Introduction
This chapter discusses how the distal lung epithelium regulates lung fluid balance by active ion transport mechanisms across both the alveolar and the distal airway epithelium. Both experimental models of pulmonary edema and clinical studies are considered to illustrate how active sodium and chloride ion transport regulate the resolution of alveolar edema. Some of the material in this chapter has been included in recent reviews.
For many years it was generally believed that differences in hydrostatic and protein osmotic pressures (Starling forces) accounted for the removal of excess fluid from the air spaces of the lung. This misconception persisted in part because some experiments that measured solute flux across the epithelial and endothelial barriers of the lung were done at room temperature and the studies were done in dogs, a species that turned out to have a very low rate of active sodium and fluid transport. Although the removal of interstitial pulmonary edema by lung lymphatics and the lung microcirculation was known for a long time, there was no information on how pulmonary edema was removed from the distal air spaces of the mature lung. Insights into the contribution of active ion transport came from experimental studies of the clearance of alveolar fluid in the mature lung and at the time of birth as well as the development of culture systems to study pulmonary epithelial cells.
Lung Epithelial Fluid Absorption
With few exceptions, the general model for transepithelial fluid movement is that active salt transport drives osmotic water transport. This paradigm is also correct for fluid clearance from the distal air spaces of the lung. The results of several in vivo studies demonstrated that changes in hydrostatic or protein osmotic pressures cannot account for the removal of excess fluid from the distal air spaces. Furthermore, pharmacologic inhibitors of sodium transport can reduce the rate of fluid clearance in the lungs of several different species, including the human lung. In addition, there is good evidence that isolated epithelial cells from the distal air spaces of the lung actively transport sodium and other ions, resulting in osmotic water absorption of fluid from the distal air spaces of the lung.
The large surface area of the alveoli favors the hypothesis that most fluid is reabsorbed at the alveolar level, although fluid may be actively reabsorbed across all of the different segments of the pulmonary epithelium of the distal lung. The precise contribution of each anatomic segment in the distal air spaces to fluid reabsorption is not firmly established. The distal airway epithelium is composed of terminal respiratory and bronchiolar units containing polarized epithelial cells that have the capacity to transport sodium and chloride, including ciliated cells and nonciliated cuboidal club cells (Clara). The alveoli themselves are composed of a thin alveolar epithelium (0.1 to 0.2 µm) that covers 99% of the air space surface area in the lung and contains thin, squamous type I cells and cuboidal type II cells. The alveolar type I cell covers 95% of the alveolar surface. The close apposition between the alveolar epithelium and the vascular endothelium facilitates efficient exchange of gases but also forms a tight barrier to movement of liquid and proteins from the interstitial and vascular spaces, thus assisting in maintaining relatively dry alveoli.
Ion transporters and other membrane proteins are asymmetrically distributed on opposing cell surfaces, conferring vectorial transport properties to the epithelium. Physiologic studies of the barrier properties of tight junctions in the alveolar epithelium indicate that diffusion of water-soluble solutes between alveolar epithelial cells is much slower than through the intercellular junctions of the adjacent lung capillaries. Large quantities of soluble protein are removed from the air spaces primarily by restricted diffusion, although there is evidence for some endocytosis and transcytosis of albumin across the alveolar epithelium.
Evidence for Active Fluid Transport in the Intact Lung
A substantial number of innovative experimental methods have been used to study fluid and protein transport from the distal air spaces of the intact lung, including isolated perfused lung preparations, in situ lung preparations, surface fluorescence methods, and intact lung preparations in living animals for short time periods (30 to 240 minutes) or for extended time periods (24 to 144 hours). The advantages and disadvantages of these preparations have been reviewed in some detail.
The first in vivo evidence that active ion transport could account for the removal of alveolar edema fluid across the distal pulmonary epithelium of the mature lung was obtained in studies of anesthetized, ventilated sheep. In those studies the critical discovery was that isosmolar fluid clearance of salt and water occurred in the face of a rising concentration of protein in the air spaces of the lung, whether the instilled solution was autologous serum or an isosmolar protein solution. The initial protein concentration of the instilled protein solution was the same as that of the circulating plasma. After 4 hours the concentration of the protein had risen from approximately 6.5 g/100 mL to 8.4 g/100 mL, whereas the plasma protein concentration was unchanged. In longer-term studies in unanesthetized, spontaneously breathing sheep, alveolar protein concentrations increased to very high levels. After 12 and 24 hours, the alveolar protein concentrations increased to 10.2 and 12.9 g/100 mL, respectively. The overall rise in protein concentration was equivalent to an increase in distal air space protein osmotic pressure from 25 to 65 cm H 2 O. Because the epithelial barrier was intact and thus prevented protein leak into the lung, the only way that the protein concentration could have risen was if water had been removed (i.e., absorbed).
Other studies in the intact lung have supported the hypothesis that removal of alveolar fluid requires active transport. For example, elimination of ventilation to one lung did not change the rate of fluid clearance in sheep, thus ruling out changes in transpulmonary airway pressure as a major determinant of fluid clearance, at least in the uninjured lung. Furthermore, if active ion transport were responsible for alveolar fluid removal, then fluid clearance should be temperature dependent. In an in situ rat lung preparation, fluid clearance was inhibited by low temperature. Similar results were obtained in ex vivo human lung studies, in which hypothermia inhibited sodium and fluid transport.
Additional evidence for active ion transport was obtained in intact animals with the use of amiloride, an inhibitor of sodium uptake by the apical membrane of alveolar epithelium and distal airway epithelium. Amiloride inhibited 40% to 70% of basal fluid clearance in sheep, rabbits, rats, guinea pigs, and mice and in the human lung. Amiloride also inhibited sodium uptake in distal airway epithelium from sheep and pigs. To explore further the role of active sodium transport, experiments were designed to inhibit Na + ,K + -ATPase. It has been difficult to study the effect of ouabain in intact animals because of cardiac toxicity. However, in the isolated rat lung, ouabain inhibited greater than 90% of fluid clearance. Following the development of an in situ sheep preparation for measuring fluid clearance in the absence of blood flow, it was reported that ouabain inhibited 90% of fluid clearance over a 4-hour period. Other investigators also established the likely role of active fluid transport for removal of fluid from the fetal lung.
Ion Transport in Alveolar and Distal Airway Epithelial Cells
The success in obtaining nearly pure cultures of alveolar epithelial type II cells from rats made it possible to study the transport properties of these cells and relate the results to the findings in the intact lung studies. When alveolar epithelial type II cells were cultured on a nonporous surface such as plastic, they formed a continuous confluent layer of polarized cells after 2 to 3 days. Interestingly, after 3 to 5 days, small domes of fluid could be appreciated below which the substratum was detached by the formation of the domes. The domes were thought to result from active ion transport from the apical to the basal surface, with water following passively, because they were inhibited by the replacement of sodium by another anion or by pharmacologic inhibitors of sodium transport, such as amiloride and ouabain. More detailed information on the nature of ion transport across alveolar type II cells was obtained by culturing these cells on porous supports and mounting them in Ussing chambers and measuring short-circuit current and ion flux under voltage clamp conditions.
The coordinated role of apical and basolateral sodium transport has been studied in several in vitro studies. Sodium ions that enter the epithelial cells at the apical membrane are pumped out of the cells at the basolateral membrane by the Na + ,K + -ATPase enzyme. Because of the continuous pumping, sodium chemical potential is lower inside the cell. The entry step is passive, and sodium flows down a chemical potential gradient through specialized pathways, where basolateral transport requires energy to move ions against the gradient. Because of the pump activity, potassium electrochemical potential is larger inside the cell, and potassium leaks through the basolateral membrane and is then recycled by the Na + ,K + -ATPase. The pathways for sodium entry into alveolar type II cells are numerous. Amiloride blocked dome formation and decreased short-circuit current in the in vitro studies, a finding that supported the critical importance of sodium uptake through an amiloride-sensitive pathway in the apical membrane of alveolar epithelial cells. As already discussed, the efficacy of amiloride as an inhibitor of fluid clearance in the intact lung was demonstrated in several in vivo studies, although the fraction of amiloride-sensitive transport was as low as 40% to 50% in some lung preparations, particularly in the rat and the human lung. The amiloride-insensitive sodium influx may be represented in vivo in part by the Na + -glucose cotransport. A detailed discussion of the pharmacologic, biophysical, and molecular bases for fluid clearance across the alveolar and distal airway epithelium is available in other references.
The role of the alveolar type I cell in vectorial fluid transport in the lung was unknown until recently; investigators have now assessed the potential contribution of the alveolar type I cell to vectorial fluid transport. On the basis of studies in freshly isolated type I cells, it is known that these cells have a high osmotic permeability to water with expression of aquaporin-5 on the apical surface. Recent studies of freshly isolated alveolar type I cells from rats demonstrated that these cells express the Na + ,K + -ATPase α 1 – and α 2 -subunit isoforms. In the same study there was evidence for Na + ,K + -ATPase α 1 -subunit expression on the basolateral surface of the alveolar epithelial type I cells in situ in the rat lung. In addition, there is evidence for expression of all the subunits of the epithelial Na + channel (ENaC) in freshly isolated alveolar type I cells, as well as in situ in the rat lung. Finally, there is some evidence that Na uptake can be partially inhibited by amiloride in freshly isolated rat alveolar type I cells, although definitive studies of cultured, polarized type I cells have not yet been achieved. Recent data demonstrate that the alveolar epithelial type I cells express a multitude of sodium transporting proteins, ENaC, cyclic nucleotide–gated sodium channels, and the Na + ,K + -ATPase. In addition, the cells express chloride transporters, that is, the cystic fibrosis transmembrane regulator (CFTR), and thus have the ion transporters necessary to participate in vectorial ion transport and clearance of alveolar fluid. The relative contribution of the type I and type II cells to alveolar edema fluid clearance is still unresolved.
The alveolar epithelium constitutes 99% of the surface area of the lung, a finding that suggests that edema fluid from the lung might be removed primarily across the alveolar epithelium. However, it has been demonstrated that the distal airway epithelium also actively transports sodium, a process that depends on amiloride-inhibitable uptake of sodium on the apical surface and extrusion of sodium through a basolateral Na + ,K + -ATPase. Club cells actively absorb and transport sodium from the apical to the basal surface. In addition, there are new data on the possible role of CFTR in up-regulating cyclic 3′,5′-adenosine monophosphate (cAMP) fluid clearance (see “ Regulation of Lung Epithelial Fluid Transport ” section). This information provides support for a possible role of distal airway epithelia in fluid clearance, because CFTR is expressed abundantly in distal airway epithelial cells, as well as in alveolar epithelial cells. Thus, even though their surface area is limited, a contribution from distal airway epithelia to the overall fluid transport is probable, especially because cells from the distal airway epithelium primarily transport salt from the apical to the basolateral surface. Figure 9-1 provides a schematic diagram of our current understanding of the location of ion transporters in the distal airway and alveolar epithelium that are responsible for vectorial fluid transport or net distal air space fluid clearance.

There are also new data confirming the importance of ENaC for alveolar fluid clearance using RNA interference against the αENaC subunit. In those studies, inhibition of αENaC expression by specific small interfering RNA against αENaC attenuated the amiloride sensitivity of both normal and stimulated alveolar fluid clearance, thus demonstrating the importance of ENaC. Finally, there is a newly cloned δ subunit of ENaC that has been identified in human lungs and may play a role in fluid balance in the lung.
Regulation of Lung Epithelial Fluid Transport
This section considers how the rate of vectorial fluid transport across the distal pulmonary epithelium can be increased by catecholamine or cAMP-dependent mechanisms. The potential relevance of these mechanisms under pathologic conditions is evaluated in the section “Alveolar Fluid Transport under Pathologic Conditions,” later in this chapter.
Studies in newborn animals indicated that endogenous release of catecholamines, particularly epinephrine, may stimulate reabsorption of fetal lung fluid from the air spaces of the lung. In most adult mammalian species, stimulation of β 2 -adrenergic receptors by either salmeterol, terbutaline, or epinephrine increases fluid clearance. This stimulatory effect rapidly follows intravenous administration of epinephrine or instillation of terbutaline into the alveolar space and is prevented by either a nonspecific β 2 -receptor antagonist (propranolol) or, in rats, by a specific β 2 -antagonist.
The increased fluid clearance by β 2 -agonists can be prevented by amiloride or RNA interference against αENaC, indicating that the stimulation is related to an increased transepithelial sodium transport. In anesthetized ventilated sheep, terbutaline-induced stimulation of fluid clearance was also associated with an increase in lung lymph flow, a finding that reflected increased removal of some of the alveolar fluid volume to the interstitium of the lung. Although terbutaline increased pulmonary blood flow, this effect was not important because control studies with nitroprusside, an agent that increased pulmonary blood flow, did not increase fluid clearance. Other studies have demonstrated that β-adrenergic agonists increased fluid clearance in rat, dog, guinea pig, and mouse, as well as human lung. The presence of β 1 – and β 2 -receptors on alveolar type II cells has been demonstrated in vivo by autoradiographic and immunochemistry techniques.
It has been difficult to quantify the effect of catecholamines on the rate of alveolar fluid clearance and edema resolution in humans. However, studies of fluid clearance in the isolated human lung have demonstrated that β-adrenergic agonist therapy increases fluid clearance, and the increased fluid clearance can be inhibited with propranolol or amiloride. Subsequent studies suggested that long-acting lipid-soluble β-agonists may be more potent than hydrophilic β-agonists in the ex vivo human lung. The magnitude of the stimulatory effect is similar to that observed in other species, with a β-agonist–dependent doubling of fluid clearance over baseline levels. These data are particularly important because aerosolized β-agonist treatment in some patients with pulmonary edema might accelerate the resolution of alveolar edema (see “ Alveolar Fluid Transport under Pathologic Conditions ” section).
What has been learned about the basic mechanisms that mediate the catecholamine-dependent up-regulation of sodium transport in the lung? Based on in vitro studies, it was proposed that an increase in intracellular cAMP resulted in increased sodium transport across alveolar type II cells by an independent up-regulation of the apical sodium conductive pathways and the basolateral Na + ,K + -ATPase. Proposed mechanisms for up-regulation of sodium transport proteins by cAMP include augmented sodium channel open probability, increases in Na + ,K + -ATPase α-subunit phosphorylation, and delivery of more ENaC channels to the apical membrane and more Na + ,K + -ATPases to the basolateral cell membrane.
Although most experimental studies have attributed a primary role for active sodium transport in the vectorial transport of salt and water from the apical to the basal surface of the alveolar epithelium of the lung, the potential role of chloride, especially in mediating the cAMP-mediated up-regulation of fluid clearance across distal lung epithelium, has been the subject of several studies. A study of cultured alveolar epithelial type II cells suggested that cAMP-mediated apical uptake of sodium might depend on an initial uptake of chloride. A more recent study of cultured alveolar type II cells under apical surface–air interface conditions reported that β-adrenergic agonists produced acute activation of apical chloride channels with enhanced sodium absorption. However, the results of these studies were considered to be inconclusive by some investigators, partly because the data depend on cultured cells of an uncertain phenotype. Type II cells cultured under conditions for studying transepithelial transport are usually studied under conditions that no longer maintain their phenotype as defined by surfactant protein expression. Furthermore, studies of isolated alveolar epithelial type II cells do not address the possibility that vectorial fluid transport may be mediated by several different epithelial cells, including alveolar epithelial type I cells as well as distal airway epithelial cells. The relative contribution of alveolar type I cells and alveolar type II cells to transepithelial transport remains unresolved.
In order to define the role of chloride transport in the active transport of salt and water across the distal pulmonary epithelium of the lung, one group has used in vivo lung studies to define the mechanisms and pathways that regulate chloride transport during the absorption of fluid from the distal air spaces of the lung. This approach may be important because studies in several species, as already discussed, have indicated that distal airway epithelia are capable of ion transport and that both ENaC and CFTR are expressed in alveolar and distal airway epithelia.
Both inhibition and ion substitution studies demonstrated that chloride transport was necessary for basal fluid clearance. The potential role of CFTR under basal and cAMP-stimulated conditions was tested using intact lung studies in which CFTR was not functional because of failure in trafficking of CFTR to the cell membrane, the most common human mutation in cystic fibrosis (ΔF508 mice). The results supported the hypothesis that CFTR was essential for cAMP-mediated up-regulation of isosmolar fluid clearance from the distal air spaces of the lung because fluid clearance could not be increased in the ΔF508 mice either with β-agonists or with forskolin, unlike in the wild-type control mice. Additional studies using pharmacologic inhibition of CFTR in both mouse and human lungs with glibenclamide supported the same conclusion, namely that chloride uptake and CFTR-like transport seemed to be required for cAMP-stimulated fluid clearance from the distal air spaces of the lung. Glibenclamide can also inhibit potassium channels, so the inhibitory effects may not be specific for CFTR, but the ΔF508 mouse studies have provided more direct evidence. Although the absence of CFTR in the upper airways results in enhanced sodium absorption, the data in these studies provide evidence that the absence of CFTR prevents cAMP–up-regulated fluid clearance from the distal air spaces of the lung, a finding that is similar to the results from studies on the importance of CFTR in mediating cAMP-stimulated sodium absorption in human sweat ducts. Because CFTR is distributed throughout the distal airway epithelium, as well as at the alveolar level in the human lung, the data also suggest that the cAMP-mediated up-regulated reabsorption of pulmonary edema fluid may take place across distal airway epithelium as well as at the level of the alveolar epithelium. Finally, additional studies indicated that the lack of CFTR results in a greater accumulation of pulmonary edema in the presence of a hydrostatic stress, thus demonstrating the potential physiologic importance of CFTR in up-regulating fluid transport from the distal air spaces of the lung. There is evidence that functional CFTR chloride channels are present in adult rat alveolar epithelial type II cells based on whole cell patch-clamp experiments. In addition, there is expression of CFTR in both alveolar type I and II cells. A study using a novel CFTR-specific inhibitor, CFTR Inh-172 , in human lungs again demonstrated the importance of CFTR and chloride transport for cAMP-driven fluid absorption.
In the last few years, several interesting catecholamine-independent mechanisms have been identified that can up-regulate fluid transport across the distal air spaces of the lung as well as in cultured alveolar type II cells. Hormonal factors such as glucocorticoids can up-regulate transport by transcriptional mechanisms, whereas thyroid hormone may work by a posttranslational mechanism. Some growth factors can work by either transcriptional or direct membrane effects or by enhancing the number of alveolar type II cells. For example, keratinocyte growth factor is a potent mitogen for alveolar type II cells. Administration of keratinocyte growth factor (5 mg/kg) into the distal air spaces of the rat lung increases fluid clearance by 66% over baseline levels. Other investigators have shown that keratinocyte growth factor can enhance sodium and fluid transport in normal and injured rat lungs. Keratinocyte growth factor may also work by enhancing the expression of sodium transport proteins. There is also evidence that a proinflammatory cytokine, tumor necrosis factor-α, can rapidly up-regulate sodium uptake and fluid transport. The effect of tumor necrosis factor-α is amiloride inhibitable in both rats and isolated A549 human cells. Finally, serine proteases can regulate the activity of ENaC and potentially increase fluid clearance across the distal airway epithelium. These catecholamine-independent mechanisms are explored in more detail in one review. Recent work has demonstrated that isolated human alveolar epithelial type II cells have the capacity to absorb sodium by ENaC-dependent mechanisms and secrete chloride by CFTR-dependent pathways. Based on these in vitro studies, alveolar epithelial type II cells (1) achieved an extracellular nucleotide concentration–dependent steady state alveolar surface liquid height of approximately 4 µm in vitro, (2) absorbed liquid when the lumen was flooded, and (3) secreted liquid when treated with uridine triphosphate or forskolin or subjected to cyclic compressive stresses mimicking tidal breathing. Collectively these studies suggest that human alveolar epithelial type II cells in vitro have the capacity to absorb or secrete liquid in response to local alveolar conditions.
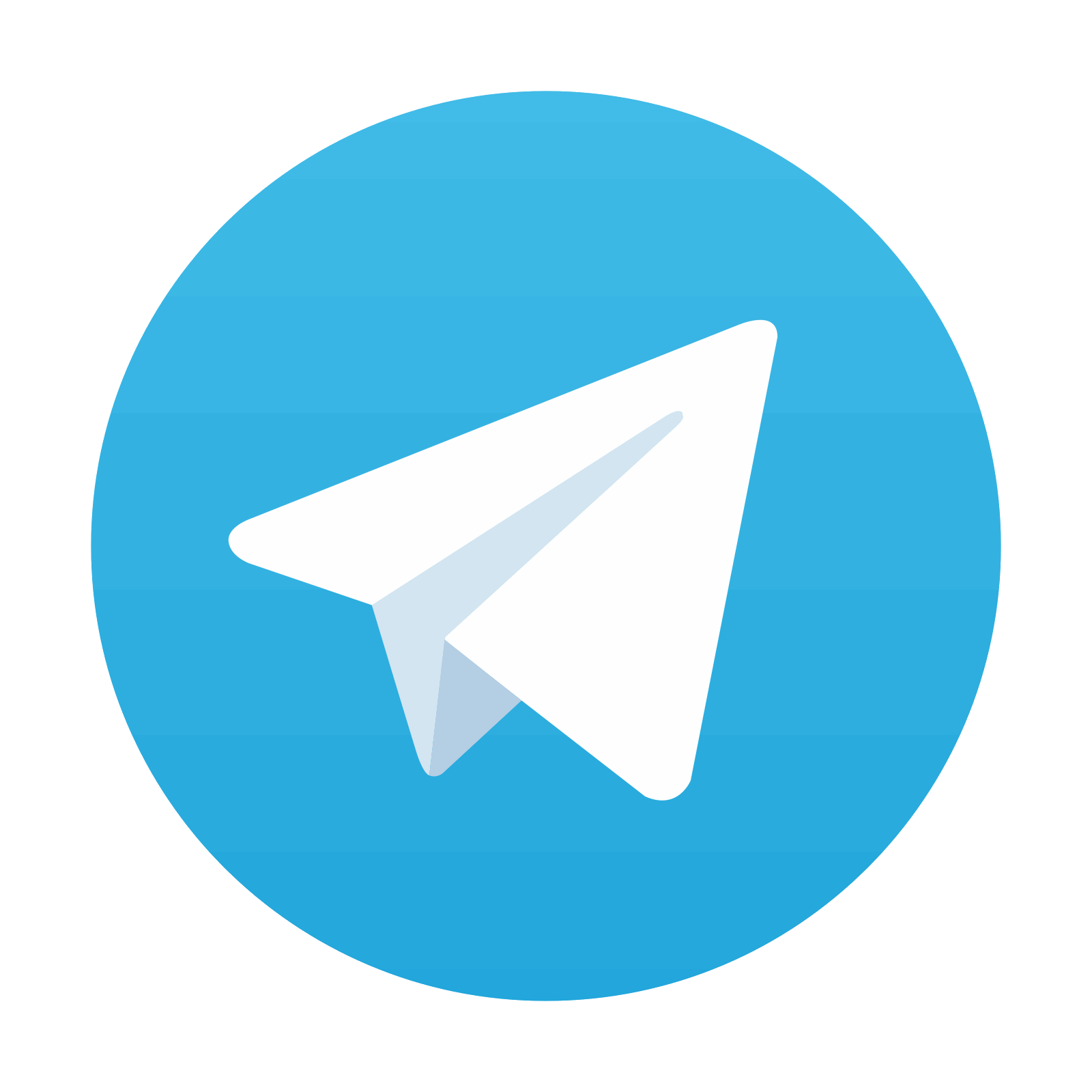
Stay updated, free articles. Join our Telegram channel

Full access? Get Clinical Tree
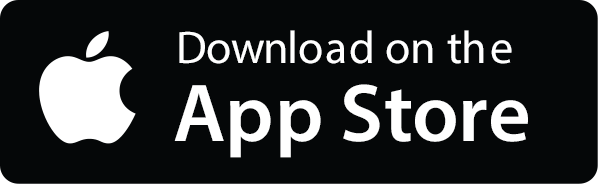
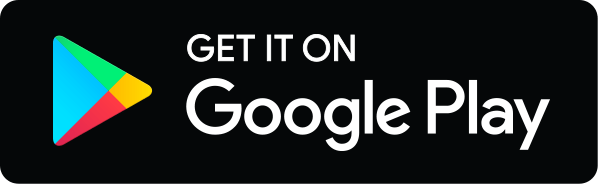