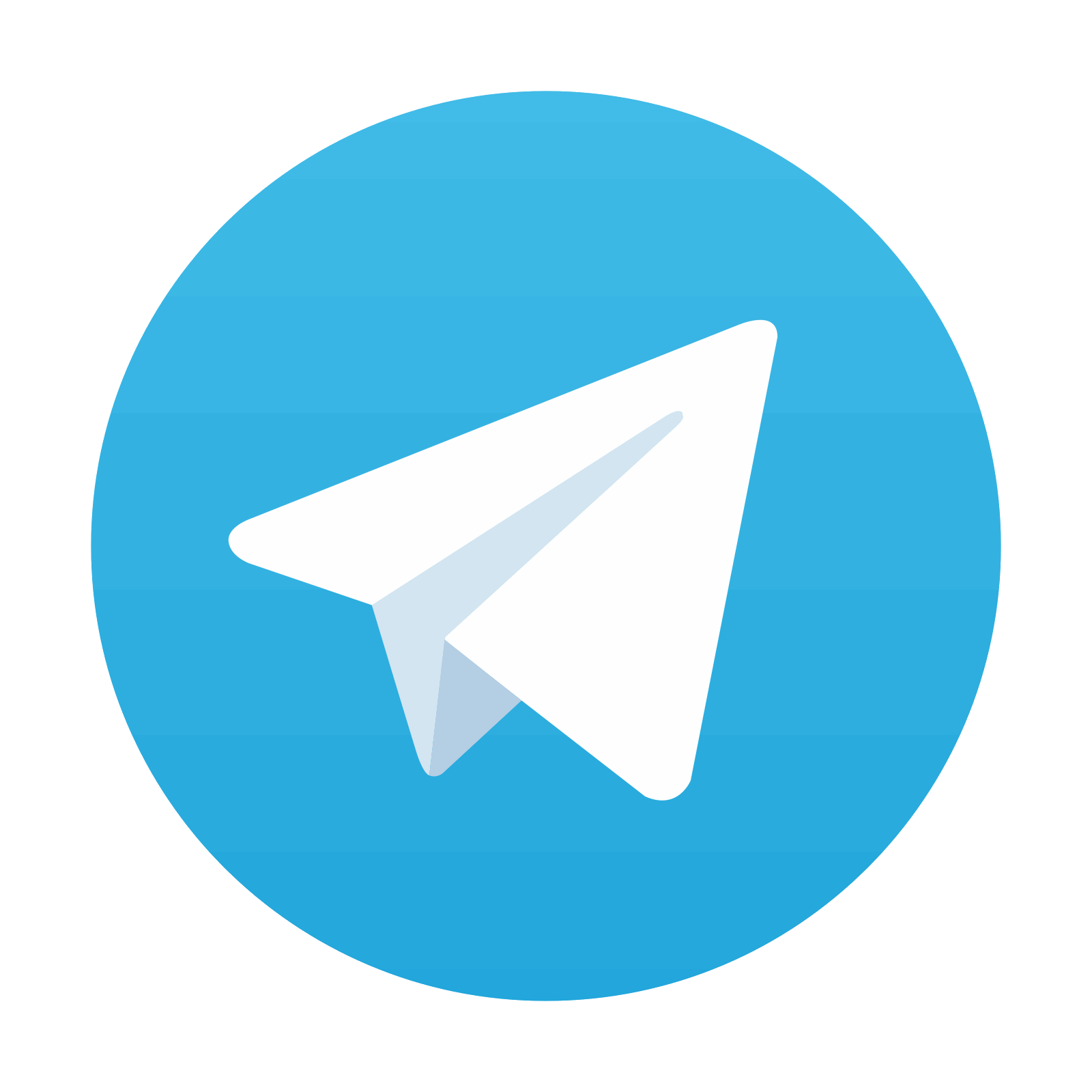
Stay updated, free articles. Join our Telegram channel

Full access? Get Clinical Tree
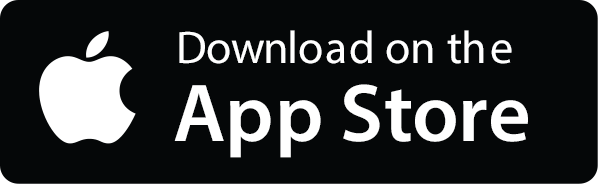
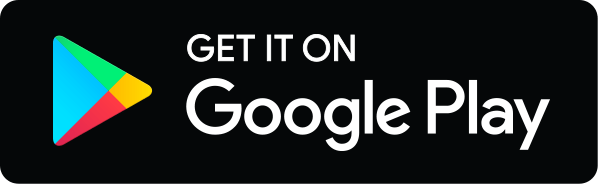