REGIONAL ANATOMY FOR THE INVASIVE ELECTROPHYSIOLOGIST
Introduction
The extraordinary advances over the last 2 decades with the invasive management of cardiac arrhythmia can be traced to the years of understanding the anatomy and electrophysiology (EP) of arrhythmogenic substrates.
The contemporary invasive electrophysiologist needs to be just as conversant with the knowledge of regional anatomy and arrhythmia correlation. This is necessary not only to understand the rationale for existing and future ablation approaches but also, important in maximizing the safety by avoiding inadvertent damage to surrounding structures.
In this chapter, we review the major clinically relevant arrhythmia (atrial fibrillation [AF], atrial flutter [AFL], and ventricular tachycardia [VT]) and sequentially discuss the major anatomic principles that guide present day ablation for these disorders. For each of the principle arrhythmia categories, a discussion of the regional anatomy for the sites that house the primary ablation targets followed by a brief discussion of the correlative value in detailed understanding of these structures to the invasive electrophysiologist follows.
Atrial Fibrillation
While the circuit of the typical AFL is anatomically well recognized, the substrate and triggers of AF are not fully understood. Circumferential ablation around the pulmonary veins (PVs) is an established management option for patients with symptomatic AF. However, other thoracic veins and the retroatrial structures are also involved in the pathogenesis and sometimes should be targeted to achieve a successful ablation. The importance of knowing the regional anatomy of the PVs is to accurately diagnose the origin of the electrograms (EGMs), which enables the electrophysiologist to perform the ablation with higher precision and safety. The other thoracic veins potentially involved in the AF mechanism are the superior vena cava (SVC) and the vein of Marshall (VOM). Both structures have complex anatomic relationships, and improving the knowledge of these boundaries will allow accurate mapping and completeness of ablation. In addition, the left atrium (LA) and thoracic veins are related to important and vital structures that should be recognized in order to avoid serious complications. In turn, the relevant anatomy of the PVs, SVC, VOM, and the LA are detailed.
Figure 2.1. Left panel: Left lateral view of an explanted heart showing a large left common pulmonary vein (LCPV). The posterior wall of the left atrial appendage (LAA) is in close relation with the anterior wall of this vein, which can generate contaminant signals when recording from the left-sided PV. Right panel: Posterior view of an explanted heart. The right superior pulmonary vein (RSPV) is related to the posterior wall of the right atrium (RA) and the left veins are close to the coronary sinus (CS) and LAA. As the right inferior pulmonary vein (RIPV) has no close anatomical relations, it is rare to record far-field potentials in this location. (Reproduced from Macedo et al4 with permission from John Wiley and Sons.).
The pulmonary veins
Gross anatomy and relations In most human hearts, there are 4 veins draining into the LA.1 A relatively frequent anatomic variation is the presence of an accessory middle right PV or a common trunk for the left PVs (Figure 2.1).2 In normal hearts, the mean distance between the left PVs is 8.4 mm and between the right PVs is 6.5 mm. The orifices of the right PVs are adjacent to the interatrial septum, while the left-sided PVs are just posterior to the LAA.1 The VOM lies in between the LAA ostium and the left PVs, an area also rich in autonomic nerve fibers.3
The RSPV courses immediately posterior to the junction of the SVC with the RA. The azygous vein and a branch of the right pulmonary artery (PA) are located superior and posterior to the right upper PV. The RIPV is the only area where a branch of the right PA courses anteriorly to the vein, which separates it from the myocardial structures.4
Importance of the PV ostium and myocardial sleeves For AF ablation, the PV ostium has a crucial role. The heterogeneous histology in and around the vein ostium is mainly responsible for AF arrhythmogenesis, and why it is the principal target for ablation. The anatomical recognition of the PV ostium is also important to avoid radiofrequency (RF) applications deep within the veins, which can lead to PV stenosis. However, with all the imaging tools available, it is still difficult to determine their exact location. Even in macroscopic histological examinations, there are no landmarks demarcating the transition from vein to atrium. Microscopically, syncytial atrial myocardium extends into the veins in a way to form a sphincter-like structure with the function of preventing venous regurgitation.5 Close to the ostium, the myocardium is thicker and ablation in this site leads to thinning of the myocardial layers and aneurismal dilation. Deep in the vein, however, the myocardium is very thin or absent and RF applications cause endothelial proliferation and luminal narrowing. In turn, the electrophysiologist should target the myocardium proximal to the ostium to avoid PV stenosis.4 The use of preprocedural computed tomography (CT) scans or magnetic resonance imaging can help in determining the approximate location where the PVs join to the LA. During ablation, an intracardiac ultrasound, rotational angiography, retrograde pulmonary venography, or electroanatomic mapping systems can also be used.6
Myocardial sleeves are extensions of atrial muscle fibers into the PVs. The longest sleeves are usually seen in the superior PVs (10 mm on average comparing to 5 mm in the inferior PVs).1,5 Although the fibers are most circularly oriented in the transmural extension, there are also fascicles of longitudinal and oblique fibers that interdigitate between the circular bundles. This change in orientation can produce nonuniform anisotropy, which can then function as a substrate for micro-reentry or automatic foci.7
Recently, node-like cells were described in the PVs of patients with AF. They were called P cells (pale cells) and had characteristics of automaticity.8 Also, Cajal cells and PAS-positive cells were encountered in the PVs, especially in AF patients.9,10 Although it looks like it is a promising mechanistic explanation, there is no sufficient data to affirm that these cells trigger AF.
The superior vena cava
The presence of myocardial sleeves in the SVC has been related to non-PV triggers of AF. In addition, automatic atrial tachycardias (ATs) and reentrant circuits (upper loop reentry) were also described in this site.11–13 Knowledge of the anatomic relationships allows the ablationist to better interpret the EGMs, avoid important surrounding structures, and perform a successful and safe procedure.
Gross anatomy and relations The SVC is formed by the confluence of the right and left brachiocephalic veins, which lie just below the junction of the right first rib to the sternum.14 Its origin landmark in imaging studies is the right tracheobronchial angle, which is always caudal 0.1 to 3.8 cm.15 From the origin to the junction with the RA, the SVC measures 6 to 8 cm and courses with a slight right convexity.4 The azygous vein enters into the posteromedial region of the SVC at variable distances from the SVC/RA junction. The lower part of the SVC is involved by the pericardium.
Anteriorly to the SVC are the anterior margins of the right lung and pleural, and posteriorly is also the right lung, the vagus nerve, and more inferiorly the RSPV. Laterally, there is the right phrenic nerve, which courses posteroinferiorly to the vein in the direction of the RA, in between the RSPV and the SVC.16 On the left lies the origin of the brachiocephalic artery and the ascending aorta (AO). Among the SVC, the AO, and the trachea, there is a reflection of pericardium called the aortocaval reflex. The floor of this reflex is formed by the right PA.14
In contrast to the inferior vena cava (IVC) wall, the SVC is endowed with muscular extensions from the RA, similar to what occurs within the CS and the PV.4,17 The muscle fibers are more circularly oriented at the ostium, which can be responsible for a delayed conduction into the vein. This region also has a rich parasympathetic plexus (see below).
The sinus node is an epicardial structure located posterolaterally at the junction of the SVC to the RA in the region of the sulcus terminalis. The nodal cells can be fanned out, occasionally reaching the adjacent veins.14
Anatomy-electrophysiology correlations The SVC-related mechanism of AF triggering and sustaining is not completely understood. It was already demonstrated that the muscular sleeves in this vein can trigger cardiac activation and be the source of AF and other atrial arrhythmias.11–13,18 The SVC musculature may also be responsible for sustaining AF, as rapid firing activity has been described.19 Both phase IV depolarization-initiated automatic activity and abnormal automaticity have been recorded inside this vein, which could yield AF.20 Occasionally, the muscular sleeves in the SVC may connect not only to the RA but also to the RSPV, making up a potential connection to the LA.
Because of the draining azygous vein, the adjacent RSPV, and the RA itself, the EGM in this region can display contaminant signals. However, pacing from the RA and then from the SVC can differentiate the PV signal and allow safe ablation in the SVC/RA junction or within the RA.4
Phrenic nerve injury is possible not only with RSPV ablation but also while targeting SVC isolation. The nerve may be spared with continuous pacing from the distal mapping/ablation electrode at 5 V output while performing cryoablation.4,21
Furthermore, extensive ablation at the RA close to the SVC junction may damage parts of the sinus node. If venous isolation requires many RF applications at the RA, close monitoring of the sinus rhythm should be done watching for any decrease in automaticity.4
In the aortocaval reflection described above, there is an important structure of the autonomic innervation of the heart: the aortocaval ganglion. As it comprises the autonomic tissue of the surrounding AO, right PVs, and PAs, ablation at this site can alter atrial repolarization and conduction times. Thus, ablating these ganglia can modulate atrial arrhythmias beyond SVC musculature isolation.22,23
The vein of Marshall
The VOM is a remnant of the left SVC, and it is located between the ostium of the LAA and the left-sided PVs. It is of great importance to the electrophysiologist because it can be the source of AF triggers or a bridge from the PVs to the LA after conventional PV isolation. In addition, it can serve as a vantage point to access and ablate the retroatrial ganglionated plexuses in this location.4
The atrial myocardium drains into the CS through various veins, VOM being the most consistent. It runs obliquely in the posterior wall of the LA to join the great cardiac vein and the main posterior lateral vein to form the CS (Figure 2.2).24 Similar to the SVC, it is possible to encounter nodal tissue and autonomic innervation in the VOM. Atrial muscle fibers are found in the CS and at the ostium of the draining atrial (VOM) and ventricular (V) veins.4
Figure 2.2. Coronary venous system. Note the course of the VOM obliquely in the posterior wall of the LA. It joins the great cardiac vein and the left posterolateral vein to form the CS. (Reproduced from Lachman et al95 with permission from John Wiley and Sons.)
Anatomy-electrophysiology correlation The VOM can be a trigger site for initiating AF. When re-initiation of AF occurs after PV and possibly SVC isolation, origin in the VOM should be considered. Ablation approaches include energy delivery within the vein, isolation at the ostium of this vein at its junction with the CS, endocardial transmural ablation, or epicardial ablation via subxiphoid access can be done.4,25,26 The VOM may possibly also act as a bridge electrically connecting the CS, LA, and epicardial surface of the PV.
The VOM can also be involved in a macro-reentrant LA flutter circuit or inter-AFL circuit. It can be suspect when there is still electrical conduction after a surgical maze procedure or after linear ablation between the left inferior PV and the mitral annulus. In such cases, electrical conduction may occur from the lateral wall of the LA to the CS via the VOM and then back to the medial LA wall or to the RA completing the circuit. Ablation of either the VOM or the CS can terminate these macro-reentrant circuits.4
Ablation of the vein VOM can be performed either directly via vein cannulation or through the atrial endocardium.27 As the endocardial ridge separating the LAA and the left PVs is a recognizable internal mark of the VOM, RF applications at this point can successfully ablate this vein without the need of cannulating the vein.4
Occasionally, it is necessary to ablate the retroatrial ganglia close to the VOM. Here, this vein can allow easy access to the epicardial ganglia, which may be targeted for ablation or modulation.
The left atrium
Knowledge of the LA regional anatomy is essential for avoiding collateral damage, as this is the most posterior chamber of the heart and is in close proximity to important structures.
The LA is posterior and superior to the RA. Posteriorly, the LA is related to the tracheal bifurcation and to the esophagus, from which it is separated only by the fibrous pericardium.28 Anteriorly, this chamber accommodates the aortic root and posteroinferiorly it is connected to the CS. The LA can be divided in 4 regions: the septum, the vestibule, the appendage, and the venous component.1 The septum is recognizable by the flap valve of the foramen ovale. The vestibule is the region around the mitral annulus. The LAA is small and tubular, and is the only component of the LA where pectinate muscles can be found. The venous component is the largest part of the LA and receives the PVs.
Anatomy-electrophysiology correlation The atrial myocardium thickness varies considerably from region to region in the LA. Importantly, the atrial roof may be so thin that can be transilluminated. Therefore, catheter manipulation must be done carefully in this area in order to avoid cardiac perforation. In addition, whenever ablating in the atrial roof, RF application should only be performed if a contact near-field EGM is displayed and should be avoided where EGMs are absent or no longer present.28
In contrast, the thickness of atrial myocardium can make ablation challenging in different regions, such as the mitral isthmus, the Bachmann’s bundle, and the endocardial ridge between the LAA ostium and the left-sided PVs.4
The mitral isthmus consists of the atrial myocardium separating the mitral valve and the left PVs, and it can participate in atypical AFL circuits.7 Linear ablation across this isthmus in the level of the left inferior PV is the typical approach. This ablation is best done after ostial isolation of the PV that serves as an anchoring point.
The primary site of interatrial conduction is the Bachmann’s bundle, which is located in the anterior portion of the LA roof. It is composed of traversing fibers from the right atrial appendage (RAA) to the LAA.29 Ablation is this site is currently used to terminate inter-AFLs occurring after surgical maze procedure. In such circuits, the Bachmann’s bundle connects both atria, and the return limb is through the CS muscular sleeves.28
Important anatomic relations to consider in order to avoid the collateral damage
With the increasing number of AF ablations, collateral damage has also become more frequent. Since the LA and the thoracic veins are adjacent to important cardiac and noncardiac structures, knowing the regional anatomy is essential for avoiding inadvertent injury of surrounding tissue. The occurrence and methods of preventing PV stenosis has been described above; therefore, in this section the esophageal trauma and coronary artery (CA) damage will be detailed.
Esophageal injury The esophagus lies immediately behind the posterior wall of the LA, separating only the oblique sinus and a variable amount of connective tissue. The oblique sinus is a pericardial reflection and has been used to monitor and prevent esophageal trauma during ablation.28
The occurrence of atrial-esophageal fistula is a rare but catastrophic collateral damage of ablation in the posterior wall of the LA.30,31 Since this region has a thin and sometimes absent myocardial layer, careful RF application should be attempted. Also to avoid this complication, monitoring the luminal esophageal temperature is used. However, thermal injury may occur even before temperature rise on the luminal probe.32,33 Therefore, also direct visualization of the esophagus through intracardiac ultrasound is used to allow early detection of any echo density formation or effusion. Nevertheless, so far there is no endpoint for temperature increase, duration of energy delivery, or power setting as a recommendation for different atrial regions.
In addition, the placement of a balloon in the oblique sinus has been attempted to move the esophagus away from the LA posterior wall.33 Also, mechanical deflection of the esophagus using an echocardiography probe has been tried in few patients with successful results.34
Coronary artery damage CA occlusion can occur not only during AF ablation but also while ablating epicardial accessory pathways (APs) and outflow tract VTs.35,36 Nonetheless, this section will address the anatomic relations important to CA collateral damage when ablation of AF is attempted.
While targeting the mitral isthmus, a potential injury to the circumflex CA may occur (Figure 2.3). This artery is typically located more V than the CS in this region.24,37 Therefore, if higher energy delivery is necessary, either from the endocardium or the epicardium, one should avoid the V portion of the mitral annulus.
Figure 2.3. Potential sites for coronary damage during AF ablation. Damage to the circumflex artery may occur while attempting ablation between the LIPV and the mitral valve or while targeting the CS near the VOM. Ablation in the proximal CS may damage a distal branch of the right coronary artery (RCA). Following PV isolation, one may encounter a tachycardia focus arising from the septal mitral annulus. Ablation in this site may lesion the proximal circumflex and left anterior descending arteries (LAD). (Reproduced from Macedo et al28 with permission from John Wiley and Sons.)
Following isolation of the PVs, automatic ATs arising from either the aortic cusp and/or the mitral annulus can occur.38,39 In this region, the distal left main CA (LMCA) and proximal circumflex CA are within 5 to 10 mm from the aortic-mitral continuity. Judicious energy delivery should be done just sufficient to terminate tachycardia and arrhythmogenic atrial substrate.
Occasionally, the myocardium of the CS is targeted for ablation. This includes ablation of the VOM or of an automatic foci in the CS itself or the interruption of a macro-reentrant inter-AFL (either connections with the LA or with the RA).4 A posterolateral branch of the RCA can be injured while ablation of the proximal CS is attempted. Otherwise, the main circumflex CA can be damaged when ablating deeper in the CS, especially if closer to the V portion of the annulus.28
Atrial Flutter
The AFL was identified as an intra-atrial circuit around the vena cava in 1921 by Sir Thomas Lewis et al.40 Forty years ago, Puech et al discriminated the typical and rare types of AFL and described that the typical AFL was the result of an activation proceeding cranially along the interatrial septum and caudally along the free wall of the RA in a large reentrant circuit (RA).41 In 1990, an area of slow conduction in the posteroinferior region of the RA was associated with the presence of AFL.42 The so-called cavo-tricuspid isthmus (CTI) was later characterized as the atrial myocardium between the hinge point of the tricuspid valve (TCV) and the IVC and was recognized as the critical isthmus for typical AFL.43,44 The RF delivery across the CTI with the creation of a bidirectional conduction block is currently the endpoint criteria for typical AFL ablation and has been correlated with high rates of success in terminating the arrhythmia and preventing recurrences.44,45 In this section, the normal CTI and its boundaries, as well as anatomic variations, will be described and correlated with the difficulties possibly encountered during AFL ablation.
Normal cavo-tricuspid isthmus
The CTI is an isthmus bounded anteriorly by the tricuspid annulus (TA) and posteroinferiorly by the transition of the RA to the IVC (Figure 2.4). The myocardial fibers in this region are usually oriented parallel to the TCV, but can occasionally be septally arranged, in which case they merge with the CS.46 The CTI is broader and thicker in its lateral portion and is thinner in the center; however, the thickness in the inferolateral portion is highly influenced by the presence and size of traversing pectinate muscles.47,48 In an anatomic study of 602 human hearts, it was found that the mean length of the CTI was 17.7 mm and that the pectinate muscles extended into the CTI in 70% of the hearts.49 In such cases, they tend to be more laterally located. In turn, the atrial myocardium close to the TA is usually smooth, and this part of the CTI is called vestibular portion.
Figure 2.4.
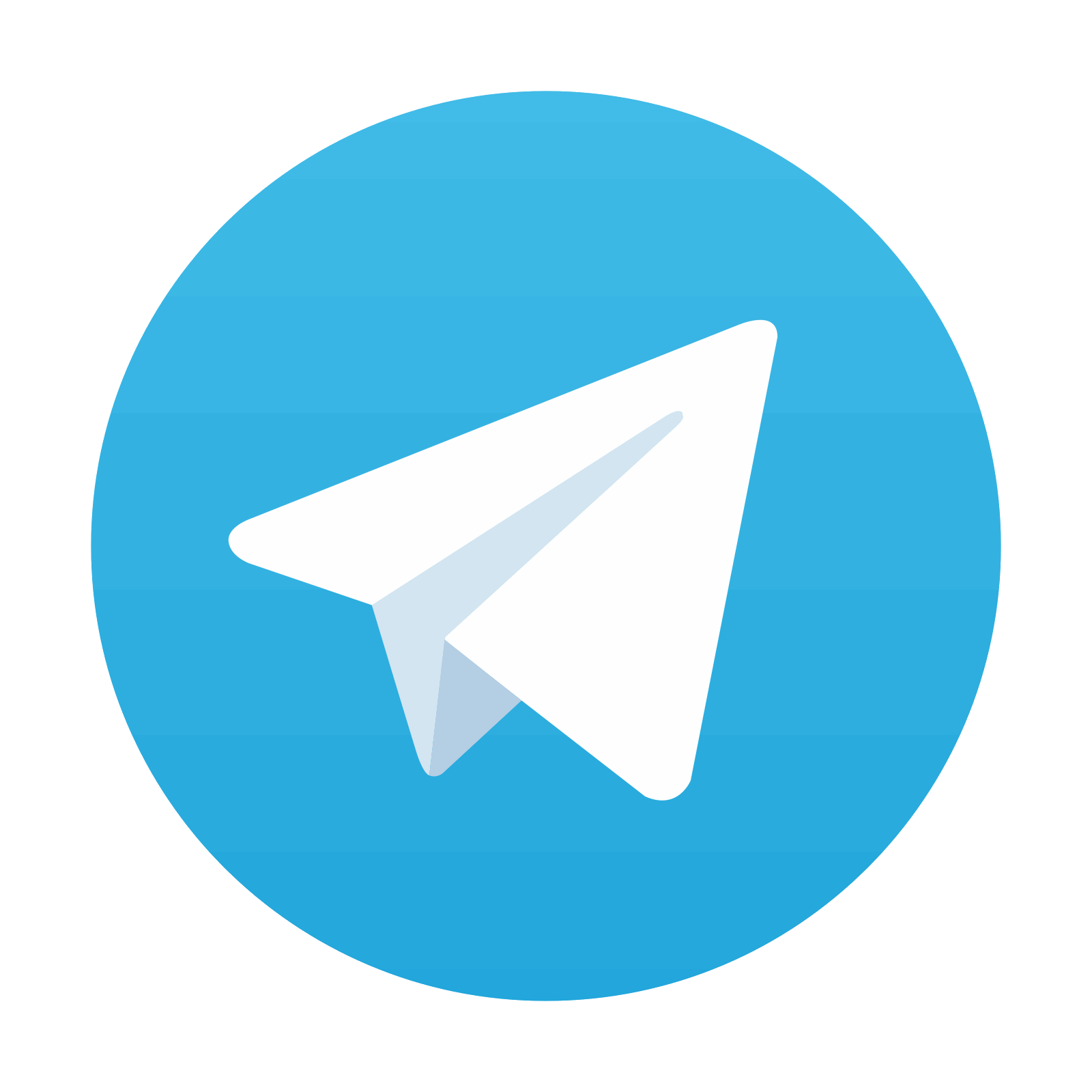
Stay updated, free articles. Join our Telegram channel

Full access? Get Clinical Tree
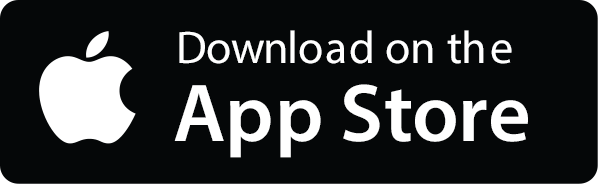
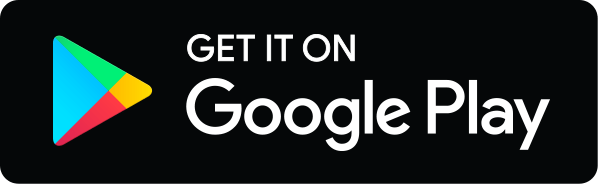