Peripheral artery disease (PAD) has been treated percutaneously since 1964, when Charles Dotter and Melvin Judkins first described the technique of progressive serial dilatation of the arteries of the lower extremity in nine patients (1). Since that time, the techniques used to mechanically improve perfusion to the lower extremities of patients with PAD have been refined significantly, with progression from the use of dilatation catheters to balloon catheters, balloon-expandable (BE) and self-expanding (SE) stents, stent grafts and novel devices like cryotherapy balloons, medicated balloons, biodegradable stents, as well as plaque removal (atherectomy) or ablation devices.
This chapter reviews the current balloons and stents used to perform peripheral interventions and the developing balloon and stent technologies that will continue to advance the percutaneous treatment of PAD.
BALLOONS
Balloon catheters used in interventions in the periphery have similar characteristics to those designed for use in the coronary bed, except that they are available in larger diameters and lengths, and are generally less compliant. There are many balloon catheters available for use and it is important to understand the characteristics of their construction and delivery requirements (Table 7.1).
Balloon catheters used in the periphery are almost exclusively made out of plastic polymers with varying degrees of compliance (Figs. 7.1 and 7.2). The more compliant a balloon is, the less dilatation force there is at the lesion site, and the more it will stretch and expand in an area of low resistance (i.e., adjacent normal vessel). Thus, when used to treat resistant lesions that are common in the periphery, highly compliant balloons have a tendency to fail to adequately fracture the target lesion and overexpand the vessel beyond the lesion site, increasing the risk of dissection in those areas. For this reason, noncompliant balloons are usually the preferred balloon type used in the periphery because they provide a greater dilating force at the target lesion while maintaining a defined diameter in the adjacent normal vessel.
The noncompliant balloons of the periphery are usually made of polyethylene terephthalate (PET) or nylon reinforced polyurethane. This design improves the radial strength of the balloon and has a higher rate of burst pressure allowance. Some manufacturers use additional proprietary additives that serve to strengthen the balloon material and improve puncture resistance. These designs allow for a greater inflation pressure and therefore radial force with maintenance of the defined diameter.
Most peripheral balloons are deliverable through 4- to 6-Fr. sheaths (except for large caliber aortic balloons). The proximal and distal margins of the balloons are usually demarcated by markers and the balloon tips are designed to minimize the crossing profile. Most peripheral balloons are designed to be delivered over the wire. Rapid exchange balloons are most commonly utilized in the treatment of cerebrovascular, renal, or mesenteric artery disease, but some lower extremity rapid exchange balloons have recently been released (e.g., SLEEK Long, Cordis). The diameter of wire with which the balloon is compatible will depend on the vessel diameter being treated (i.e., 0.014″ and 0.018″ for smaller caliber vessels and 0.035″ for larger caliber vessels) (Table 7.1). Peripheral angioplasty balloons are usually inflated using a mixture of 70% saline and 30% contrast for ease of inflation and deflation given their longer lengths and larger diameters. These balloons also tend to have lower nominal and rated burst inflation pressures when compared to coronary balloons.
Focal Pressure Balloons—Scoring/Cutting Balloons
Routine balloon angioplasty achieves luminal gain by fracturing the plaque and creating an irregular dissection in the vessel wall. This can be particularly problematic in patients with fibrocalcific plaque where the barotrauma can be unevenly distributed. It has been argued that focal delivery of pressure can score the target lesion, creating a more controlled dissection that results in a larger luminal gain and a reduction in the incidence of flow-limiting dissections, which ultimately provides a more predictable angiographic result. In addition, these focal pressure balloons tend to achieve greater stability at the treatment site, resulting in less device slippage, and thus reduce injury in adjacent normal vessel. Much of the basic engineering data supporting the use of these devices have not been published in the peer reviewed literature and it is unclear if the plaque scoring benefits of these devices apply in calcific peripheral vessels where they are often used.
The AngioSculpt (AngioScore, Fremont, CA) and VascuTrak 2 (Bard, Tempe, AZ) balloon catheters represent the newest additions to the focal pressure balloon family. The AngioSculpt balloon catheter incorporates a laser-cut highly flexible nitinol scoring element containing three spiral struts that encircle a semicompliant balloon catheter that creates a focal concentration of dilating force along the edges of the scoring element (Fig. 7.3). The VascuTrak 2 balloon has two guidewires placed external to the semicompliant balloon to provide focal longitudinal force to score the plaque. It has been estimated that the focal stress concentration factor provided by this balloon may be 50 to 400 times that produced by a conventional balloon.
Cutting balloons (Boston Scientific, Natick, MA) have been used for nearly two decades. Initially approved for treatment of instent restenosis in the coronary circulation, they have increasingly found application in peripheral intervention (2). Depending on the diameter of the cutting balloon, there are three (i.e., for 1.5- to 4-mm diameter balloons) or four (i.e., for 5- to 8-mm diameter balloons) atherotomes (microsurgical blades) that are fixed longitudinally on the surface of a noncompliant balloon (Fig. 7.4). The microtomes are covered by the balloon in the wrapped defaulted state and are exposed to the vessel wall on inflation, extending 0.127 mm above the height of the balloon. Based on the assumption that the microtomes induce a longitudinal incision with less wall tension than the diffuse circumferential stress provided by conventional percutaneous transluminal angioplasty (PTA) balloons, trauma may be reduced. The current generation of cutting balloons has flexion points at 5-mm intervals along the atherotome, permitting lateral flexibility and better trackability. While the cutting balloon is routinely used in the peripheral vasculature, it is currently FDA approved for the treatment of obstructive lesions of synthetic or native arteriovenous dialysis fistulae.
Figure 7.1 • An example of a noncompliant peripheral angioplasty balloon with polyethylene terephthalate construct with proximal and distal radiopaque markers and tapered ends.
Cryotherapy Balloons
There is currently one commercially available balloon device that uses compressed nitrous oxide at −10°C to deliver cryotherapy to the peripheral arteries (Fig. 7.5). This device delivers both the mechanical treatment of balloon angioplasty and the purported biologic therapy of freezing to effect treatment of the vessel. Freezing of the tissue is believed to alter the plaque’s inflammatory response to angioplasty, reduce elastic recoil, and reduce smooth muscle cell proliferation by inducing apoptosis. The PolarCath Peripheral Dilatation System (Boston Scientific) is indicated to dilate stenoses in the peripheral vasculature, including the iliac, femoropopliteal, infrapopliteal, and renal arteries. This device is also indicated for use in the treatment of restenosed areas within expanded polytetrafluoroethylene (ePTFE) graft.
Figure 7.2 • The Dorado® balloon (Bard) has a reinforcing proprietary composite material that improves puncture resistance and permits use of higher inflation pressures.
Figure 7.3 • AngioSculpt balloon—one of the three nitinol wires on surface of balloon is labeled.
The PolarCath is available in 0.014″ and 0.035″ compatible systems. The 0.014″ system is available in diameters of 2 to 4 mm (in 0.5-mm increments) and 5 and 6 mm, and lengths of 20, 40, 60, and 100 mm. These are deliverable through 5- to 6-Fr. sheaths. The 0.035″ system is available in diameters of 4 to 8 mm (in 1-mm increments) and lengths of 20, 40, 60, 80, and 100 mm. These are deliverable through 6- to 8-Fr. sheaths.
A number of nonrandomized small studies using the PolarCath device have suggested a lower incidence of clinically significant dissections and a reduced need for stenting when this device is used in the femoropopliteal artery. In one study, the 12-month freedom from restenosis was >80% (3). In a separate population with femoropopliteal disease, primary patency rates by ultrasound were ~70% at 9 months (4) and clinical patency at 3 years was 75% (5). Cryotherapy, in its initial evaluation, appears to provide similar results to PTA alone but did not perform well in heavily calcified lesions, areas of instent restenosis, or in vein graft lesions (6). Given its additional cost ($1700 per case on average), randomized data are needed to assess if indeed this strategy has any benefit beyond that associated with conventional PTA.
STENTS
Balloon-Expandable and Self-Expandable Stents—Materials and Design
Stents are broadly classified as either BE or SE based on how deployment is effected (Tables 7.2 and 7.3) (7). In brief, BE stents are mounted in a crimpled state on a balloon that is inflated to deploy the stent against the vessel wall. In contrast, SE stents are manufactured in an expanded state and are then crimped and constrained by a covering sheath that is retracted at the target site to allow the SE stent to expand to a predefined diameter and appose the vessel wall.
The major determinant of whether a stent will behave as a SE versus a BE stent is the material composition of the stent. In general, BE stents are made from steel that is composed of chromium and nickel (L316) (8). This is a corrosion resistant material with low carbon content. Newer BE stents made of cobalt–chromium have been developed for use in the coronary and peripheral circulation. Based on the properties of cobalt–chromium, these stents have the ability to be stronger than stainless steel stents with thinner metal struts, and thus potentially can provide increased radial strength, lower crossing profiles, with enhanced flexibility and deliverability.
Figure 7.4 • Cutting balloon. A: Cutting balloon deflated. B: Cutting balloon inflated. Atherotomes indicated by thin black arrows. Inset—demonstrates protection of atherotomes by pockets on the balloon surface when the balloon is deflated. (With kind permission from Springer Science+Business Media: Cejna M. Cutting balloon: review on principles and background of use in peripheral arteries. Cardiovasc Intewent Radiol. 2005;28:400–408).
SE stents are typically made of nitinol, which is an alloy that consists of an ultrapure mixture of titanium (~55%) and nickel (45%). The unique properties of nitinol (i.e., shape, memory, and superelasticity) allow for SE stents to be constrained in a coaxial design by an external shaft at temperatures 4°C for delivery with very low crossing profiles and for the stent to expand upon warming (>4°C) to its prespecified diameter and shape, providing lasting contact between the stent and the vessel wall.
Figure 7.5 • PolarCath peripheral dilatation system.
Regardless of stent type, the majority of all stents has either a coil or slotted-tube design. A coil stent is derived from a single piece of wire. The coil design is associated with low radial strength but excellent flexibility (which enhances deliverability). This stent design has lost favor due to poor clinical performance both in the coronary and peripheral circulations (9), and such stents are currently mainly used in nonvascular applications. Slotted-tube stents are made from metal cylinders and laser is used to achieve the stent geometry desired. Initial slotted tube designs had excellent radial strength but poor flexibility (and deliverability). Over the last 15 years, significant modifications to the geometry of slotted-tube stents have occurred in an attempt to maintain the same degree of radial strength but enhance flexibility and deliverability. In the coronary circulation, the major emphasis has been on achieving these modifications in BE stents where flexibility and deliverability through tortuous and diseased small caliber vessels have been the priority. In the periphery, there has been significant interest in the modification of SE stents to improve the performance of stents in locations such as the femoropopliteal and internal carotid artery that are subject to conformational change.
The dominant stent geometry that has emerged is that of a sequential ring design (Fig. 7.6). This describes stents that are composed of a series of Z-shaped structural elements (referred to as struts), which are joined by connecting elements (variable referred to as bridges, hinges, or nodes). Sequential ring design stents may be broadly divided into two broad categories—closed cell and open cell. In closed cell stents, all of the inflection points of the structural elements are connected by bridging elements. The original coronary stents had a closed cell design (i.e., Palmaz), which provided optimal mechanical scaffolding but resulted in poor flexibility.
Most current generation coronary and peripheral BE and SE stents have an open cell design, where some or all of the internal inflection points of the structural elements are not connected by bridging elements (10). The periodic connection between adjacent elements may occur in one of four patterns—peak-to-peak (e.g., Sentinol, Boston Scientific, or Luminexx, Bard [Fig. 7.7]), peak-to-valley (e.g., Absolute, Abbott Vascular [Fig. 7.8], or Zilver, Cook [Fig. 6B]), mid-strut to mid-strut, or a hybrid combination of these. These connections may be direct (Absolute, Abbott Vascular) or via off-set bridges (SMART, Cordis). These connectivity features are thought to contribute to what creates the radial and axial strength, the flexibility, and the likelihood of fracture of each individual stent. For example, peak-to-peak connections provide superior radial strength, whereas peak-to-valley connections provide superior scaffolding characteristics.
Other features of the SE stent design that impact performance includes the presence of flaring, and the presence and type of radiopaque markers at the stent ends. Flaring at the stent end is felt to improve the accuracy of stent placement and to reduce the risk of stent migration. Some manufacturers have looped the free strut tips to reduce tissue injury whereas others have placed radiopaque markers at the tips for better radiographic identification. One study acknowledged the improved accuracy of radiographic identification of stents with these tantalum radiopaque markers, but it raised some concern over the potential for corrosion at the junction between the body of the stent and the markers (11). Markers that are clipped on (e.g., SMART, Cordis) proved to have a more homogenous response to this testing. Regardless, this junction of the corpus of the stent to the markers raised the concern for the potential, whether by stent fracture or a mechanism associated with corrosion, for a continued stimulus for inflammation and neointimal hyperplasia and therefore restenosis. This would require dedicated research to further delineate the validity of this concern.
Balloon-Expandable and Self-Expanding Stents—Clinical Application
BE stents have been used in peripheral vascular interventions since 1987, when the Palmaz–Schatz stent was first tested in human iliac arteries (12). Because of their rigidity, BE stents are typically employed in peripheral intervention for the treatment of disease in relatively static arteries, such as the iliac vessels, where torsion and flexion forces are low and there is minimal risk of stent deformation or fracture (Table 7.2) (13). In contrast, these stents perform poorly at sites subject to significant deformation (e.g., superficial femoral artery [SFA], internal carotid artery), and their use at these locations has been supplanted by nitinol SE stents. There is some role for the use of BE stents below the knee, especially with improvements in the drug-eluting stent era, but their widespread use in this location is still investigational. The superior radial strength provided by the BE stainless steel design, relative to the nitinol SE stent design, allows for excellent luminal expansion, especially in larger diameter calcified vessels. Another advantage of BE stents is the ability to achieve precise placement of the stent. This is particularly important in the treatment of ostial disease (e.g., ostium or renal, visceral, and subclavian arteries, and aortoiliac bifurcation). With current antiplatelet regimens including aspirin and clopidogrel, the incidence of stent thrombosis with these stents in the periphery is very low.
SE stents are used in peripheral intervention at sites that are subject to significant deformation (e.g., external iliac artery, SFA, popliteal artery, and internal carotid artery) (Table 7) (13). There is a significant variation in the radial strength and flexibility of various SE stents, which may influence stent choice at certain locations and in certain disease anatomies. For example, a stent with high radial strength (e.g., SMART, Cordis) would be favored for the treatment of a heavily calcified iliac lesion, whereas a highly flexible stent with less radial strength would be favored in treating a noncalcified lesion in the highly mobile distal SFA and popliteal artery (e.g., Life-Stent, Bard [Fig. 7.9]).
Figure 7.6 • Examples of three stents based on a symmetrical sequential ring design. A: SMART Control stent (Cordis) with peak-to-peak off angled connections and radiopaque markers at its flared ends. B: Zilver stent (Cook) with symmetric in-line stent rings connected in a peak-to-valley pattern with radiopaque markers at its unflared ends. C: Everflexstent (ev3) with peak-to-peak direct connections in a spiral connectivity pattern and radiopaque markers at its unflared ends.
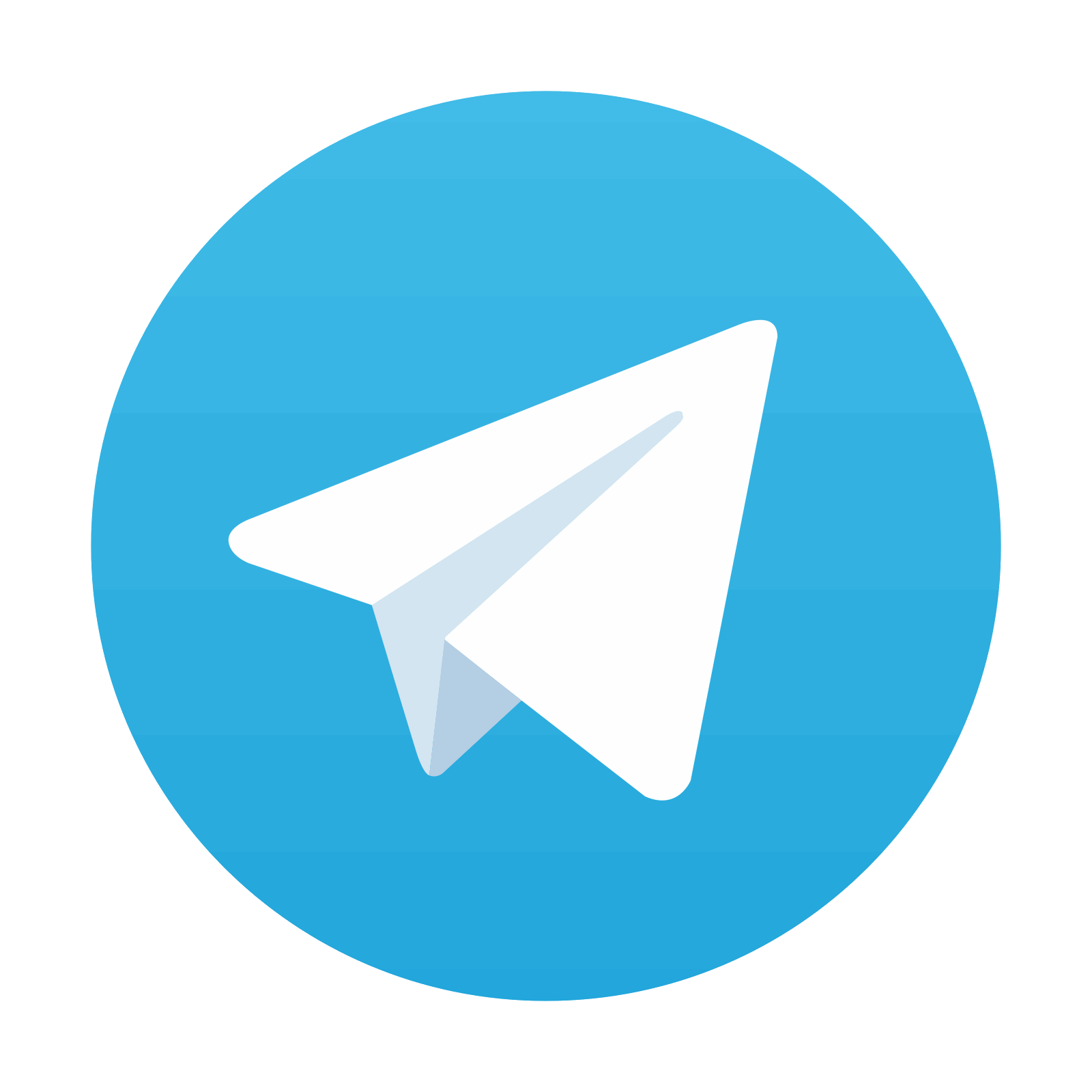
Stay updated, free articles. Join our Telegram channel

Full access? Get Clinical Tree
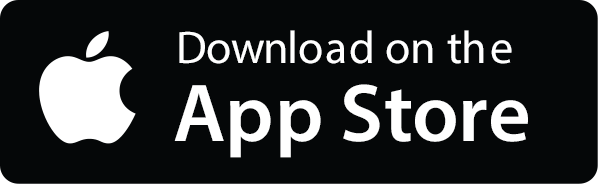
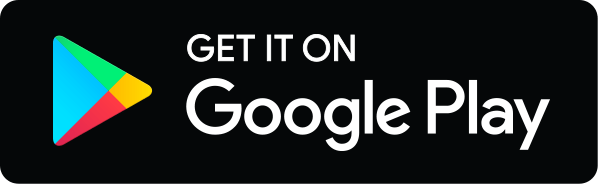