CHAPTER 60 Adult Cardiac Anesthesia
PREOPERATIVE EVALUATION
The preoperative anesthetic evaluation of the cardiac surgical patient includes the following: (1) review of the events that led to the indication for cardiac surgery, (2) identification and optimization of risk factors that could lead to mortality and morbidity resulting from cardiac surgery, and (3) gathering of information relevant to selecting monitoring modalities and management techniques during surgery. Higgins and colleagues1 performed a retrospective logistic regression on data from more than 5000 patients who underwent coronary artery bypass grafts, and they then prospectively applied the retrospectively identified risk factors to a group of more than 4000 patients. They found that 30-day mortality was predicted by the emergency nature of the procedure, preoperative serum creatinine of more than 168 mmol/L, left ventricular ejection fraction of less than 35%, preoperative hematocrit level of less than 35, age greater than 70 years, chronic pulmonary disease, prior vascular surgery, reoperation, and mitral regurgitation. Emergency cases were transfers from the coronary care unit with unstable angina (48%), complications of percutaneous intervention (40%), and complications of routine cardiac catheterizations (12%). In addition to these risk factors, nonfatal morbidity was predicted by diabetes mellitus, body weight of 65 kg or less, aortic stenosis, and cerebrovascular disease. Presence of these risk factors needs to be ascertained when obtaining preoperative history prior to cardiac surgery.
Emergency patients from the catheterization laboratory may have taken inhibitors of platelet aggregation. Clopidogrel (Plavix) and ticlopidine (Ticlid) inhibit ADP-induced platelet aggregation by irreversibly modifying platelet P2 receptors for the life span of these cells. Abciximab (ReoPro) is a monoclonal antibody fragment against glycoprotein (GP) IIb/IIIa receptor, which allows platelet binding to von Willebrand factor and fibrinogen. Abciximab is administered intravenously with an initial bolus of 0.25 mg/kg, followed by an infusion of 0.25 mg/kg/min (up to 10 mg/min) for 12 hours. After discontinuance, platelet function recovers gradually over the next 48 hours.2,3 Eptifibatide (Integrilin) is a peptide inhibitor of GP IIb/IIIa receptor with an elimination half-life of 1 to 2 hours. After discontinuance, platelet function (as measured by bleeding time) recovers in 2 to 4 hours.4 Tirofiban (Aggrastat) is a peptidomimetic antagonist of GP IIb/IIIa receptor with an elimination half-life of about 2 hours; platelet function returns to normal within 4 to 8 hours after discontinuing the medication.5,6 Whereas abciximab consistently prolongs the activated clotting time (ACT) used to monitor heparinization by 30 to 50 seconds and necessitates a reduction in the dosage of heparin used concurrently to decrease bleeding complications, eptifibatide does not have a similar effect on ACT.7
Additional historical information about anticoagulant use that needs to be gathered preoperatively includes duration of use of heparin, heparin resistance, heparin-induced thrombocytopenia, use of Coumadin, dosage and the time of the last dose, and the use of thrombolytics such as streptokinase. Additional historical information pertinent to cardiac surgery includes the presence of esophageal disease that may contraindicate the use of TEE, a history of cold agglutinin antibody (which may contraindicate hypothermic CPB), a history of antiphospholipid syndrome (which will adversely affect measurement of ACT), a history of previous thoracic surgery or radiation, and the presence of a pacemaker or an implantable cardioverter/defibrillator. Finally, a list of medications should be obtained. In general, all cardiovascular medications should be continued even on the day of surgery, except perhaps diuretics. Preoperative β-adrenergic blocker use has been associated with survival after coronary artery surgery.8
Physical examination of the patient should be focused on the airway and the cardiovascular system. The airway examination should include dentition: any evidence of infection or abscess must be discovered. Blood pressure should be measured on both arms, and any significant difference should be noted to rule out possible subclavian artery stenosis. Peripheral pulses should be palpated and graded. Allen’s test may be performed in case a radial artery harvest is being considered to provide a conduit. Femoral pulsation should be palpated, and the presence of any peripheral vascular disease should be noted (this may make the placement of an intra-aortic balloon challenging). Carotid bruits should be listened for, and cardiac sounds should be auscultated. Hepatomegaly, jugular venous distention, and peripheral edema should be noted.
INTRAOPERATIVE MANAGEMENT
Monitoring
The American Society of Anesthesiologists’ standard monitoring protocol includes surface electrocardiography (ECG), pulse oximeter, capnography, (noninvasive) blood pressure monitoring, and temperature monitoring. On surface ECG, ST-segment analysis should be continuously performed. When ischemia is defined as greater than or equal to 0.1 mV (1 mm) horizontal or downsloping ST depression, greater than or equal to 0.15 mV (1.5 mm) upsloping ST depression, or greater than or equal to 0.15 mV (1.5 mm) ST elevation in a non-Q wave lead, the sensitivity of the V5 lead alone is 75%; that of V5 and V4 is 90%; that of V5 and II is 80%; and that of V5, V4, and II is 96%.9 Use of the standard five-lead ECG system does not allow simultaneous monitoring of V5 and V4, and the most practical alternative is to monitor leads V5 and II, the latter of which is also useful for arrhythmia monitoring. When right-sided ischemia is of concern, the right shoulder lead (usually white) may be placed in the right precordial area, and then lead I becomes the right precordial lead (V4R).10 Simultaneous monitoring of V5, II, and V4R allows for the detection of virtually 100% of all ischemic episodes that are detectable with full 12-lead ECG.10
A central venous line (CVL) is routinely employed in cardiac surgery to allow the reliable administration of cardiovascular medications and heparin and to monitor central venous pressures. Whether the use of a pulmonary artery catheter (PAC) should be routine or used selectively in cardiac surgery has been a matter of debate. In a prospective comparison of a CVL with a PAC in 1094 patients undergoing coronary artery surgery (including the highest-risk patients), Tuman and colleagues11 found that there was no difference in outcome. Likewise, Schwann and coworkers12 reported that, in their experience with 2685 consecutive patients undergoing coronary artery bypass surgery, the use of a PAC was limited to 9% (2.4% unplanned—i.e., converted from a CVL) of the patients and predicted by ejection fraction, Society of Thoracic Surgeons risk score, intra-aortic balloon pump, congestive heart failure, re-do surgery, and New York Heart Association class IV. With such a selective use, their observed-to-expected ratio of mortality as compared with the Society of Thoracic Surgeons database was 0.73. Furthermore, the presence of a PAC has not been shown to influence anesthetic management during induction,13 which is generally considered one of the most risky periods during anesthesia. These studies suggest that the surgeon should be highly selective when choosing coronary surgery patients in whom to use a PAC. However, a PAC, unlike a CVL, can provide information about cardiac output, systemic and pulmonary vascular resistance, and mixed venous saturation, and it can also be used to pace the heart. On the other hand, TEE may negate some of the potential advantages of a PAC over a CVL: cardiac output measured by TEE can be reliable and comparable with that measured by a PAC,14 TEE-guided right and left ventricular oximetry may be feasible,15 and pulmonary artery pressures can be estimated by TEE.16
A detailed review of the indications and capabilities of TEE is beyond the scope of this chapter, but several excellent books about TEE are available. The American Society of Echocardiography and the Society of Cardiovascular Anesthesiologists have published guidelines for the comprehensive intraoperative TEE examination17 and how to be trained and certified for competence in perioperative TEE.9,18 Current indications and use of perioperative TEE are expanding. In addition to serving as a monitor of myocardial function and ischemia and valvular function, TEE is an invaluable guide for the placement of coronary sinus and intra-aortic catheters, for the delineation of the anatomy and pathology of valve lesions, and for before-and-after comparison of myocardial perfusion and function and valvular anatomy and function (Figs. 60-1 and 60-2). In addition, TEE may be used to assess the delivery of cardioplegia,19,20 and to predict changes in regional myocardial function after coronary artery bypass.21
In surgeries of the descending thoracic aorta, cerebrospinal fluid pressure (CSFP) may be measured with an intrathecal catheter. If such a catheter is placed, CSFP should be maintained at less than or equal to 10 cm H2O, and CSF should be allowed to drain when CSFP is greater than 10 cm H2O.22 However, spinal cord perfusion depends not only on CSFP but also on the arterial pressure distal to the distal clamp, and maintenance of the distal arterial pressure may be just as important when using, for example, a partial bypass or a shunt. Whether monitoring of CSFP and drainage of CSF to maintain CSFP at less than 10 cm H2O prevents paraplegia after descending thoracic aortic surgery is still being debated.23
Choice of Anesthetic Agents
High-dose opioid anesthesia was developed in the late 1960s, and it was based on the observation by Lowenstein and colleagues24 that patients on mechanical ventilation after cardiac surgery tolerated large dosages of morphine (0.5 to 3 mg/kg) for sedation and analgesia with minimal hemodynamic effects. Such large dosages were then tried immediately before operation as an adjunct to anesthesia for cardiac surgery, with significant success.25 Opioids by themselves do not ensure amnesia, and they require supplementation with an amnestic such as a benzodiazepine or scopolamine, the latter with unreliable effectiveness and declining popularity. Synthetic opioids such as fentanyl (50 to 100 mg/kg) or sufentanil (5 to 10 mg/kg) provide similar hemodynamic effects but without the fluid retention often seen with high-dose morphine anesthesia. Although highly successful and popular in the 1980s and early 1990s, high-dose opioid anesthesia has gradually given way to a balanced anesthetic technique that involves employing a modest dosage of opioids in conjunction with inhalational anesthetics and other intravenous adjuncts that have a faster onset and offset to facilitate early extubation and discharge from the intensive care unit (ICU).
Although the observation by Reiz and colleagues26 in 1983 that isoflurane may cause coronary steal (a phenomenon in which coronary blood flow is diverted away from the collateral-dependent region of the myocardium) tended to discourage the use of the inhalational agent, subsequent investigators failed to duplicate the findings.27–29 The newer agents sevoflurane and desflurane do not cause coronary steal, either.30,31 In addition, animal studies suggested that isoflurane may preferentially dilate large coronary arteries rather than arterioles (thus mimicking the effect of nitroglycerin32) and normal coronary arterioles rather than arterioles from a collateral-dependent region of the myocardium.33,34 Furthermore, there is mounting evidence that inhalational agents precondition the heart, thus protecting it from subsequent ischemic episodes in a fashion analogous to that of ischemic preconditioning, in which a brief period of ischemia protects the heart from subsequent prolonged ischemic episodes.35–37 Anesthetic-induced preconditioning occurs via activation of ATP-sensitive potassium channels.38 Currently, inhalational agents are the mainstay of anesthesia for cardiac surgery, and these are supplemented with muscle relaxants and a modest amount of opioids for analgesia.
Regional Anesthesia and Analgesia
The use of thoracic epidural anesthesia (TEA) as a supplement to general anesthesia carries several potential advantages. TEA attenuates the sympathetic stress response to surgical stimulation, reduces heart rate and myocardial oxygen consumption, and decreases the release of troponin T.39,40 Postoperatively, TEA provides superior analgesia, thus enabling early extubation and vigorous pulmonary toilet and reducing the incidence of lower respiratory tract infections as compared with conventional intravenous analgesia.41,42 Despite these advantages, TEA has not been widely accepted for cardiac surgery because of concern for a potential epidural hematoma in patients who are to be anticoagulated for CPB. The true incidence of an epidural hematoma in cardiac surgery is not known, because such a complication has not yet been reported in cardiac surgical settings. A mathematical model suggests that the risk ranges from 1:150,000 to 1:1500.43 If TEA is to be used, it should be avoided in patients who are receiving preoperative anticoagulation, and catheter placement may be performed the day before surgery, with postponement of surgery and neurologic monitoring if the placement results in bleeding.
Recently, with the advent of off-pump, minimally invasive cardiac surgery, several European groups have reported using TEA as the sole anesthetic modality without endotracheal intubation in patients undergoing coronary artery bypass surgery,44,45 and aortic valve replacement.46 The authors state that potential advantages of avoiding general anesthesia might include the use of the patient’s mental status as a monitor of cerebral ischemia and achievement of truly fast-track cardiac surgery. In a study of this modality by Karagoz and colleagues,45 8 out of 137 patients went home the day of surgery, and 58 of these 137 did not require any ICU stay, for an overall mean hospitalization of 1 day. However, 39 out of the 137 patients had a pneumothorax, and 4 of them had to be converted to general anesthesia because of pneumothorax-related respiratory difficulties. Potential disadvantages of an awake technique in cardiac surgery include the stress response of an awake patient to surgical maneuvers such as sternotomy, and a delay in surgical progress.47 An awake TEA for cardiac surgery has not yet found acceptance in the United States.
Intraoperative Blood Glucose Control
Acute and chronic hyperglycemia increase the risk of myocardial ischemic injury through several mechanisms.48 Hyperglycemia decreases coronary collateral blood flow, causes endothelial dysfunction, and compromises the salutary effect of ischemic preconditioning or anesthetic-induced pharmacologic preconditioning.49,50 Among oral hypoglycemic agents, sulfonylureas and other insulin secretagogues impair KATP channel activation and may therefore hinder ischemic or pharmacologic preconditioning. Such agents should be discontinued for 24 to 48 hours before surgery and avoided perioperatively.48
Hyperglycemia occurs frequently in patients with and without diabetes during cardiac surgery.51 Intraoperative hyperglycemia is associated with a higher morbidity and mortality in patients undergoing cardiac surgery,52–54 which might suggest that tight intraoperative glycemic control would reduce postoperative complications. Although postoperative strict blood glucose control with insulin has been shown to definitely decrease mortality and morbidity in patients after cardiac surgery,55,56 similar results have not been obtained when the control was initiated during the surgery.57 Furnary and others58 performed a prospective study of nearly 2500 consecutive diabetic patients undergoing cardiac surgery who were randomized to receive either intensive insulin therapy with continuous infusion to maintain blood glucose at less than 200 mg/dL or intermittent subcutaneous insulin injections on a sliding scale. Blood glucose was better controlled with intravenous infusion (85% of patients with blood glucose <200 mg/dL on postoperative day 1, with a mean level of 199 ± 1.4 mg/dL) than with subcutaneous injections (47% of patients with <200 mg/dL, with a mean level of 241 ± 1.9 mg/dL). The incidence of deep sternal wound infection was significantly reduced in the infusion group (0.8% versus 2.0%, P = .01) and approached that of the nondiabetic patients. Mortality was likewise reduced with intensive insulin therapy (3.0% versus 6.1%, P = .03). In a retrospective review of 3554 diabetic patients from the same group,59 mortality was directly related to postoperative mean glucose; it was demonstrated to be 0.9% for those with a level of less than 150 mg/dL, 1.3% for those with 150 to 175 mg/dL, 2.3% for those with 175 to 200 mg/dL, 4.1% for those with 200 to 225 mg/dL, 6.0% for those with 225 to 250 mg/dL, and 14.5% for those with greater than 250 mg/dL.
Intensive insulin therapy to tightly control blood glucose should not be employed intraoperatively only but should be extended into the postoperative period in the ICU. In a prospective study of 1548 patients in the surgical ICU (about two thirds of whom had been through cardiac surgery), intensive insulin therapy to a maintain a glucose level between 80 and 110 mg/dL was associated with a lower mortality as compared with conventional therapy to maintain a glucose level between 180 and 200 mg/dL (4.6% versus 8.0%, P < .04).55 For perioperative glycemic control in diabetes, Martinez and colleagues60 have suggested that all patients, even those not on an oral diet, require a basal insulin infusion, and that exogenous insulin must be given for insulin-deficient patients. Their recommendation was to maintain the blood glucose level at less than 180 mg/mL at all times (between 80 and 110 mg/mL in the ICU), to avoid oral hypoglycemics until the patients are on an oral diet, to provide basal insulin to patients who are insulin deficient,61 and to implement a hypoglycemia-prevention protocol.60
Acid–Base Management during Hypothermia
With pH-stat measurement, the addition of CO2 corrects the hypothermia-induced leftward shift of the oxyhemoglobin dissociation curve and may improve oxygen delivery to the tissues. On the other hand, because the cerebral blood flow response to CO2 is preserved during hypothermia, pH-stat management may lead to excessive cerebral blood flow and interference with cerebral autoregulation and flow–metabolism coupling.62,63 However, clinical neuropsychological outcome has not been shown to differ between pH-stat and alpha-stat management.64
Anticoagulation for Cardiopulmonary Bypass
Heparin sulfate, which is produced from porcine gut or bovine lung, is used to mimic the natural role of heparan sulfate on the vascular endothelial surface. Heparin—although it has no anticoagulant effect by itself—complexes with and enhances the activity of antithrombin-III (AT-III) in irreversibly neutralizing thrombin and factor Xa. Heparin also increases the activity of heparin cofactor II to inhibit the action of thrombin.65 What constitutes a safe and effective level of heparinization for CPB has not been established, in part because of the inconsistent relationship between heparin dosage, plasma heparin concentration, and clinical effect (as measured by the ACT), and in part because the ACT is a nonspecific test affected not only by heparin but also by platelets and temperature.66 Most currently accepted heparin regimens use an initial dosage of 250 to 400 IU/kg to maintain an ACT of greater than 400 to 480 seconds.67 However, animal studies suggest that reduced heparin administration to maintain an ACT between 250 and 300 seconds may be just as adequate.68
Administration of heparin may be followed by hypotension in association with hyperkalemia69; part of the reason for hypotension may be the heparin-induced production of nitric oxide.70 Heparin resistance may be defined as the failure of 500 IU/kg of heparin to prolong the ACT to 480 seconds or greater.71 Factors that predict heparin resistance include AT-III levels of 60% or less, preoperative subcutaneous or intravenous heparin therapy, platelet count of 300,000 or more per milliliter, and age of 65 years or older.72 AT-III levels may be low because of reduced synthesis related to genetic defects or from acquired causes such as liver disease, oral contraceptive use, and disseminated intravascular coagulation; from increased consumption related to disseminated intravascular coagulation, extensive deep vein thrombosis, sepsis, or preeclampsia; or from concomitant use of heparin, nitroglycerin, or the chemotherapeutic agent L-asparaginase. Treatment of heparin resistance with increasingly higher dosages of heparin is intended to maximally bind all available AT-III and may, at times, be effective. Alternatively, the patient may receive fresh frozen plasma73 or AT-III concentrate. In vivo recovery of AT-III activity is about 1.4% per unit of administered AT-III concentrate,74 and the therapeutic goal should be to maintain AT-III activity at more than 80%. After separation from CPB, heparin activity is neutralized by the administration of protamine. Protamine in dosages of up to 1 to 1.3 mg per milligram of heparin (i.e., 100 IU of heparin) may be used, taking into account the heparin activity half-life of 90 minutes. Just as incomplete reversal of heparinization may lead to excessive post-bypass bleeding, excessive protamine can decrease both platelet number and function,75 can prolong ACT,76 and can reduce the effect of thrombin.77 Determining whether a prolonged ACT after an adequate dosage of protamine indicates incomplete heparin reversal or excessive protamine may require measurement of the thrombin time and the heparinase-ACT, as well as the low-level titration of heparin and protamine.
Another cause of postoperative bleeding is heparin rebound. Heparin that has bound nonspecifically to plasma proteins may induce the reappearance of anticoagulant activity after adequate neutralization with protamine and cause excessive post-bypass bleeding. Postoperative protamine infusion eliminates heparin rebound.78
Heparin can cause thrombocytopenia. Heparin-induced thrombocytopenia (HIT) is divided into type I (HIT-I) and type II (HIT-II). HIT-II is a serious adverse effect of heparin exposure that can progress to arterial or venous thrombosis; it has also been called heparin-associated immune thrombocytopenia, heparin-associated thrombocytopenia and thrombosis (HITT), and white clot syndrome.79,80 HIT usually occurs within 4 to 10 days after heparin treatment,79 but it may also occur after heparin has been withdrawn (delayed-onset HIT)81 or rapidly after the start of heparin administration (median time, 10.5 hours for early-onset HIT).82 It is useful to know the duration of the prior exposure to heparin and platelet count.
Unfractionated heparin (UFH), which is used for anticoagulation during CPB, is associated with a higher risk for HIT than low-molecular-weight heparin (LMWH).83 The incidence of clinically significant HIT was low in cardiac surgery with CPB,84 although the incidence of heparin-dependent immunoglobulin G (IgG) antibodies was 15% to 20% in patients undergoing cardiac surgery.85 Patients who have a history of HIT but are negative for HIT antibodies at the time of surgery, and who require CPB, have been successfully anticoagulated with a brief course of treatment with UFH, without complications.86 If perioperative HIT is diagnosed, all exposure to heparin should be immediately ceased, and argatroban, lepirudin, or bivalirudin should be considered for prophylactic therapy of thrombosis until the platelet count has recovered.
Five types of protamine-related adverse reactions have been recognized. First, rapid administration of protamine (>5 mg/min) may at times induce the release of histamine from mast cells and thus cause systemic hypotension.87 Second, protamine may act like an antigen and initiate an immunoglobulin (Ig)G- or IgE-mediated anaphylactic reaction, especially in diabetic patients who take protamine-containing insulin preparations,88–90 and possibly in those with a true fish (not shellfish) allergy or those who have just had a vasectomy.87 Third, protamine-heparin complexes may activate the complement system, thereby leading to an anaphylactoid reaction.91,92 Fourth, protamine-heparin–mediated complement activation may lead to thromboxane A2 generation, precipitating catastrophic pulmonary hypertension93; this is in contrast to the low systemic and pulmonary pressures seen in classic anaphylactoid reactions. In a pig model, this reaction was preventable by prior administration of indomethacin or a thromboxane A2 receptor antagonist.94 Last, fulminant noncardiogenic pulmonary edema and systemic anasarca have been reported 15 minutes to more than 1 hour after the administration of protamine.87 Treatment of a protamine reaction is supportive, and various agents (e.g., diphenhydramine, steroids) and hemodynamic support have been tried. In a patient with a history of prior adverse reaction to protamine, it should be determined whether the reaction was immunologically mediated. Levels of IgG, IgE, thromboxane, and C5a at the time of the reaction, if available, may be useful, but a skin test or enzyme-linked immunosorbent assay has not been found to be useful.95 When the cause of the reaction is uncertain, some people advocate pretreatment of the patient with steroids and histamine blockers and the slow administration of protamine. If a true immunologically mediated protamine reaction occurred, it would be best to avoid it. Protamine alternatives that are being tested include recombinant platelet factor 4,96 hexadimethrine,97 heparinase,98 and a heparin removal device that uses a venovenous circuit with a poly-L-lysine-agarose surface.99
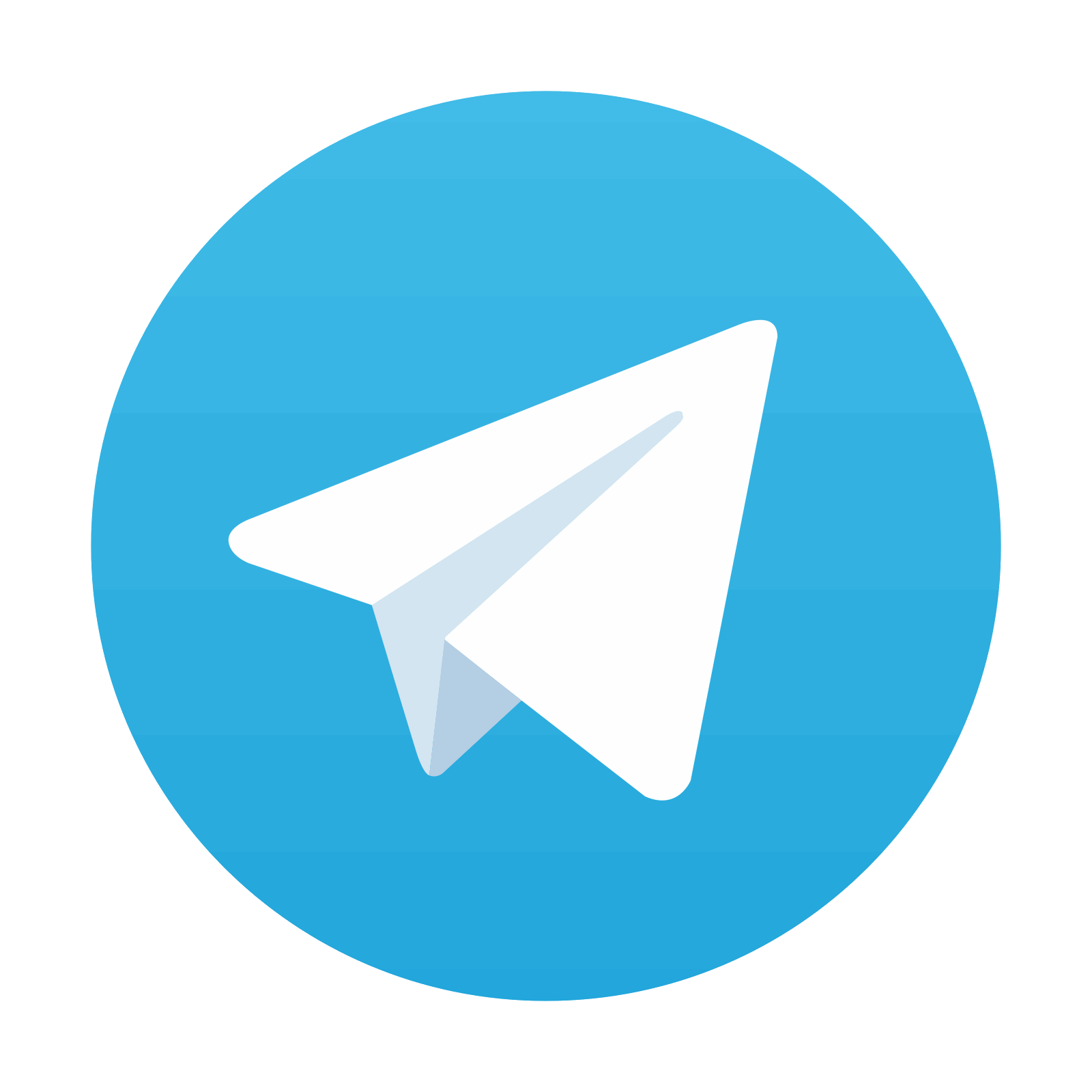
Stay updated, free articles. Join our Telegram channel

Full access? Get Clinical Tree
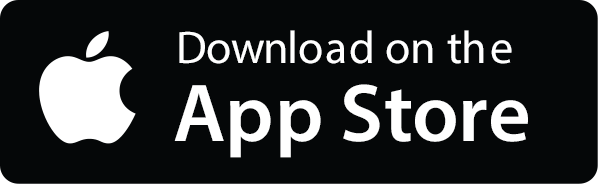
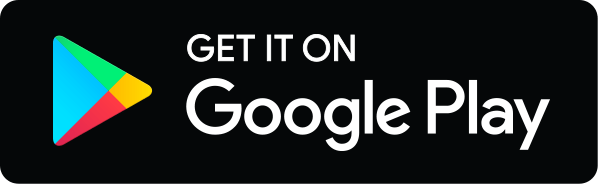