Background
Stress echocardiography has been advocated for the detection of abnormal myocardial function and unmasking diminished myocardial reserve in pediatric patients. The aim of this study was to create a simplified index of myocardial reserve, derived from the myocardial inotropic response to peak semisupine exercise in healthy children, and illustrate its applicability in a sample of pediatric oncology patients.
Methods
In this prospective analysis, children (7–18 years of age) with normal cardiac structure and function performed semisupine stress echocardiography to volitional fatigue. The quotient of wall stress at peak systole and heart rate–corrected velocity of circumferential fiber shortening were calculated at baseline and at peak exercise, the difference of which was termed the index of myocardial reserve (IMR). The IMR was also calculated in a retrospective sample of pediatric oncology patients with normal resting left ventricular function who had received anthracycline treatment and had performed the same exercise protocol to illustrate utility.
Results
Fifty healthy subjects (mean age, 13.2 ± 2.6 years) and 33 oncology patients (mean age, 12.7 ± 4.0 years) were assessed. In the healthy children at peak exercise, heart rate–corrected velocity of circumferential fiber shortening significantly increased (from 1.17 ± 0.17 to 1.58 ± 0.24 circ · sec −1 , P < .001), while the quotient of wall stress at peak systole significantly decreased (from 75.3 ± 17.1 to 55.3 ± 13.8 g · cm −2 , P < .001), shifting the plot of the relationship between the two parameters upward and to the left. The mean IMR was −30.8 ± 17.8, and the normal distribution ranged from −4.7 (fifth percentile) to −67.3 (95th percentile). The IMR was abnormal in 10 oncology patients who were treated with anthracyclines.
Conclusions
The authors have developed a novel IMR. Relative to the normal distribution of this IMR in healthy subjects, it is possible to identify patients with abnormal myocardial reserve. Thus, this study demonstrates the application of the IMR to aid in clinical decision making in individual patients.
Highlights
- •
Semisupine cycle ergometry is ideal for imaging during exercise in pediatric patients.
- •
Stress echocardiography may unmask diminished myocardial reserve.
- •
A novel index of myocardial reserve has been developed, providing clinicians with a simplified approach to assess patients.
Exercise requires an effective increase in myocardial contractility to maintain and enhance stroke volume and, ultimately, cardiac output to meet increased hemodynamic and metabolic demands. Exercise testing has been used as an indirect measure of cardiac function in both adult and pediatric cardiac disease, and exercise capacity is used to assist in decision making for transplantation. The rapid reduction in heart rate and the delay in imaging the heart immediately upon the cessation of treadmill exercise make this mode unsuitable for stress echocardiography. This has resulted in a preference for supine or semisupine cycle ergometry for imaging during exercise in the pediatric setting. Exercise stress echocardiography has become an important diagnostic tool for unmasking abnormal myocardial reserve that may not be identified in patients at rest. Although measures of cardiac performance such as ejection fraction are informative, changes in intrinsic cardiac contractility cannot be discerned from these load-dependent measures of systolic function. Recent studies have assessed the utility of more advanced methods of echocardiography, such as tissue Doppler and speckle-tracking imaging, to examine local myocardial mechanics and the physiologic response to exercise. However, technical limitations may challenge the utility of these methods at peak exercise, and clinicians are more versed in the left ventricular (LV) response to exercise measured by the more traditional measures of contractility that evaluate global LV function. Although the physiologic response of these traditional measures of LV function to exercise has been described, the minimal change of function with exercise for an individual patient has not been determined. This information would support clinical decision making for or against intervention or the suitability of more treatment with potentially cardiotoxic therapy.
The best noninvasive measure of intrinsic LV contractility that has been developed is the relationship between heart rate–corrected mean velocity of circumferential fiber shortening (MVCFc) and the quotient of wall stress at peak systole (σPS). Previously, our group published the variability of normal resting MVCFc-σPS values. This preload-independent, afterload-adjusted index has been used to describe resting myocardial contractility in children with cardiomyopathy, neonates with and without patent ductus arteriosus, preterm and term infants, children with congenital heart disease, and patients with muscular dystrophy. Furthermore, MVCFc-σPS has been a reliable surrogate of cardiac contractility during exercise, and normal values have been provided during supine exercise in healthy children. This type of cross-sectional data may illustrate when a patient group shows reduced contractility compared with healthy control subjects, as we have done previously, but these data cannot be applied for clinical decision making in individual patients. For example, a group of pediatric oncology patients who have been given high-dose anthracycline therapy will display lower myocardial reserve to an exercise challenge compared with a group of children who received low-dose anthracycline treatment. However, cumulative anthracycline dose is only one of various established risk factors for developing cardiotoxicity, and other unknown risk factors and genetic mechanisms have limited our ability to accurately determine those children at most risk for developing anthracycline-induced cardiotoxicity. Accordingly, the purposes of this research were to describe the change in contractility in individual patients and to develop a clinically useful index of myocardial reserve (IMR) to simplify clinical interpretation of myocardial performance during semisupine exercise derived from the MVCFc-σPS relationship. A secondary purpose of this study was to reevaluate the stress echocardiographic data in a group of pediatric oncology patients who were treated with chemotherapeutic agents of the anthracycline class to see how the IMR can more accurately gauge myocardial reserve, which may be important in guiding further chemotherapy.
Methods
Subjects
Healthy children and adolescents (7–18 years of age) free of known medical conditions or contraindications to exercise were prospectively recruited for this study. Subjects were excluded from the study if they presented with any abnormality of cardiac structure or function as determined from physical examination, electrocardiography, and resting echocardiography as assessed by a pediatric cardiologist. In addition, we conducted a retrospective analysis of 33 pediatric oncology patients (7–18 years of age, 50% girls) treated with anthracyclines who underwent the same exercise protocol in our laboratory. This patient cohort consisted of various cancer diagnoses (leukemia; lymphomas; bone, brain, and soft tissue tumors; and skin cancer), and all children were in complete remission and asymptomatic with normal resting indexes of LV function at the time of testing. Patients were divided into subgroups on the basis of cumulative anthracycline dose (low, <260 mg/m 2 ; high, >260 mg/m 2 ), as this represents the cutoff associated with an increased risk for cardiotoxicity.
Subjects consented to one visit including measures of body height and mass, systolic blood pressure (SBP) and diastolic blood pressure, heart rate, and echocardiographic imaging, assessed at baseline and at peak exercise. Body mass index was calculated as weight (kg)/height 2 (m 2 ) and then standardized for sex and age using data from US Centers for Disease Control and Prevention growth charts. The study protocol was approved by the University of British Columbia’s Clinical Research Ethics Board.
Exercise Protocol
The exercise protocol involved a progressive maximum exercise test on a cycle ergometer in the semisupine position with the subject’s trunk elevated to a 45° angle with the heart approximately 15 cm above the level of the crank axis of the ergometer (Angio Ergometer and Stress Echo Table; Lode BV, Groningen, The Netherlands). Workloads began at and increased 20, 30, or 40 W per stage, depending on the age and size of the child, for 3-min stages, pedaling at a cadence of 60 rpm throughout the test, until volitional fatigue. Simultaneous blood pressure assessment (auscultation) and echocardiography were performed at baseline, 1.5 min into each stage of exercise, at peak exercise, immediately after exercise, and 3 min after exercise. The electrocardiogram (Case 8000 Stress System; GE Medical Systems, Milwaukee, WI) was monitored continuously throughout the exercise test. Subjects were instructed to provide a warning to the investigators when they felt they were within 90 sec of their maximal exercise limit, so that final echocardiographic images and blood pressure could be obtained at peak exercise. Verbal encouragement was provided to all subjects near the end of the test. Maximal effort was confirmed by the inability of the participant to keep a minimum cadence of 60 rpm despite verbal encouragement. In a subset of the children, cardiopulmonary testing occurred simultaneously with the stress echocardiographic protocol, with expired gases analyzed by a metabolic cart (Moxus Metabolic Cart; AEI Technologies, Pittsburgh, PA). In these children, additional parameters such as ventilation and respiratory exchange ratio confirmed maximal effort.
Echocardiography
Stress echocardiography was performed by an experienced clinical pediatric ultrasonographer using a Vivid system (GE Vingmed Ultrasound AS, Horten, Norway) and 3.5-MHz transducer. M-mode images were acquired in the parasternal short-axis view for the assessment of LV dimension at end-systole (LVES) and LV dimension at end-diastole (LVED) and posterior wall thickness in systole (LVPWs) at the level of the papillary muscles. Care was taken to ensure that the position of the ventricles was consistent at each measurement. The diameter of the aortic annulus was measured at the base of the valve using a parasternal long-axis view, and an apical four-chamber view was used to obtain ascending aortic Doppler flow. Peak aortic velocity, the velocity-time integral, and ejection time were obtained and recorded.
Data Analysis
Data were analyzed from the images obtained at baseline and peak exercise. Ventricular dimensions were indexed to body surface area. Stroke volume was determined from the Doppler signal using the velocity-time integral and aortic cross-sectional area (π · aortic diameter 2 /4) and indexed to BSA (SVI). Cardiac index was calculated as the product of SVI and heart rate. Fractional shortening (FS) was calculated as LVED − LVES/LVED × 100%. MVCFc (circ · sec −1 ) was calculated by dividing FS by ejection time corrected for heart rate (calculated as ejection time divided by the square root of the R-R interval), and σPS (g · cm −2 ) = SBP × LVES × 1.36/4 × LVPWs[1 + (LVPWs/LVES)]. The relationship of MVCFc and σPS at baseline and peak exercise were plotted to show the mean of control, low-dose, and high-dose anthracycline exercise response. The IMR was calculated by subtracting the σPS/MVCFc ratio at peak exercise from the σPS/MVCFc ratio at baseline: IMR = (σPS/MVCFc) P − (σPS/MVCFc) B . The IMR was calculated for the healthy children and pediatric oncology patients and plotted by group.
Statistical Analysis
Outcome variables were assessed for normality using the Shapiro-Wilk statistic. Paired-samples t tests were performed to evaluate the effect of exercise on blood pressure (SBP and diastolic blood pressure), cardiac dimensions (LVED index and LVES index), heart rate, cardiac volumes (SVI and cardiac index), and cardiac function (MVCFc, σPS, and σPS/MVCFc). A subgroup analysis was performed to compare workload and hemodynamic variables between subjects who underwent metabolic testing and those who did not. All values in the text and tables are expressed as mean ± SD unless otherwise specified. Multiple linear regression was used to assess the associations between contractility indices, age, and sex and change in stroke volume. The MVCFc-σPS relationship at baseline and peak exercise for all groups are illustrated as mean ± SD. Percentiles were calculated from the normal distribution of IMR of the control group. All analyses were conducted using SPSS version 20.0 (IBM, Armonk, NY), and statistical significance was set a priori at P < .05.
Results
Healthy Children
Fifty healthy subjects were recruited for this study, including 32 girls and 18 boys (mean age, 13.2 ± 2.6 years; Table 1 ). Metabolic data were obtained in 20 of the subjects throughout exercise, with maximal ventilation (70.8 ± 16.6 L/min), peak oxygen uptake (39.0 ± 7.6 mL · min −1 · kg −1 ), and respiratory exchange ratio (1.06 ± 0.07) reaching values expected for children and adolescents for this mode of exercise. Average work performed was 402.8 ± 120.2 W, and peak heart rate was 85.7 ± 5.6% predicted. The mean maximal heart rate achieved by the 20 children who were assessed metabolically and those who were not metabolically assessed did not differ (180 ± 13 vs 184 ± 8 beats/min, P < .05). Heart rate, SBP, SVI, cardiac index, and FS significantly increased from baseline to peak exercise ( P < .001 for all; Table 2 ), as did diastolic blood pressure ( P = .007). Change in heart rate was positively associated with age ( r = 0.45, P = .001). At peak exercise, MVCFc increased, while σPS decreased ( P < .001; Table 2 ) compared with the values at baseline. Linear regression revealed that change in MVCFc was significantly associated with change in SVI, accounting for age, sex, and σPS in the model (standardized β = −0.366, P = .009). No other associations were found.
Characteristic | Healthy children | Oncology patients |
---|---|---|
( n = 50) | ( n = 33) | |
Female sex | 32 (64) | 16 (50) |
Age (y) | 13.2 ± 2.6 | 12.7 ± 4.0 |
Height (cm) | 158.0 ± 12.4 | 150.9 ± 21.5 |
Weight (kg) | 48.7 ± 11.2 | 48.4 ± 19.9 |
BMI Z score | −0.02 ± 0.98 | 0.35 ± 1.16 |
Anthracycline dose (mg/m 2 ) | Low ( n = 15), 189.6 ± 101.6; high ( n = 18), 398.5 ± 50.8 |
Baseline | Peak exercise | Δ | |
---|---|---|---|
SBP (mm Hg) | 118.3 ± 10.5 | 177.7 ± 23.0 ∗ | 59.4 ± 21.3 |
DBP (mm Hg) | 73.1 ± 7.5 | 76.4 ± 10.1 † | 3.3 ± 8.6 |
HR (beats/min) | 78 ± 13 | 182 ± 11 ∗ | 105 ± 18 |
SVI (mL/m 2 ) | 36.5 ± 9.0 | 42.6 ± 9.1 ∗ | 6.1 ± 6.7 |
Cardiac index (L · min −1 /m 2 ) | 2.87 ± 0.95 | 7.75 ± 1.74 ∗ | 4.88 ± 1.35 |
LVED index (cm/m 2 ) | 3.88 ± 0.80 | 3.64 ± 0.69 ∗ | −0.24 ± 0.33 |
LVES index (cm/m 2 ) | 2.47 ± 0.54 | 1.78 ± 0.40 ∗ | −0.69 ± 0.30 |
FS (%) | 36.4 ± 4.2 | 51.0 ± 5.1 ∗ | 14.6 ± 4.9 |
MVCFc (circ · sec −1 ) | 1.17 ± 0.16 | 1.58 ± 0.24 ∗ | 0.42 ± 2.0 |
σPS (g · cm −2 ) | 75.3 ± 17.1 | 55.3 ± 13.8 ∗ | −20.1 ± 14.2 |
σPS/MVCFc (g · cm −2 · s · circ −1 ) | 66.7 ± 21.3 | 35.4 ± 11.3 ∗ | −30.8 ± 17.8 ‡ |
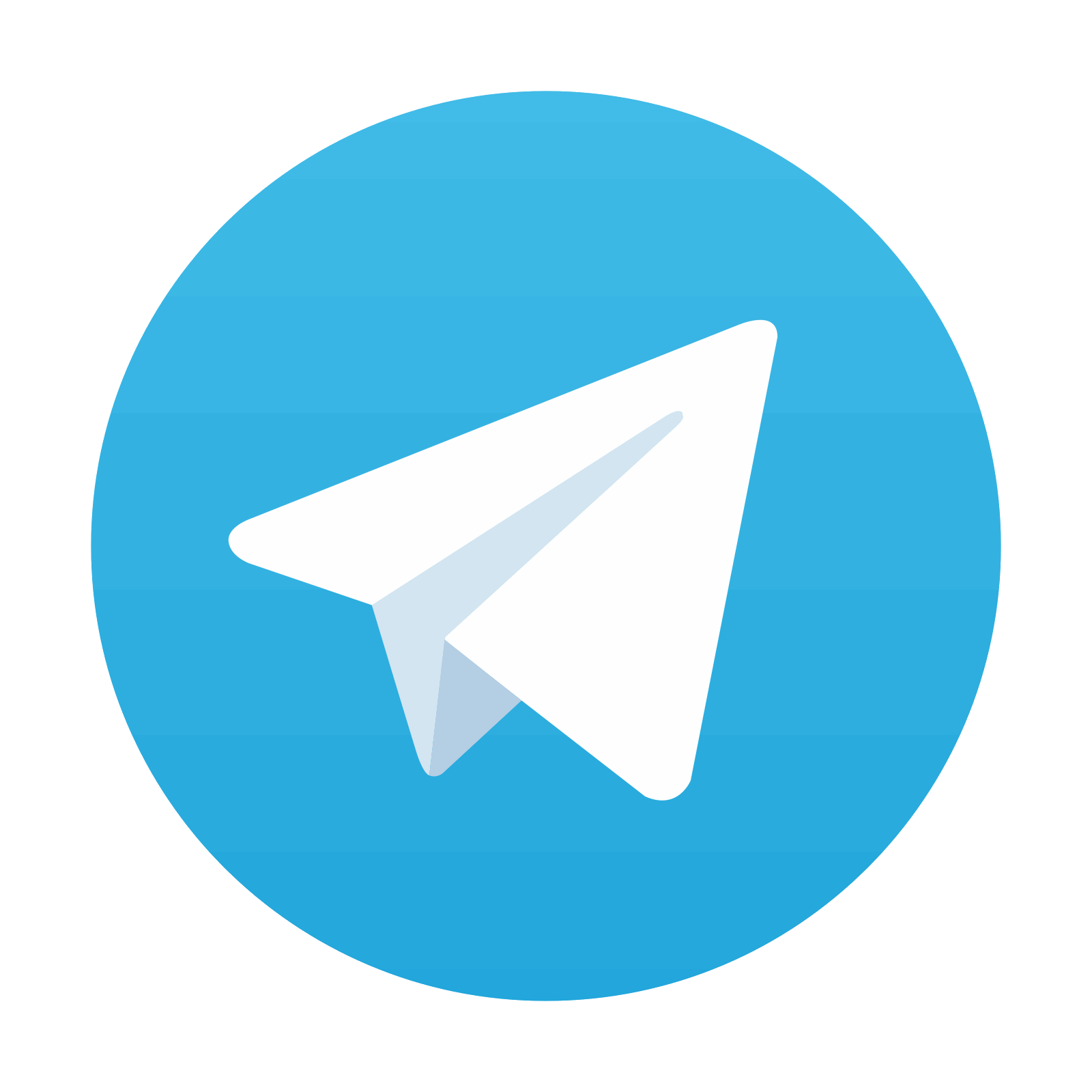
Stay updated, free articles. Join our Telegram channel

Full access? Get Clinical Tree
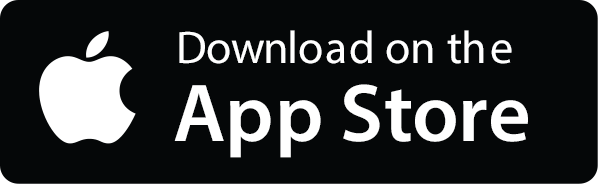
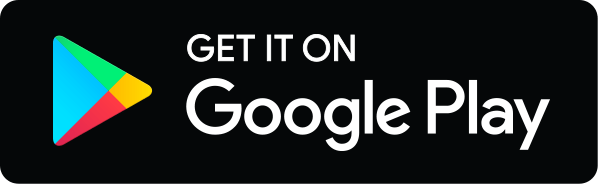