Over the past several decades, advances in medical imaging have drastically improved the ability to noninvasively evaluate the vascular tree. In particular, computed tomography angiography (CTA) and magnetic resonance angiography (MRA) have emerged as tests of choice for the anatomic assessment of a variety of vascular disorders.
Despite these advances, however, clinicians must be aware of the limitations of anatomic tests and the application of this information to their patients. When approaching vascular disease, it is critical to remember that the presence of an anatomic lesion does not always explain the patient’s symptoms. Treatment of anatomic abnormalities without physiologic information and sound clinical judgment may lead to an incorrect diagnosivs and inappropriate treatment of asymptomatic lesions.
Noninvasive tests to evaluate vascular disorders can be broadly divided into physiologic and anatomic tests. The noninvasive vascular lab provides a number of diagnostic tools such as the ankle brachial index, segmental limb pressures, pulse volume recordings, exercise stress testing and Doppler ultrasound (Chapter 2 and 3). These are primarily physiologic tests, although they do provide limited segmental anatomic data. Their primary role is to establish the presence of physiologically significant vascular disease, although they are also quite useful in the follow-up evaluation post-revascularization. After establishing a physiologic problem that explains the patient’s symptoms, an anatomic test such as CTA or MRA can localize and characterize the disease providing an excellent road map for therapeutic intervention.
Although peripheral artery disease (PAD) is the main reason for ordering a CTA or MRA, almost 20% of arterial occlusive disease is secondary to causes other than atherosclerosis. Unlike digital subtraction angiography (DSA), volumetric tests such as CTA and MRA are able to visualize much more than the vascular lumen alone. They provide additional information about the vascular wall including the presence of soft or calcified plaque and the extent of positive remodeling. Both provide visualization of the tissues and organs surrounding the vascular segment of interest and document any pathology affecting these tissues and organs that may be indirectly causing the vascular symptoms and signs. Additionally, both modalities have been used with some success to image venous anatomy and pathology. With increasing acceptance in clinical practice, CTA and MRA are now utilized to evaluate a variety of vascular disorders (Table 4.1).
Both CTA and MRA have distinct advantages and disadvantages. The selection of which test to perform is largely based on the clinical question, patient factors, hospital factors,and physician preferences. The goal of this chapter is to provide the reader with a general overview of each test and its associated strengths and weaknesses.
COMPUTED TOMOGRAPHY ANGIOGRAPHY
CTA utilizes iodinated contrast media and ionizing radiation to obtain high-quality images of the vascular tree. Improvements in multidetector technology have revolutionized CTA in the past several decades. With 16- and 64-channel scanners, large segments of the body can be imaged with a single contrast bolus, often during a single breath-hold. Multidetector CT angiography (MD-CTA) has been shown to have diagnostic accuracy equivalent to DSA for PAD and is an excellent tool in pretreatment planning (1–4).
A typical CTA involves a digital tomogram, an optional noncontrast acquisition, and a contrasted arterial phase acquisition, which may be empirically timed or based on bolus tracking algorithms. Bolus tracking is particularly important in patients with cardiac disease where contrast may arrive to the lower extremities slower than in the average patient. Typical injection rates are 3 to 5 cc/sec for a total volume of 70 to 120 cc of iodinated contrast. Axial images are usually reconstructed in 1- to 2-mm increments. Post-processing algorithms are used to reconstruct images in multiple planes and 3D volumes.
One of many advantages of CTA is widespread availability of multidetector CT scanners, which are often staffed and available at all times for emergent imaging (Fig. 4.1). Protocols are simple, easily reproduced, and imaging quality is often comparable across institutions. Since CT acquisition is rapid, it is substantially less prone to motion artifact than MRA. The CT gantry is relatively large compared with an MR magnet, and CTA is better able to image those with claustrophobia or severe obesity. CTA also has higher spatial resolution and lower overall cost compared with MRA. Finally, CTA has particular utility in the evaluation of stents, stent grafts, and endoleaks (Fig. 4.2).
Figure 4.1 • 71-year-old patient following percutaneous intervention with pain at the access site after use of closure device. Maximum intensity projection (MIP) of a MD-CTA showing acute occlusion of the left common femoral artery (white arrows). Profunda femoral artery indicated by interrupted white arrow. Superficial femoral artery indicated by black arrow.
One of the major disadvantages of CTA is the use of iodinated contrast, which can lead to renal dysfunction,particularly in those with preexisting chronic renal insufficiency (CRI). CT imaging utilizes ionizing radiation, which contributes to an increasing dose of medical radiation to the population. CTA is prone to beam hardening or streak artifacts from calcium and bone that can limit the ability to visualize the vascular lumen. This is particularly evident in infrapopliteal calcified vascular disease where it may be impossible to determine vascular patency in heavily calcified tibial vessels. While widely quoted in the literature as a disadvantage, the ability of CTA to detect calcium can also be quite advantageous. The amount and distribution of calcium are critically important for both surgical and endovascular procedures and can greatly affect the approach to therapeutic intervention. A circumferentially calcified vessel that is widely patent may not be an appropriate target for surgical bypass, and heavy calcification may greatly affect the success of endovascular procedures such as re-entry during subintimal recanalization, balloon angioplasty, atherectomy, or stenting (Fig. 4.3). The advantages and disadvantages of CTA are presented in Table 4.2.
Figure 4.2 • A: Sagittal reformat of CTA demonstrating distal migration of aortic stent graft with type Ia proximal endoleak. Solid white arrow indicates the position of the proximal margin of the stent graft. B: Axial CTA of aortic stent graft patient with type II endoleak in the posterior aneurysm sac from a lumbar artery (arrow).
MAGNETIC RESONANCE ANGIOGRAPHY
MR imaging utilizes powerful magnetic fields to measure the relaxation of protons when subject to various excitation fields. MRA has seen many improvements in the past several decades relating to high field magnets with larger fields of view, stronger and faster gradients, multichannel surface coils covering body segments of interest, parallel imaging, and moving table technology. Perhaps the greatest improvement in MRA quality and speed, however, has been with the use of contrast-enhanced MRA (CE-MRA) (Fig. 4.4), which utilizes gadolinium-chelated contrast agents. CE-MRA has also been shown to have a diagnostic accuracy equivalent to conventional DSA in the evaluation of PAD and provides an excellent layout for pretreatment planning (5) (Fig. 4.5). MRA protocols are significantly more complex than CTA protocols and vary across institutions and vendors.
Figure 4.3 • A: Axial CTA in a patient with severe right greater than left claudication demonstrates severely calcified stenosis of the infra-renal abdominal aorta that extends into the right common iliac artery. B: Correlate angiogram demonstrating severely calcified aortoiliac disease (worst sites of calcification indicated by arrows). C: Angiogram after placement of covered balloon-expandable aortic and kissing iliac stents. D: Axial CTA after kissing stent placement.
CE-MRA is generally superior to nonenhanced MRA as it is less prone to in-plane saturation and turbulence artifacts and requires substantially less time to image long vascular segments. CE-MRA of the lower extremities is performed using a multistation, moving-table, boluschase technique that is widely used and available on most magnets. Initial protocols optimized the bolus to the first abdominal aortic station. Subsequent thigh and calf stations were imaged consecutively as fast as possible, making venous contamination in the calf, and to a lesser degree in the thigh, a problem in interpretation of the arterial tree (6). Later protocols addressed venous contamination problems by using separate bolus timing injections combined with time-resolved images of the calf vessels performed prior to acquiring the CE-MRA of the more proximal arteries (7). These techniques, however, used large volumes of gadolinium contrast and increased the risks of acquiring nephrogenic systemic fibrosis (NSF) in patients with moderate to severe CRI and patients with ESRD on dialysis. NSF is further discussed later in the text in the section regarding complications.
More current protocols for CE-MRA of the lower extremities utilize a blood pressure cuff compression technique (Fig. 4.6). Blood pressure cuffs are applied to each thigh and inflated to a pressure that is sufficient to achieve venous occlusion but insufficient to cause arterial occlusion. This results in delayed venous filling allowing more time to image the thigh and calf stations before venous contamination occurs (8) (Fig. 4.7). In spite of these techniques, however, venous contamination can still be a problem in patients with leg hyperemia secondary to cellulitis, ulcers, or other injuries (Fig. 4.8).
Figure 4.4 • Maximum intensity projection (MIP) reconstruction of a contrast enhanced (CE)-MRA runoff of a 46-year-old patient with atypical symptoms in the right lower extremity showing normal abdominal aorta and bilateral iliac and lower extremity arteries.
A variety of noncontrast MRA techniques are available to image the vascular tree, although these are more time-consuming and subject to greater artifacts than CE-MRA. These include 2D time-of-flight (TOF) imaging (Fig. 4.9), 3D TOF, 2D phase contrast (PC), 3D PC, and balanced steady state free precession (Fig. 4.10). The most recent of the nonenhanced MRA techniques is the 3D flow-spoiled, electrocardiogram-triggered, half-Fourier fast spin echo imaging. This technique subtracts the vascular signal during diastole and systole. Imaged arteries are bright during diastole and dark during systole; so subtractions of these two images display arteries as bright. Veins are bright during both diastole and systole, which eliminates venous signal from subtracted images (Fig. 4.11).
Figure 4.5 • A: MIP reconstruction of a CE-MRA from a 56-year-old male smoker showing bilateral chronic superficial femoral artery (SFA) occlusions. B: MIP reconstruction of a CE-MRA from a 59-year-old patient with bilateral lower extremity claudication and chronic nonhealing right foot ulcer showing bilateral diffuse mild SFA disease and diffuse three vessel tibial disease with hyperemia (arrow) secondary to a nonhealing right foot ulcer. C: MIP of a CE-MRA runoff from a 56-year-old patient with bilateral lower extremity claudication showing bilateral diffuse moderate SFA disease and diffuse three vessel tibial disease. D: MIP of a CE-MRA of the upper extremities from a 51-year-old patient showing occlusion of the innominate artery (arrow). E: MIP of a CE-MRA of the upper extremities from a 43-year-old patient showing moderate narrowing of the right subclavian artery (arrow).
Figure 4.6 • A: Third station MIP image from a CE-MRA runoff without the use of thigh cuff showing venous contamination (arrows) involving the right calf that causes difficulty in interpretation of tibial arterial anatomy in this patient with a chronic nonhealing right foot ulcer. B: CE-MRA MIP of the right calf showing moderately diseased anterior tibial and mildly diseased peroneal arteries without venous contamination. C: CE-MRA MIP of the left calf showing normal tibial vessels. D: Third station MIP image from a runoff without the use of thigh cuff showing venous contamination involving both calves. E: CE-MRA MIP of the right calf showing occlusion of the tibioperoneal trunk (solid arrow) and severe disease of anterior tibial artery (interrupted arrow) without venous contamination. F: CE-MRA MIP of the left calf showing occlusion of the anterior tibial (solid arrow) and posterior tibial (interrupted arrow) arteries, and patency of the peroneal artery without venous contamination.
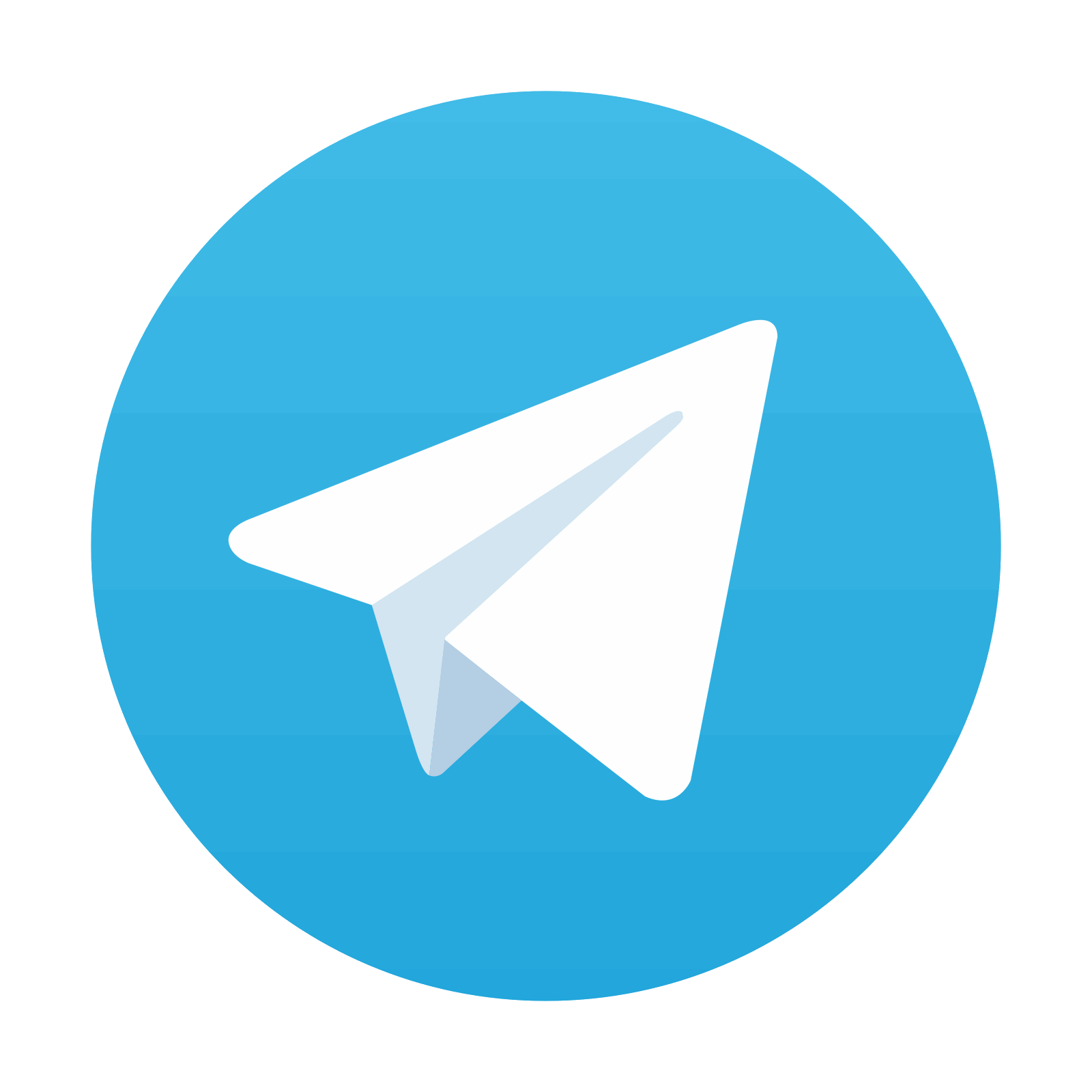
Stay updated, free articles. Join our Telegram channel

Full access? Get Clinical Tree
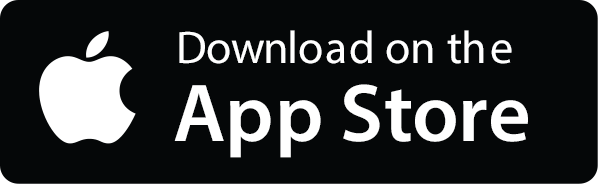
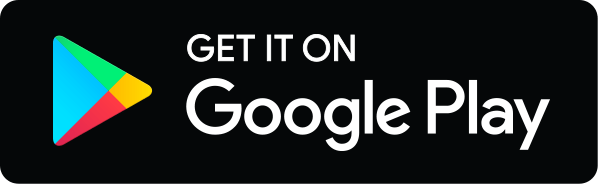