CHAPTER 24 Paul M. Johnson, Shriti Mehta, Prashant Kaul and George A. Stouffer The ability accurately to assess the physiologic significance of a coronary stenosis in clinical practice is critical. For clinicians in the cardiac catheterization laboratory, it is difficult to determine by angiography alone if an intermediate coronary lesion is responsible for ischemic symptoms. Lesions that are responsible for inducible ischemia are associated with worse outcomes, and those patients may benefit from revascularization, either by percutaneous intervention (PCI) or coronary artery bypass grafting (CABG). On the other hand, lesions that do not produce inducible ischemia are associated with a favorable prognosis and medical treatment alone is reasonable. Due to the practical limitations of the Doppler wire and other methods to calculate coronary flow reserve (CFR; Figure 24.1), the use of a pressure transducer to measure pressure‐derived fractional flow reserve (FFR) has gained wide acceptance as a clinically useful tool to assess the hemodynamic significance of coronary stenosis. Early efforts to measure pressure gradients across a stenosis with small fluid‐filled catheters were limited because the catheters were large enough to increase artifactually the translesional gradient in diseased arteries. The development of a pressure transducer mounted on a 0.014 inch coronary guidewire overcame this limitation and made measurement of the pressure gradient across a coronary lesion efficient and safe. Figure 24.1 Simplified graph of coronary flow reserve. The ability to increase coronary flow is essential to meet metabolic demands during physiologic stress. Resistance to flow from a stenosis in an epicardial artery can compromise the ability of coronary flow to increase. The basic principle of FFR is that when resistance is constant, changes in pressure are proportional to changes in flow. Since pressure is easier to measure than flow, FFR has replaced CFR for clinical use. Ohm’s Law, which describes the relationship between pressure, flow, and resistance, forms the hemodynamic basis for FFR: As described in Chapter 23, the heart has the ability to maintain adequate coronary blood flow across a range of perfusion pressures because of the autoregulatory system. The resistance of the myocardial microcirculation is regulated to vary coronary blood flow based on the oxygen demand of the myocardium. FFR is measured when microvasculature resistance is at a minimum and coronary flow is at a maximum, which enables determination of maximal pressure loss across a stenosis in an epicardial coronary artery (Figure 24.2). FFR is defined as the maximum myocardial blood flow in the presence of a stenosis divided by the theoretical maximum myocardial blood flow in a normal coronary vessel (i.e., in the absence of a stenosis). Thus, it represents the fraction of normal coronary blood flow that is achievable in the presence of an epicardial coronary stenosis. This index is independent of heart rate, systemic blood pressure, or myocardial contractility. Figure 24.2 Measurement of FFR. Simultaneous measurement of pressure in aorta and distal LAD (labeled pressure wire) at rest (a) and following intracoronary administration of adenosine (b) in a 63‐year‐old male with chest pain and an intermediate lesion on angiography. Under basal conditions, there is a 7 mm Hg difference in mean pressures between the distal left anterior descending coronary artery (measured with the pressure wire) and the aorta (measured with the guide catheter) and the FFR was 0.94. Following administration of 60 µg of adenosine, distal perfusion pressure decreased, the difference in mean pressures increased, and the FFR decreased to 0.58. FFR can be represented in the following equation. The perfusion pressure across the myocardium is equal to the mean aortic pressure, Pa, minus the central venous pressure, Pv. When a coronary stenosis is present, this gradient is equal to the pressure distal to a stenosis, Pd, minus the central venous pressure, Pv. At maximal hyperemia, resistance of the myocardium is minimized, Rmin, and can thus be removed from the equation: Furthermore, the venous pressure is minimal compared to the arterial pressure and can be removed from the equation: Thus in practical terms, FFR is obtained by simultaneously measuring the mean aortic pressure (from the distal tip of a coronary catheter) and coronary pressure distal to a stenosis (from the pressure transducer of the guidewire distal to a lesion) at maximal hyperemia. It is important to remember that several assumptions have to be made to enable the use of pressure data as a surrogate for coronary flow. Various vasodilators can be used to induce maximal hyperemia, including adenosine, nitroglycerin, or paparavine. Adenosine, which is safe and effective at producing maximal hyperemia, is the most frequently used vasodilator in clinical practice. It can be administered either by intracoronary bolus or intravenously, with clinical studies requiring the IV route to ensure that a steady state of hyperemia is achieved. A description of some clinical scenarios (see also Figure 24.3) illustrates the relationship between FFR and coronary flow: Figure 24.3 Examples of FFR measurements in four patients with coronary disease of different hemodynamic severity. Simultaneous pressure recordings, following intracoronary administration of adenosine, from the aorta and distal coronary artery in four different patients showing normal FFR and mildly, moderately, and severely decreased FFR. Initial studies done to determine the appropriate threshold of FFR for identifying patients who would benefit from revascularization compared FFR to noninvasive stress testing. Pijls et al performed bicycle stress testing, thallium scintigraphy, and stress echocardiography in 45 consecutive patients with chest pain and moderate coronary stenosis. In the 21 patients with FFR <0.75, all had at least one stress test with a positive result. Using a cutoff of 0.75 for FFR produced a sensitivity of 88%, specificity of 100%, and accuracy of 93% in this population. Furthermore, after revascularization of patients with FFR <0.75, all of the positive FFR results reverted to normal [1]. Subsequent studies (most noticeably the FAME studies) modified the FFR threshold to 0.80, which is used in clinical practice today. There are many clinical scenarios in which measurement of FFR has been shown to be clinically useful. Some of the most important studies are summarized here: FFR is now standard of care for evaluation of intermediate angiographic lesions in stable ischemic disease. In a recent study, the supervising cardiologist was asked to determine a management strategy (PCI, medical therapy, CABG) after diagnostic angiography in 200 patients with stable chest pain [5]. Then FFR was performed on all vessels >2.25 mm and the supervising cardiologist was asked to determine a second management strategy, based on angiography and the results of FFR testing. The addition of FFR to angiography changed the management plan in 26% of the patients, and the number and location of lesions changed in 32%. To measure FFR of a left main lesion, intravenous adenosine should be used to induce maximal hyperemia. The guide should be disengaged to avoid ostial narrowing causing obstruction of blood flow with pressure damping in the aortic tracing and false elevation of the FFR value. The pressure sensor should be placed distal to the lesion. It has been suggested that FFR is not useful to assess left main lesions in the presence of severe disease in the left anterior descending or circumflex arteries [9], although other studies have simulated how downstream disease has a small but not clinically significant difference on the FFR value of the left main lesion, and thus FFR is still valid in that scenario [10]. There are several limitations that must be kept in mind when using FFR. There is the need for adenosine, which increases the time, cost, and risk of side effects. Moreover, technical aspects such as removing the introducer, clearing the guide of contrast, disengaging the guide for an ostial lesion, and ensuring absence of drift are important to ensure an accurate FFR value [17]. From a theoretical standpoint, several assumptions have to be made to simplify the equation from Lastly, recent studies suggest that FFR has a continuous and independent relationship with clinical outcomes rather than a defined threshold value [18]. The role of FFR in ischemic risk stratification continues to evolve. Instantaneous wave‐free ratio (iFR) is a recently developed measurement of the hemodynamic significance of a coronary artery stenosis that relies on comparison of pressures during diastole in the absence of hyperemia. It is measured during the wave‐free period of mid to late diastole, when flow during the cardiac cycle is the highest and the microcirculatory resistance is the lowest. During this period, pressure and flow velocity are linearly related, allowing pressure ratios to be used to determine the limitation to flow of a lesion. Unlike in FFR assessment, hyperemia (and thus adenosine) is not required. Using an FFR threshold of 0.80 as the gold standard, an iFR threshold of <0.90 has been proposed for revascularization based on the RESOLVE study [19]. The ADVISE studies took a different approach and proposed a hybrid iFR–FFR approach, which involves performing revascularization for iFR <0.86, deferral for iFR >0.93, and using FFR to guide revascularization if iFR is between 0.86 and 0.93. This approach allowed a 95% classification agreement between the hybrid strategy and FFR while sparing 60% of patients from adenosine [20]. Further outcome studies, including DEFINE‐FLAIR and iFR‐SWEDEHEART, are being performed to determine the safety of using iFR to guide revascularization.
Fractional flow reserve
Concept of fractional flow reserve
Key clinical studies of FFR
Limitations of FFR measurement
to
as detailed earlier, including that venous pressure and resistance are negligible. However, if either central venous pressure or resistance is elevated, the FFR value may be overestimated.
Instantaneous wave‐free ratio
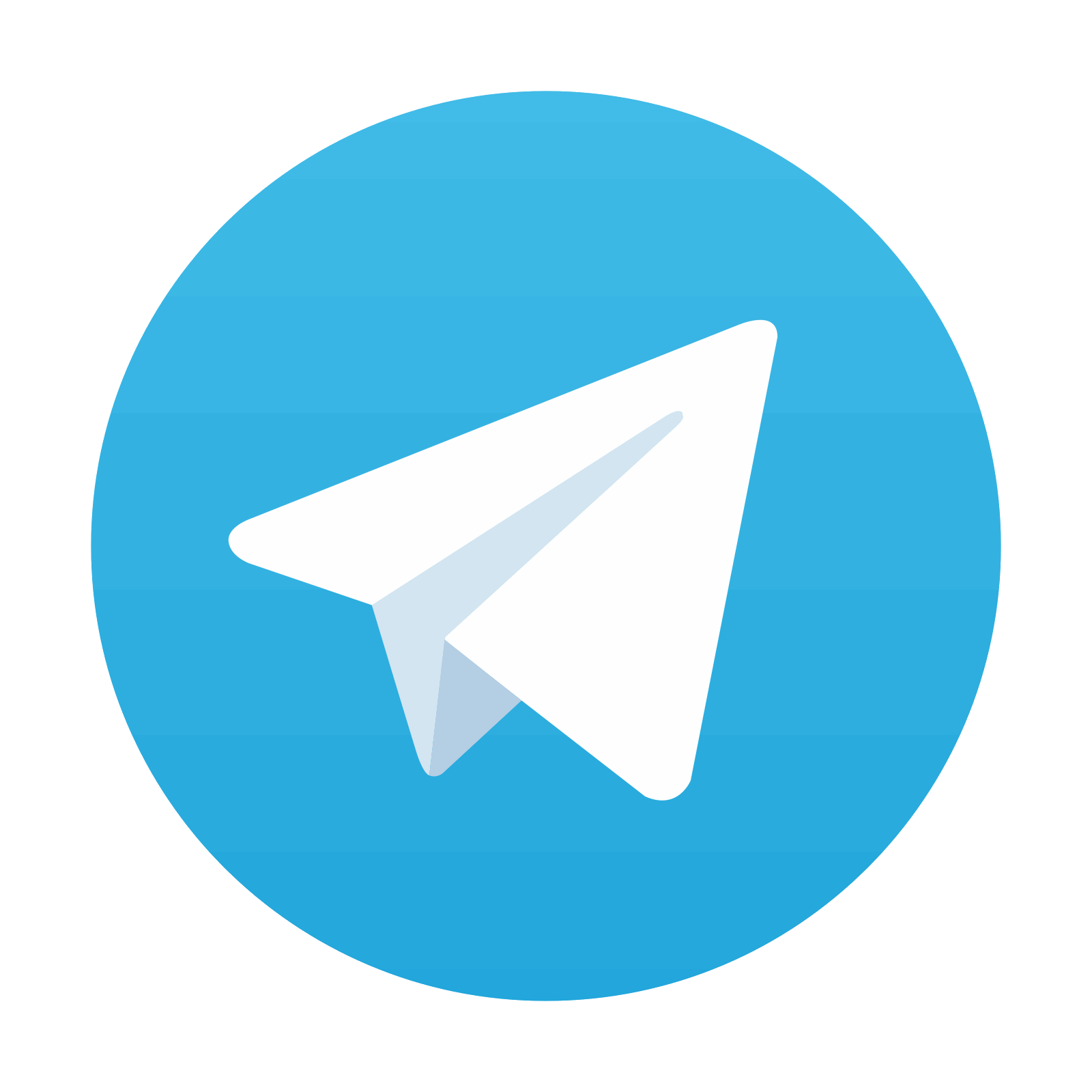
Stay updated, free articles. Join our Telegram channel

Full access? Get Clinical Tree
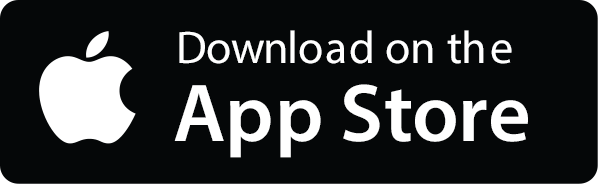
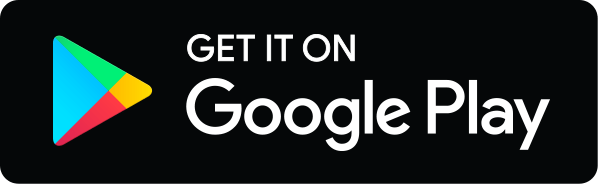