Atherothrombosis is a systemic arterial disease of large- and medium-sized arteries including the coronary, carotid, aorta, and peripheral arteries. The clinical manifestations depend on the size of the vessels and the regional circulation involved and include coronary artery disease, stroke, and peripheral vascular disease. A paradigm shift is occurring with a change in focus from the assessment and treatment of luminal narrowing toward greater understanding of the vascular biology in the arterial wall that leads to plaque vulnerability. Recent research has shown that inflammation plays a key role in the pathogenesis and progression of atherothrombosis. The basic concepts of evolution of atherothrombosis, and the role of inflammation leading to vulnerable plaque, will be discussed here.
The main components of atherothrombotic plaques are1,2,3,4,5,6,7 (1) connective tissue extracellular matrix, including collagen, proteoglycans, and fibronectin elastic fibers; (2) crystalline cholesterol, cholesteryl esters, and phospholipids; (3) cellular components such as macrophages, T lymphocytes, and smooth muscle cells; and (4) thrombotic material with platelets and fibrin deposition. The proportion of these components varies in different plaques, and explains the heterogeneity of lesions and potential vulnerability to plaque rupture.
According to a simplified modification of the criteria set forth by the American Heart Association Committee on Vascular Lesions,3 plaque progression can be classified into five phases as shown in Figure 4-1.
Phase 1: (early): These lesions are small and commonly seen in individuals younger than 30 years. Based on their composition, these plaques are categorized into three types: type I lesions, consisting of macrophage-derived foam cells with intracellular lipid droplets; type II lesions, consisting of macrophages, smooth muscle cells, and extracellular lipid deposits; and type III lesions, consisting of smooth muscle cells surrounded by extracellular connective tissue, fibrils, and lipid deposits. It is possible for these early lesions to regress to normal.8
Phase 2: (advanced): These lesions, although not necessarily stenotic, may be prone to disruption because of their high lipid content, thin fibrous caps, and increased inflammation. They are categorized into two types: type IV lesions, which consist of confluent cellular lesions with a great deal of extracellular lipid intermixed with fibrous tissue, and type Va lesions, which possess an extracellular lipid core covered by a thin fibrous cap. Phase 2 plaques can evolve into the acute phases 3 and 4, and either of these can evolve into phase 5 plaques.
Phase 3: This phase consists of acute complicated type VI lesions, originating from disrupted type IV or Va lesions, and leading to mural, nonobstructive thrombosis. Although clinically silent, the process may occasionally lead to the onset of angina.9
Phase 4: This phase includes acute complicated type VI lesions, with fixed or repetitive occlusive thrombosis. This process may be silent,10,11 but usually leads to an acute coronary syndrome (ACS).
Phase 5: This phase includes type Vb (calcific) or Vc (fibrotic) lesions that may cause angina; however, if preceded by stenosis or occlusion with associated ischemia, the myocardium may be protected by collateral circulation, and such lesions may then be silent or clinically inapparent.12,13
FIGURE 4-1.
Modified AHA classification of lesion morphology of coronary atherosclerosis according to gross pathological and clinical findings.
Reproduced with permission from Springer Science and Business Media. Corti R et al. Evolving concepts in the triad of atherosclerosis, inflammation and thrombosis. J Thromb Thrombolysis. 2004;17:35-44.

Under basal conditions, the endothelium functions to maintain the vessel in a relatively dilated state. In response to various physical stimuli, such as shear stress, the blood vessels dilate by an endothelial dependent process called flow-mediated dilation. This response is principally regulated by release of nitric oxide (NO) from the endothelium. NO is synthesized from amino acid l-arginine by endothelial nitric oxide synthase (eNOS). By virtue of NO, the endothelium regulates anti-inflammatory, mitogenic, and contractile activities of the vessel wall as well as the hemostatic process within the vessel lumen14 (Figure 4-2).
FIGURE 4-2.
Healthy endothelium under laminar flow conditions and no risk factors. A single molecule, nitric oxide (NO), is involved in multifactorial pathways preventing monocyte adhesion, platelet aggregation, and smooth muscle cell proliferation. PGI2, prostacyclin 2; SMC, smooth muscle cell; tPA, tissue plasminogen activator.
Reproduced with permission from Elsevier Science. Fuster V et al. Atherothrombosis and high-risk plaque. J Am Coll Cardiol. 2005;46:937-954.

Endothelial dysfunction has been traditionally described as the earliest manifestation of atherosclerosis. It is often the result of a disturbance in the physiologic pattern of blood flow at bending points and near bifurcations.15,16 In addition, it is associated with biohumoral risk factors such as hypercholesterolemia, diabetes, hypertension, obesity, smoking, and advanced age17,18,19 (Figure 4-3). A dysfunctional endothelium, characterized by decreased NO synthesis, facilitates vessel wall entry and oxidation of circulating lipoproteins, monocyte entry, smooth cell proliferation and extracellular matrix deposition, vasoconstriction, as well as a prothrombotic state within the vessel lumen.20,21
FIGURE 4-3.
Diseased endothelium with nonlaminar flow, low-density lipoprotein (LDL) deposition, cell adhesion molecule (CAM) expression, macrophage migration, tissue factor (TF), and matrix metalloproteinase (MMP) expression leading to smooth muscle cell (SMC) proliferation and vasa vasorum neovascularization. PDGF, platelet-derived growth factor; PAI-1, plasminogen activator inhibitor-1; TXA2, thromboxane A2.
Reproduced with permission from Elsevier Science. Fuster V et al. Atherothrombosis and high-risk plaque. J Am Coll Cardiol. 2005;46:937-954.

Low-density lipoproteins (LDLs) infiltrate through the arterial endothelium into the intima.8 The binding of LDL to proteoglycan in the intima leads to the retention of these particles. The proteoglycan bound LDL particles have an increased susceptibility to oxidative and other chemical modifications, making them proinflammatory, chemotoxic, cytotoxic, and proatherogenic. The mechanisms responsible for the atherogenic modification of these LDL particles are not known, but may involve oxidation mediated by myeloperoxidase, 15-lipoxygenase, and nitric oxide synthase (NOS).22
Normal endothelium generally resists leukocyte adhesion. However, in response to atherogenic and proinflammatory stimuli, particularly oxidized LDL particles in the intima, the endothelial cells are activated. Activated endothelial cells express adhesion molecules, primarily vascular cell adhesion molecules-1 on their surface. These molecules regulate the interaction of monocytes and T cells with the endothelium. Besides vascular cell adhesion molecules-1, other adhesion molecules, such as intercellular adhesion molecule-1, E selectin, and P selectin, likely contribute to recruitment of leukocytes to the atherosclerotic lesion.23,24
Once adherent to the endothelium, migration of leukocytes into the arterial wall involves the action of protein molecules known as chemotactic cytokines, or chemokines. Experimental studies indicate that the most important chemoattractants are oxidized LDL and monocyte chemotactic protein-1 (MCP-1). MCP-1 is a powerful chemokine and its receptor CCR2 on the monocyte/macrophages may be significantly upregulated during plaque progression. It attracts both monocytes and T cells, but not neutrophils and B cells, and likely plays a key role in the intimal recruitment of these cells. Endothelial cells, smooth muscle cells (SMCs), and macrophages all contribute to the overexpression of MCP-1 in atherosclerosis. Thus, monocyte-derived macrophages in the intima may recruit more monocytes into the intima by secreting MCP-1. Cytokines, such as interleukin-8, may also play a role in leukocyte migration.22
Once within the intima, the monocytes differentiate into macrophages under the influence of macrophage colony-stimulating factor (M-CSF), produced by endothelial and SMCs. These macrophages internalize the oxidized LDL particles via the scavenger receptors, of which SR-A and CD36 have been shown to play a significant role in experimental atherosclerosis.25 The development of lipid-laden macrophages, the foam cells, is the hallmark of both early and late atherosclerotic lesions. Aside from their scavenger function, macrophages also express matrix degrading proteolytic enzymes, matrix metalloproteinases (MMPs), and tissue factor (TF), which contribute to their significant destabilizing and thrombogenic properties.
Once macrophages have taken up residence in the intima and become foam cells, they not infrequently replicate. The factors that trigger macrophage cell division in the atherosclerotic plaque likely include M-CSF, interleukin-3, and granulocyte-macrophage colony stimulating factor.
In the development of early and asymptomatic foam cell lesion, the fatty streak, the cells responsible are mostly endothelial cells, macrophages, and a few T cells. With plaque progression, the fibroproliferative response mediated by the SMCs plays a key role in the evolution of atheroma into more complex plaques. The SMCs are the principal connective tissue producing cells in the normal and atherosclerotic intima.26 Some SMCs likely arrive in the arterial intima early in life, while others migrate into the intima from the underlying media. The chemoattractants for SMCs include molecules such as platelet-derived growth factor, secreted by activated macrophages and overexpressed in human atherosclerosis. These intimal SMCs are capable of multiplying by cell division.
While the repairing and protective capabilities of SMCs are considered beneficial, the impaired function or death of these cells is likely detrimental, suggested by the local loss of SMCs at the rupture site of plaques. The exact mechanism for SMC loss at rupture sites is not known, but apoptotic cell death may play a role.27,28 Apoptosis can occur in response to inflammatory cytokines in the evolving atheroma or by elimination of some SMCs by the T cells.
Thus, SMC accumulation in the growing atherosclerotic plaque results from a balance between cell replication and cell death. In addition to SMCs, the endothelial and monocyte/macrophage foam cells are also subject to cell death by apoptosis, and this process contributes significantly to increased TF activity and thrombogenicity of the lipid-rich core.29
Over the past few years, basic and clinical research has demonstrated a fundamental role for inflammation in atherogenesis.30,31 The macrophage foam cells not only serve as a reservoir for excess lipid but also provide a rich source of proinflammatory mediators, such as cytokines and chemokines and various eicosanoids and lipids such as platelet-activating factor. These phagocytic cells can elaborate significant amounts of oxidant species such as superoxide anion within the atherosclerotic plaque.32 The inflammatory mediators promote inflammation in the plaque and thus contribute to the progression of lesions. This amplification of the inflammatory response, which is not dependent on antigenic stimulation, is referred to as innate immunity. The important receptors for innate immunity in atherothrombosis are the scavenger receptors and the toll-like receptors (TLRs).33
As described earlier, the scavenger receptors SR-A and CD-36 mediate the uptake of oxidized LDL and transform the macrophage into a foam cell.34,35 Macrophage foam cells produce cytokines that promote monocyte migration, macrophage foam cell formation, and activation of neighboring smooth muscle cells.7 Furthermore, this pathway activates the proinflammatory NF-κ-B nuclear transcriptional factor, which triggers a potent chemoattractant response involving MCP-1, leukotriene LTB4, and M-CSF.34,35,36 This leads to further monocyte migration and macrophage/foam cell formation.
The role of cytokine-mediated inflammation and the resulting plaque instability are being increasingly recognized. The cytokine production is initiated by signaling through TLRs that recognize host-derived molecules released from injured tissue and cells. TLRs also activate the NF-κ-B pathway, resulting in production of cytokines that augment local inflammation.37 TLR1, TLR2, and TLR4 are shown to be upregulated in the endothelium and in areas infiltrated with inflammatory cells. In addition, adventitial fibroblasts and dendritic cells express TLR4 receptors and are able to produce a variety of cytokines after TLR4 activation.38
In addition to innate immunity, evidence also supports a prominent role for adaptive or antigen-specific immunity in plaque progression.30,31 In addition to the mononuclear phagocytes, dendritic cells in atherosclerotic lesion can present antigens to the T cells, including modified lipoproteins, heat shock proteins, beta-2 glycoprotein 1b, and infectious agents. The antigen-presenting cells (macrophages, dendritic cells, or endothelial cells) allow the antigen to interact with T cells, leading to their activation and subsequent release of large quantities of cytokines that modulate atherogenesis.
Extracellular matrix constitutes much of the volume of an advanced atherosclerotic plaque. The major extracellular matrix components of atheroma include interstitial collagens (types I and III), proteoglycans, and elastin,39,40 produced primarily by SMCs. Stimuli for excessive collagen production by SMCs include platelet-derived growth factor and transforming growth factor-beta (TGF-β)—a constituent of platelet granules and a product of many cell types found in lesions.
The biosynthesis of the extracellular matrix molecules is balanced by breakdown catalyzed in part by catabolic enzymes known as MMPs. Dissolution of extracellular matrix macromolecules undoubtedly plays a role in the migration of SMCs as they penetrate into the intima from the media through a dense extracellular matrix, traversing the elastin-rich internal elastic lamina. Extracellular matrix dissolution also likely plays a role in the arterial remodeling that accompanies lesion growth.
Focal calcification in atherosclerotic plaques is common and increases with age.41 Plaque calcification is considered an active process and resembles calcification in bone. The total amount of calcification, the coronary artery calcium score, is a marker of coronary plaque burden and provides prognostic information beyond that provided by traditional risk factors.42 Coronary calcification is composed of both hydroxyapatite and organic matrix, including type I collagen and noncollagenous bone-associated proteins.43 The most relevant noncollagenous bone-associated proteins associated with vascular calcification include osteopontin, osteonectin, osteoprotegerin, and matrix Gla protein. The most studied noncollagenous bone-associated protein in atherothrombosis is osteopontin, which is highly expressed by macrophages in the intima of human arteries.44 Clinical observations suggest that culprit lesions responsible for ACSs are generally less calcified than plaques responsible for stable angina, and the pattern of plaque calcification may be different in these two patient subsets.45,46
Continuous exposure to the systemic, proatherogenic milieu will increase chemotaxis of monocytes thus leading to lipid accumulation, development of necrotic core, and fibrous cap formation, evolving into advanced atherosclerosis. More recently, new structural and functional features of these lesions have been described, including vascular remodeling and vasa vasorum neovascularization.
Described by Glagov et al. in 1987,47 vascular remodeling involves eccentric and outward plaque growth with compensatory enlargement of the vessel wall (Figure 4-4). Luminal stenosis tends to occur only after the plaque burden exceeds some 40% of the cross-sectional area of the artery. This so-called positive remodeling or “compensatory enlargement” must involve turnover of extracellular matrix molecules to accommodate the circumferential growth of the artery. Several studies have shown increased macrophage-derived matrix metalloproteinases MMP-2 and -9 expression within the intima–media interface of remodeled plaques.48 The increased activity of MMPs digests the internal elastic lamina, modulating the process of remodeling.
FIGURE 4-4.
Vascular remodeling. Early plaque accumulation in human coronary arteries is associated with compensatory enlargement of the vessel size (positive remodeling) to accommodate the growing plaque. Hence, lumen size is either unaffected or minimally reduced. As atherosclerosis becomes severe, enlargement is overcome by plaque progression (left to right) and luminal narrowing is no longer prevented.
Adapted from Glagov S et al. Compensatory enlargement of human atherosclerotic coronary arteries. N Engl J Med. 1987;316:1371-1375.

Plaques susceptible to rupture and those responsible for ACSs are more likely to exhibit expansive remodeling, while those that more likely lead to luminal narrowing and resulting stable angina exhibit “negative” or “constrictive remodeling.”49
Angiogenesis is common in advanced atherosclerosis and is associated closely with plaque progression.50,51,52,53 Endothelial proliferation is predominantly thought to arise from the adventitia, where there is abundance of preexisting vasa vasorum. A breech in the medial wall likely facilitates the rapid in-growth of microvessels from the adventitia, and exposure to an inflammatory environment stimulates the development of immature endothelial tubes with leaky linings. This network of incompetent blood vessels is a viable source of intraplaque hemorrhage that may promote transition from a stable to an unstable lesion.
Angiogenesis depends on the interplay of various cytokines and growth factors released by the infiltrating inflammatory cells, including macrophages and T cells. Macrophages, attracted by oxidized LDL, are responsible for cytokine production, leading to neo-vessel growth.54,55 In addition, varying degrees of T lymphocytes are present in areas of neovascularization, specifically in the deep intima around the base and shoulder region of the necrotic core. Activated T cells are a known source of angiogenic factors, including vascular endothelial growth factor, and can stimulate angiogenesis in association with early lymphocyte recruitment.56
Two mechanisms can trigger plaque rupture. The first is related to physical forces and occurs most frequently where the fibrous cap is weakest. This is most often the shoulder region between the plaque and the adjacent vessel wall,57 where the cap is thinnest and is heavily infiltrated by foam cells with relative loss of SMCs.
The second mechanism involves an active process within the plaque leading to rupture. The macrophages and mast cells are capable of degrading extracellular matrix by phagocytosis or secretion of proteolytic enzymes. Thus enzymes such as plasminogen activators and MMPs, including collagenases, elastases, gelatinases, and stromelysins, degrade components of the extracellular matrix, may weaken the fibrous cap, and predispose it to rupture.58,59
Rupture of a high-risk vulnerable plaque may result in mural thrombus without evident clinical symptoms, or acute occlusion or subocclusion with clinical manifestations of unstable angina or ACS.60,61 A number of factors, including plaque-derived thrombogenic substrate, rheology, and systemic procoagulant activity, may influence the stability and magnitude of the resulting thrombus and, thus, the severity of the coronary syndrome.62
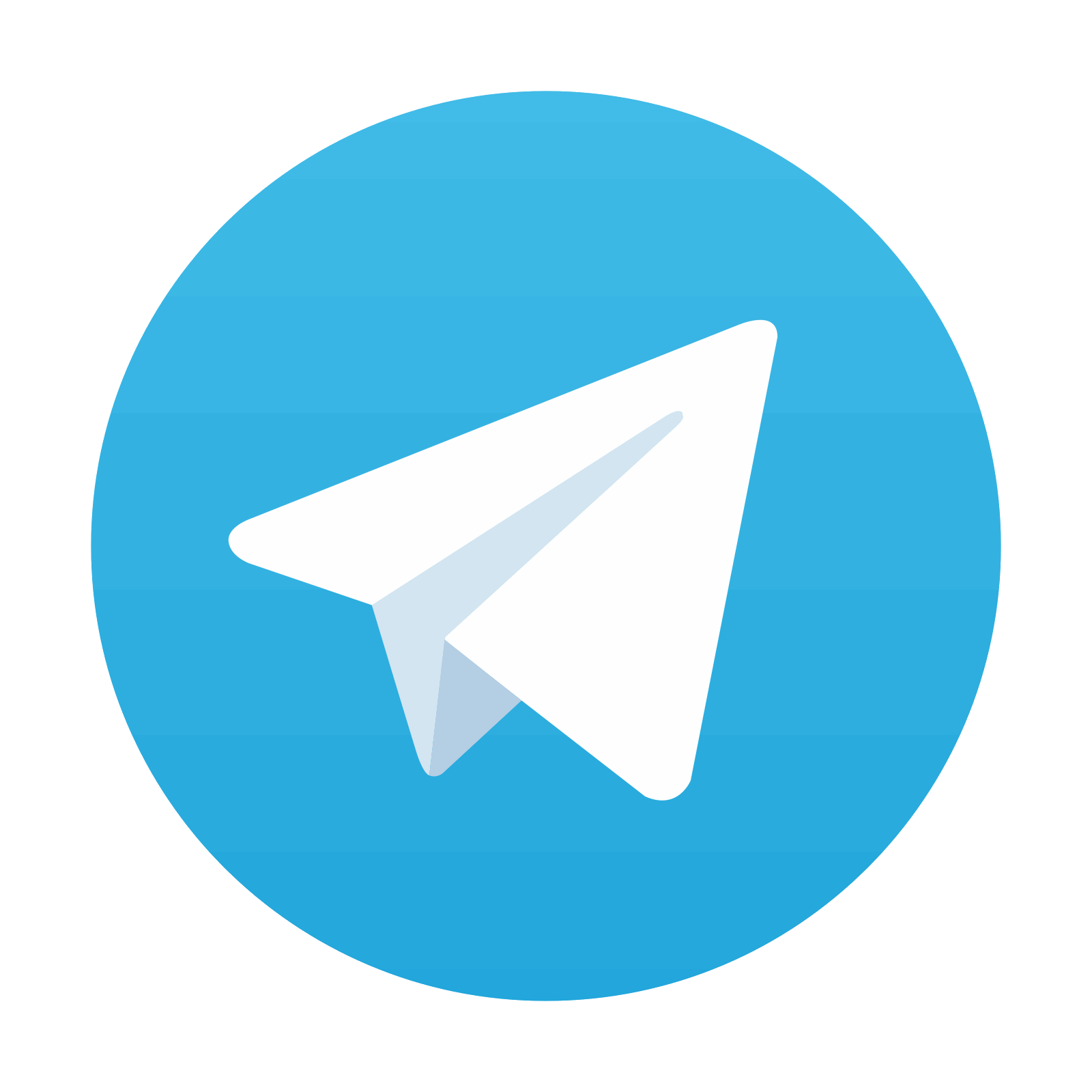
Stay updated, free articles. Join our Telegram channel

Full access? Get Clinical Tree
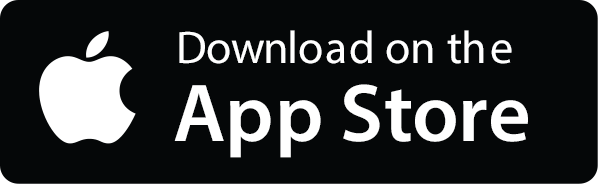
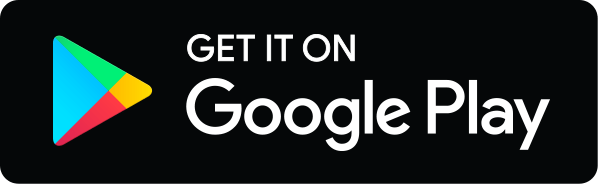