ANTIARRHYTHMIC DRUG THERAPY
Case presented by:
A.The Na channel shows transition between open and inactivated states.
B.At any point in time, the Na channel may be open or inactivated.
C.Tonic block refers to the drug-induced reduction of channel availability after a long rest period.
D.Use-dependent block implies progressive increase in Na+ current during repetitive depolarization.
E.The association rate constant is more helpful in classifying antiarrhythmic drugs than the dissociation rate constant.
Antiarrhythmic Drugs and Molecular and Cellular Targets
Na+ Channels
The modulated receptor hypothesis suggests that:
- Na channels exist in 3 primary states: rested (R, a closed state most prevalent at negative membrane potentials); inactivated (I, a closed state at depolarized membrane potentials); and open or activated (A). This means that the R state is more prevalent during diastole, the A state during the upstroke, and the I state more prevalent during the plateau or when the tissue is depolarized. Each Na channel shows a transition between the R, A, and I states. A substantial fraction of the Na channels undergo inactivation before they open. The implication of this is that both processes, that is, channel opening or inactivation, occur simultaneously. Tonic block refers to the drug-induced reduction of channel availability after a long rest period.
- Drugs can interact with resting, activated, and inactivated states of Na+ channels. The voltage dependence of inactivation of drug-bound channels is shifted to more negative potentials.
- Affinity of the drugs is greater for activated and inactivated states than the resting state.
- Activated state blockers include class IA and class IC drugs, such as disopyramide, procainamide, quinidine, flecainide, and propafenone. Inactivated state blockers include amiodarone, lidocaine, and mexiletine.
- Presence of two or more drugs usually results in additive block. Mexiletine plus quinidine or disopyramide may have more effect than either of them alone. A drug with fast association and dissociation kinetics may displace a drug with slower kinetics from the receptor. As Na+ ions cross the membrane by binding to multiple pores, Na+ ion-containing solutions could be used to reverse the side effects of Na+ channel-blocking drugs.
- Conditions such as ischemia or hyperkalemia cause membrane depolarization, leading to an increased number of Na+ channels in the inactivated state. This explains the efficacy of drugs such as lidocaine and amiodarone in these clinical settings.
- Drugs fall into three groups according to the rate at which they block. Class IB drugs, such as lidocaine, have fast kinetics. Class IA drugs, such as quinidine, have intermediate kinetics, and class IC drugs, such as flecainide, have slow kinetics. Drugs fall into three groups based upon the development of a steady state of frequencies over a wide range.
- Group I: Fast association and dissociation constants. Class IB drugs, such as lidocaine and mexiletine, fall into this group.
- Group II: Slow association and dissociation constants. This group includes class IA and class IC drugs, such as quinidine, procainamide, and flecainide.
- Group III: Slow association and fast dissociation constants. Disopyramide and propafenone are included in this group.
- Group I: Fast association and dissociation constants. Class IB drugs, such as lidocaine and mexiletine, fall into this group.
- Use-dependent block is seen in group II where, although there is a significant block at rest, the block is further increased during faster heart rates. There is a progressive decrease of Na+ current during repetitive depolarization. Although Group III drugs have increased block at rest, similar to Group II, no additional block occurs at increased heart rates.
- Tonic block refers to continuous access to binding site in the absence of activation.
K+ Channel Blockers
- K+ channel blockers prolong action potential duration (APD) and increase refractoriness. Sotalol, amiodarone, and bretylium are relatively nonselective. Dofetilide selectively blocks the delayed rectifier IKr.
- As opposed to Na+ channel blockers, pure K+ channel blockers (eg, sotalol) produce maximal prolongation of APD at slower rates and have diminished effects at faster rates. This behavior is referred to as reverse use-dependent. Amiodarone, however, does not show reverse use-dependence and maintains its efficacy over a wide range of cycle lengths.
Ca++ Channel Blocking Agents
The L-type Ca++ channel has three different kinetic states depending upon the transmembrane voltage changes:
- As voltage descends below –40 mV, the channel is in resting state. It goes through the activation and inactivation stages during and after depolarization. Transition to inactivated state is voltage- and Ca++ dependent. Increased intracellular Ca++ accelerates the development of the inactivation state.
- Ca++ channels can be blocked at distinct binding sites by three major classes of drugs: dihydropyridines, phenylalkalamines, and benzothiazepines. Nifedipine belongs in the dihydropyridine class, verapamil in the phenylalkalamine class, and cardiazem in the benzothiazepine class.
- The receptor for dihydropyridines is located extracellularly. They have increased affinity for the channel in the inactivated state. They unbind rapidly from their receptors and do not show use-dependence.
- Phenylalkalamines bind to receptors located on the intracellular surface of Ca++ channels. They interact with the channels in the open (activated) state. They unbind slowly (slow dissociation constant) and show use-dependent block, that is, more channel blockade at faster rates.
- Benzodiazepines demonstrate kinetics intermediate between dihydropyridines and phenylalkalamines. They work in the inactivated state.
Question No. 2: With regard to impulse propagation in the heart:
A.Enhanced normal automaticity results from Ca++ overload.
B.Accelerated ventricular rhythm postmyocardial infarction is an example of triggered activity and results from decreased refractoriness.
C.Early afterdepolarizations (EADs) occur during phase 2 of action potential.
D.Delayed afterdepolarizations (DADs) occur due to outward Na+ current.
Mechanisms of arrhythmias include both enhanced normal automaticity and abnormal automaticity, triggered activity based upon EAD and DAD, and reentry. Some other mechanisms include reflection and parasystole.
Cellular Mechanism of Normal and Abnormal Automaticity
Normal Automaticity
In the sinoatrial node, there is a slow diastolic (phase 4) depolarization from a maximum diastolic potential of about –60 mV. This is characteristic of automatic cells. There is closure of K+ channels, and pacemaker-inward current If, carried by Na+ and K+ ions, is activated at about –45 mV. If is also a major current responsible for diastolic depolarization in Purkinje cells. Ca++ channels in the sinus node and Na+ channels in the Purkinje cells cause rapid depolarization. Catecholamines increase the heart rate by moving the potential for If activation to more positive potentials and by increasing ICa and IK.. Parasympathetic stimulation causes bradycardia by: (1) activating K+ current IKACH; (2) reduction of ICa; and (3) reduction of If. Arrhythmias associated with enhanced normal automaticity include inappropriate sinus tachycardia. The vulnerable parameter is phase 4 depolarization.
Abnormal Automaticity
Abnormal automaticity is the mechanism by which spontaneous impulses are generated in tissues that are partially depolarized due to disease. During reduction of maximum diastolic potential from –75 mV to –50 mV as a result of ischemia, depolarization during phase 4 cannot occur due to If. Rather, it occurs due to decay of IK and the action potential generated by ICa-L. Examples of arrhythmias caused by abnormal automaticity are: certain ectopic atrial tachycardias and accelerated idioventricular rhythms, and ventricular tachycardia (VT) occurring 24 to 72 hours after myocardial infarction.
Triggered Activity
Triggered activity refers to arrhythmias that arise as a consequence of depolarization. Afterdepolarizations can interrupt or occur after completion of repolarization and are termed EAD or DAD. EADs arise from the reduced level of membrane potential during phase 2 or from a more normal voltage potential during phase 3. The primary mechanisms include reduction of IK and abnormal prolongation of inward currents carried by Ca++ or Na+ or both. DAD is caused by the transient inward Na+ current ITO that is carried by the Na+/Ca++ exchanger, and is small or absent under normal physiological conditions.
EADs are secondary depolarizations arising from the incomplete repolarized membrane. EADs are associated with APD prolongation, which is reflected as QT interval prolongation on the surface ECG. This may cause torsade de pointes. Blockade of outward K+ current during repolarization with drugs, such as sotalol or dofetilide, or enhancement of inward current, such as Ca++ or Na+, will cause QT prolongation. Rapid upstroke of EADs due to ionic currents may occur due to Ca++ channels in the early phase and Na+ channels at more negative potentials.
DADs are secondary depolarizations that arise from a fully repolarized membrane. DADs may arise under conditions causing intracellular Ca++ overload such as ischemia or digitalis intoxication. DADs can also occur during catecholamines and positive inotropic agents. Acceleration of the heart rate can increase the amplitude of DADs, eventually making them reach threshold potential.
Reentry
Circus movement tachycardia causing reentry has an excitable gap. A premature extrastimulus can result in premature excitation of the reentrant loop. For reentry to occur, the length of the path must exceed the wavelength determined by effective refractoriness and there must be unidirectional block. Electrophysiologic parameters such as conduction are anisotropic. Anisotropy is determined by fiber orientation (see previous cases for more details).
Wavelength equals conduction velocity × refractory period. This relationship between wavelength and path length can be achieved by slowing conduction uniformly along the whole path length, slowing conduction locally, or shortening the refractory period. Cellular depression of the level of INa and ICa may cause the slowing of conduction. Depending upon the speed of impulse propagation and the effective refractory period, an excitable gap may be present in the reentrant circuit. The excitable gap may be present at some sites but not at others. Reentry can be suppressed by altering the two vulnerable periods, conduction and refractoriness. Examples of arrhythmias with a long excitable gap include typical atrial flutter and monomorphic VT. Depressing conduction will help alleviate these arrhythmias. Arrhythmias such as atrial fibrillation, polymorphic VT, and ventricular fibrillation (VF) have a short excitable gap. Prolongation of refractory period will be needed to suppress these arrhythmias.
Reflection
Reflection can be seen in a single bundle. Reflection is caused by electrotonic transmission across an inexcitable segment in a linear bundle. The proximal fibers recover excitability due to delay in impulse transmission. This allows these fibers to be made available for excitation by current flowing retrogradely across the inexcitable zone.
Parasystole
During parasystole, there is abnormal automatic impulse initiation, which is affected by exit block. For example, an automatic focus in Purkinje fibers may be surrounded by an area of inexcitable fibers, thus protecting this focus from sinus depolarizations. However, subthreshold depolarizations can delay or accelerate the automatic firing. Parasystole is usually benign but could conceivably provide the trigger for sustained arrhythmias.
Question No. 3: During ischemia, the following is true:
A.There is increase in action potential amplitude and APD.
B.Channels, such as IKATP, are open.
C.There is decrease in gap junction resistance.
Ischemic Changes in the Myocardium
Several changes occur at the cellular level during ischemia, leading to ischemia-related electrophysiologic changes such as vulnerability to VT/VF.
- Initial ischemic changes occur in the subepicardium.
- There is a decrease in action potential amplitude and APD. The cells depolarize from –60 to –50 mV within 4 to 10 minutes.
- The K+ channel IKATP is normally closed by intracellular ATP but opens up when ATP levels are low, such as during ischemia or hypoxia. The channel is also present in pancreatic cells and is blocked by sulfonylureas, such as glibenclamide and glipizide. During myocardial ischemia, there is a rapid loss of intracellular K+, causing membrane depolarization, slowing of conduction, and altered refractoriness. Increased K+ conductance is due to low ATP levels reducing ATP-inhibition of IKATP levels, leading to action potential shortening during acute myocardial ischemia.
- Metabolic acidification occurs due to hydrolysis of ATP and extracellular transfer of K+.
- There is an increase in gap junction resistance. This causes electrical cell-to-cell uncoupling and conduction block. The initial phase of conduction block occurs due to cellular inexcitability.
- Sympathetic activity is enhanced—refractory period may increase due to inhomogeneous effects.
- Accelerated idioventricular rhythm is a form of reperfusion arrhythmia found after myocardial infarction. The mechanism is enhanced automaticity, and beta-adrenoceptor blockade can suppress this arrhythmia.
Question No. 4: Which of the following is true for dofetilide?
A.Properties are concentration dependent.
B.It is predominantly excreted renally as a metabolite.
C.Verapamil use is contraindicated.
D.A and C.
Dofetilide
- Dofetilide has class III (APD prolongation) properties, which are concentration dependent. The drug acts by selectively blocking the delayed rectifier K+ current IKr.
- It does not cause changes in PR interval or QRS duration. It does not increase defibrillation thresholds (DFTs). It has no negative ionotropic effect.
- Dofetilide has >90% bioavailability. About 80% of the substance is excreted by the kidneys in an unchanged state. Twenty percent is excreted as inactive or minimally active metabolites. A small amount of the drug is metabolized in the liver.
- Increased QT interval is directly related to dofetilide dose and plasma concentration.
- Dose-calculated creatinine clearance is
in males. In females, creatinine clearance is calculated by multiplying the formula result by 0.85.
- When creatinine clearance is <20 mL/min, dofetilide is contraindicated and recommended dose with 20 to 40 mL/min is 125 mcg twice daily; for creatinine clearance of 40 to 60 mL/min, it is 250 mcg twice daily; and for creatinine clearance > 60 mL/min, the dose can be 500 mcg twice daily.
- Dofetilide is contraindicated in patients with congenital or acquired long QT syndrome. Concomitant use of verapamil and cationic transport inhibitors such as cimetidine is also contraindicated.
Question No. 5a: Which of the following is true?
A.Class I drugs depress phase 0.
B.Class I drugs can slow conduction.
C.Both are true.
Classification of Antiarrhythmic Drugs
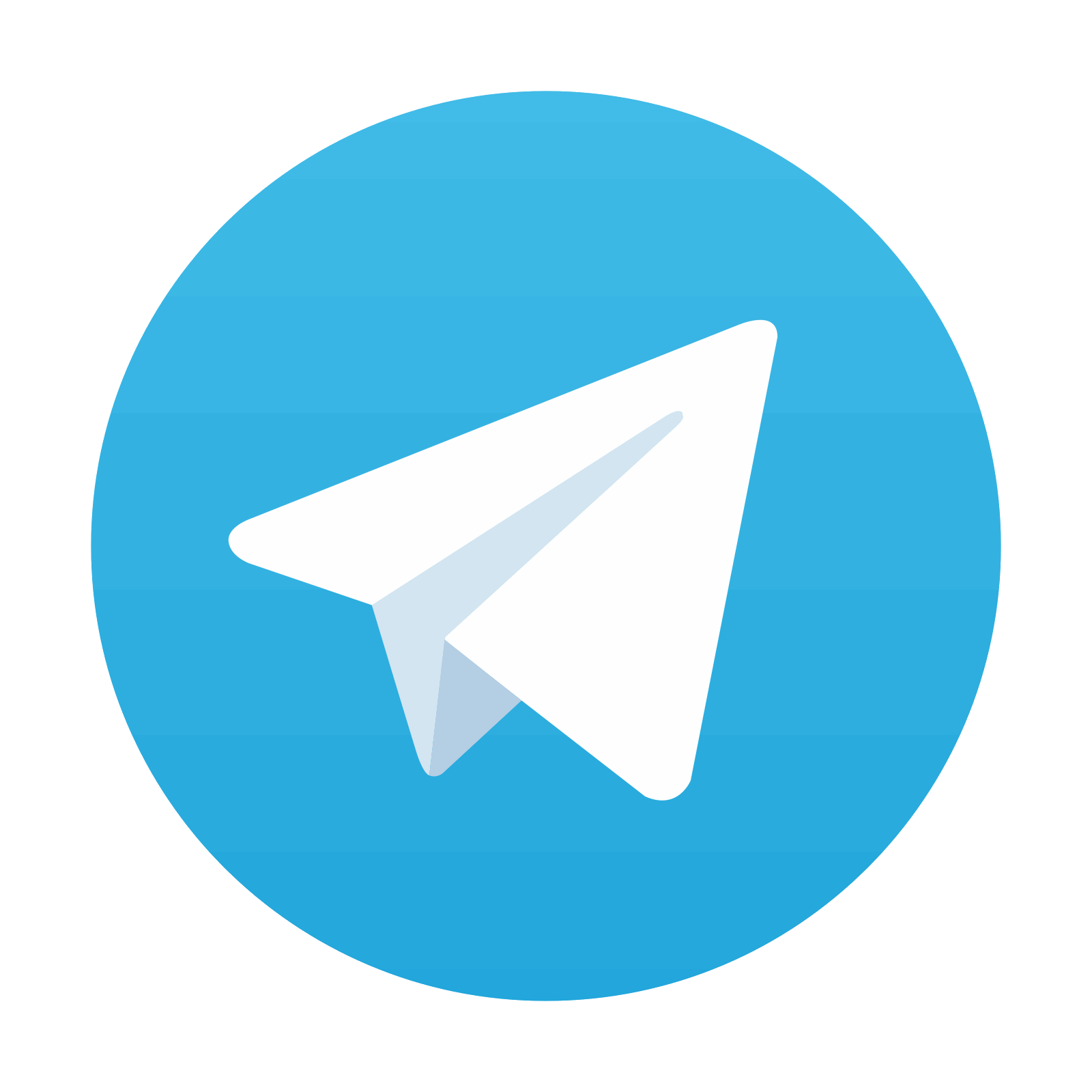
Stay updated, free articles. Join our Telegram channel

Full access? Get Clinical Tree
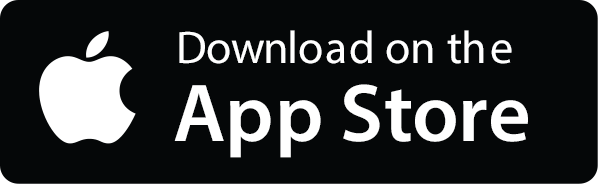
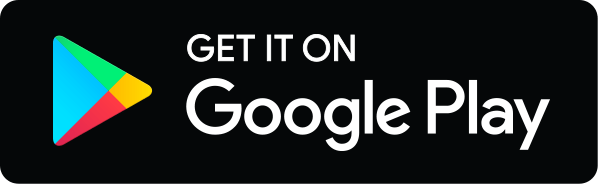