The abdominal aorta is frequently affected by both degenerative and occlusive disease. Since these diseases of the aorta frequently afflict the elderly, and with an aging U.S. population, surgical and endovascular treatment of aortic disease is common in a vascular specialist’s practice. Approximately 42 000 operations for the treatment of abdominal aortic aneurysms (AAA) were performed in the United States in 2003.1,2 Though in the past open surgical therapy for aortic diseases was the primary therapy, endovascular therapy of aortic diseases has emerged as an acceptable alternative to open aortic reconstruction and expanded the treatment options for those who treat aortic lesions. Advances in graft materials, surgical technique, and perioperative care have led to a marked reduction in perioperative morbidity and mortality with improvements in long-term results. With proper patient selection and appropriate procedure choice, the management of aortic diseases is one of the most rewarding areas of a modern vascular specialist’s practice.
The abdominal aorta is the final segment of the aorta and the continuation of the thoracic aorta beginning at the median aortic hiatus and terminating at the level of fourth lumbar vertebra by dividing into two common iliac arteries. The average diameter of the abdominal aorta is 2 cm (range of 1.4–3 cm). The abdominal aorta is frequently classified as suprarenal or infrarenal segments (Figure 30.1). The branches of the abdominal aorta are subdivided as either ventral, lateral, or dorsal. The ventral branches are unpaired visceral branches consisting of the celiac artery, the superior mesenteric artery, and the inferior mesenteric artery. The lateral branches are primarily paired visceral branches including the suprarenal artery, renal artery, and ovarian or testicular arteries. The inferior phrenic artery is also a lateral branch but is a paired parietal branch. The lumbar and sacral arteries are the dorsal branches of the abdominal aorta.
Though obstructive, atherosclerotic changes of the abdominal aorta are a frequent finding in the aging population, AAA is the most common disease of the abdominal aorta that requires treatment. First described by Vesalius, a 16th century anatomist, AAAs are defined by a diameter 50% greater than the expected diameter. This is frequently accepted to be a size of 3 cm or greater. When less than 50% diameter enlargement is encountered, it is defined as arteriomegaly or ectasia.
More than 90% of all AAAs are caused by degenerative changes of the aortic wall. However, atherosclerosis cannot be the sole factor leading to AAA development. Since the atherosclerosis theory does not completely explain the development of either occlusive or aneurysmal changes in the aorta of similar patients, aneurysmal changes of the abdominal aorta must be caused by a more complex degenerative mechanism than just atherosclerosis.
The most important matrix proteins in the aortic wall, which can be affected by aneurysmal changes and lead to AAA, are elastin and collagen. These proteins are arranged in such a manner to withstand intense arterial pressures, with the principal load-bearing element in the aorta being elastin. In the normal aorta, there is a gradual but marked reduction in the number of medial elastin layers from the proximal thoracic aorta (60–80 layers) to the infrarenal aorta (28–32 layers), accompanied by medial thinning and intimal thickening in the more distal aorta.3,4 Halloran and colleagues described a marked 58% decrease in elastin content between the suprarenal and infrarenal aorta and noted that this was the only location within the aorta where the proportion of elastin decreases relative to collagen.3 Because elastin fragmentation and degeneration is observed histologically in aneurysm walls, these observations may help explain the predilection for aneurysm formation in the infrarenal aorta.
Proteolytic degradation of medial elastin fibers and collagen degradation leading to the weakening and dilatation of the aortic wall is the most predominant pathophysiologic concept of aneurysm development.5 The medial layer destruction in aortic aneurysms is associated with an inflammatory response of the media and adventitia with infiltration of T and B lymphocytes, mast cells, and macrophages in response to oxidized lipids within the intima. Degradation and thinning of aortic media in response to these changes implies that these cells maybe responsible for the noted increase in proteolytic enzymes relative to their inhibitors. Numerous reports have documented increased expression and activity of matrix metalloproteinases (MMPs) in the wall of aortic aneurysms.6,7,8,9 MMPs are the zinc- and calcium-dependent proteolytic enzymes that are produced by macrophages. Smooth muscle cells and inflammatory cytokines are known to upregulate MMP production. There is an abundant wealth of data in the literature to suggest alterations in MMPs, including MMP-1 (collagenase-1), MMP-3 (Stromelysin-1), MMP-2 and MMP-9 (gelatinases), and MMP-12 (macrophage elastase), leading to AAA development.10,11 MMP-9 is of particular interest because it is the most abundant elastolytic proteinase having activity against insoluble elastin fibers. Abundantly expressed in aneurysm infiltrating macrophages at the site of tissue damage, MMP-9 as well as its synthesis is directly correlated with aneurysm size.12 Further evidence that MMP-9 may play a role in AAA pathogenesis was shown when an experimental murine model for aortic aneurysm targeting the deletion of the MMP-9 gene prevented aneurysmal degradation. Two potent elastolytic cysteine proteases, cathepsins S and K, may also play a role in AAA pathogenesis. These proteases are overexpressed in atherosclerotic plaques by macrophages and smooth muscle cells in the presence of proinflammatory cytokines found in aneurysm atheroma. Inhibition of proteases is also thought to play a part in AAA development. Cystatin C is the most abundant extracellular inhibitor of cysteine proteases and is severely reduced in aneurysmal lesions. The imbalance between cysteine proteases and cystatin C maybe responsible for the arterial wall remodeling in aneurysmal diseases.12,13,14,15
Histologic studies of AAAs have shown elastin fragmentation and chronic medial and adventitial inflammation. This differs from aortic occlusive disease, which shows inflammation of the intimal plaque. This transmural inflammatory infiltrate seems to be the key process for development of AAA, but its cause is not understood. Some studies have found Chlamydia pneumoniae in the wall of AAAs, which suggested that infection could be a possible causative agent for transmural inflammation. In rabbit models, Chlamydia can induce AAA. Other authors suggest an autoimmune source of chronic, transmural inflammation. An autoimmune component was hypothesized since the inflammatory cellular infiltrate of AAAs have shown the presence of B lymphocytes, plasma cells, and large amounts of immunoglobulin. Tilson and coworkers have identified a 40-kDa matrix protein, which may mediate an autoimmune response leading to aneurysm formation.16 Termed aortic aneurysm antigenic protein (AAAP-40), it is immunoreactive with IgG isolated from the aneurysm wall.17
In addition to reduced elastin content in the infrarenal aorta, hemodynamic and structural factors unique to this location predispose the infrarenal aorta to aneurysm formation. Reflected waves from the aortic bifurcation increase pulsatility and wall tension in the distal, less compliant atherosclerotic aorta. Absence of vasa vasorum in the infrarenal aorta has been suggested to reduce nutrient supply and potentiate degeneration. More recently, Xia and coworkers proposed an autoimmune mechanism for aneurysm formation and found that immunoreactive protein is more conspicuously expressed in the abdominal aorta compared with the thoracic aorta.17,18 This may explain the increased frequency of aneurysms in this location.
AAA development also has a genetic component reflected by an increased prevalence of AAA seen in siblings and first-degree family members. The defects in genes of connective tissue lines, especially the collagen III gene responsible for Ehlers-Danlos syndrome type IV, play a mechanistic role in the formation of some aneurysms. Any connective tissue disease, which negatively affects microfibrillar integrity, may lead to AAA development. Patients with Marfan’s syndrome, a disease of defective fibrillin, are particularly prone to aneurysmal disease.
Evidence also suggests that there maybe other independent, nonatherosclerotic AAA-forming pathways similar to chronic obstructive pulmonary disease (COPD). Lederle et al. conducted a systematic review of the relative risk of smoking on aortic aneurysms compared to other smoking-related diseases. The mechanism is still unclear, but smoking can lead to decreased aortic elasticity and induce MMP production by macrophages leading to degeneration of collagen and elastin of the arterial media and ultimately the development of an aortic aneurysm.
Less common causes of AAA include infection, cystic medial necrosis, vasculitis, trauma, and anastomotic pseudoaneurysms. Aortic aneurysms are rare in children and are most commonly associated with infection from umbilical artery catheters.
Rupture of AAA is the 13th leading cause of death in men older than 55 years of age.19 It is therefore important to know the epidemiology and etiology to help identify and reduce the mortality from AAA. The exact prevalence of AAA varies according to the criteria and methodology used.20 The first epidemiologic information was obtained from necropsy studies with an increased prevalence after the age of 55 years. An incidence of 4.7% in men 65 to 74 years old with a peak incidence of 5.9% by the age of 80 years was found. The prevalence among women was 1.2% between 65 and 74 years of age increasing to 4.5% above 90 years of age.21 Various estimates from screening studies have shown that the prevalence of AAA varies depending on age group screened, diagnostic method, criteria used to define AAA, and associated risk factors. AAA prevalence in the Wanhainen study ranged from 3.6% to 16.9% in men and 0.8% to 9.4% in women, depending on the different definition and diagnostic techniques used. The Aneurysm Detection and Management (ADAM) study identified AAAs in 4.6% of patients screened (infrarenal aorta 3 cm or larger) and 1.4% had an infrarenal aneurysm 4 cm or larger.22 Age, male gender, smoking, and family history had the most significant positive associations with AAA. Female gender, diabetes mellitus, and black race had negative associations with AAA. A family history of AAA has been documented in the literature with 15% to 25% of patients with AAA having a first-degree relative with an AAA. In a systematic review of the literature, it has been found that smoking and aortic aneurysm are closely linked. The relative risk associated with aortic aneurysm related events was 3 to 6 compared to 1 to 2 for coronary artery disease and 5 to 12 for COPD.23 The Veteran’s Administration cooperative study demonstrated that smoking was also an associated risk factor for 4 cm or greater AAA in 75% of AAA cases, with the association between smoking and AAA increasing with the number of years of tobacco use. High cholesterol levels, coronary artery disease, peripheral arterial disease, COPD, and hypertension have also been positively associated with AAA.
The majority of patients with AAA are asymptomatic with the AAA detected on routine physical examination or seen incidentally by noninvasive imaging. Occasionally the patient may note the ability to feel their heartbeat in their stomach, which leads to the diagnosis of an AAA. Symptoms of AAA are secondary to acute aneurysm sac expansion or rupture. The pathognomic features of a symptomatic or ruptured AAA are abdominal or back pain and pulsatile abdominal mass. Most ruptured AAAs are tender on palpation and associated with hypotension.
The presence of a pulsatile mass between the xiphoid and umbilicus when palpating the abdomen in a nonobese patient is the best way to detect an AAA on physical examination (Figure 30.2). Unfortunately, abdominal palpation has limited sensitivity for detecting AAA. The sensitivity of this technique depends on the AAA size, the obesity of the patient, the skill of the examiner, and the focus of the examination. The abdominal examination may detect large, easily palpable AAAs, but by itself cannot exclude the presence of an aneurysm. Small aneurysms may not be detectable by physical examination and obesity can hide even large AAAs. Moreover, physical examination cannot reliably distinguish between a clinically relevant AAA and an ectatic/tortuous aorta. Patients with hypertension and widened pulse pressure as well as patients with abdominal masses overlying the aorta may also lead to the incorrect diagnosis of an AAA.
Various diagnostic modalities are available to detect, measure, and monitor AAA. Plain abdominal x-ray or lumbosacral spine x-ray may demonstrate aortic wall calcifications. Measurements of the aneurysm may overestimate the size of the AAA because of the 20% magnification of the AAA by these types of x-ray. Ultrasonography (US) has been widely used in many published studies for AAA screening and has a sensitivity of 95% with near 100% specificity when used with adequate quality assurance.17 It is a useful tool to distinguish aneurysms from other conditions and there is a high degree of correlation between aneurysm size measured by US and operative size in both transverse and longitudinal diameter. Reported advantages for ultrasound include its simplicity and relatively low cost. It can be used to perform serial measurements of aneurysm growth, rapidly identify rupture, and to distinguish aneurysms from other conditions. The major limitation of US is that the abdominal aorta might not be well visualized as a result of obesity, excessive bowel gas, periaortic disease, or scarring from previous abdominal surgery. Moreover, the US measurements of the suprarenal aorta are not as reliable because of difficulty in visualization. Also noted was modest interobserver variability in US measuring of AP and transverse aneurysm diameters.23,24,25,26 Many physicians will generally follow patients with US surveillance until they reach the size of approximately 4 to 4.5 cm in diameter at which time more accurate monitoring with computed tomography (CT) imaging maybe utilized. Although it can be helpful for postoperative evaluation of endografts, it provides insufficient data for preoperative endograft planning.
Unlike US, contrast-enhanced spiral CT scanning provides more accurate information about the size, shape, and three-dimensional configuration of the AAA, independent of body habitus. CT scan can also provide more accurate anatomic information and better visualization of the suprarenal aorta and the iliac arteries. Compared to US, the major disadvantages of CT include its expense, use of iodinated contrast, and the radiation dose received.
Magnetic resonance imaging (MRI) in combination with magnetic resonance angiography (MRA) is another modality, which is as accurate as CT but more expensive and less available. Furthermore, it is not tolerated in claustrophobic patients and those with certain metallic implants. There is less familiarity with its use and image manipulation for endograft planning is less user-friendly. Unlike CT, bony landmarks are less well visualized. Gadolinium-based MR contrast agents, once thought to be safe in patients with chronic kidney disease, has now been associated with nephrogenic fibrosing dermopathy. This limits its use in this patient subpopulation.
Aortography, particularly digital subtraction angiography (DSA), is an excellent tool in defining perianeurysmal anatomy, particularly visceral and renal arteries (Figure 30.3). It can underestimate the size of aneurysms in the presence of nonspecified mural thrombus. Therefore, it is not useful in determining the size of AAA. Angiography is expensive and associated with the use of contrast and ionizing radiation. It is the most invasive imaging modality available in imaging AAA.
The natural history of an AAA is expansion and rupture, with rupture being the most dreaded complication of AAA. The risk of rupture increases with the diameter of the aneurysm, as predicted by LaPlace’s law. According to Laplace’s law, the wall tension of the AAA is directly proportional to the radius and blood pressure and inversely proportional to the wall thickness. Rupture risk also increases with an increased rate of expansion and certain comorbid conditions, particularly COPD. According to the UK Small Aneurysm Trial, 25% of patients with a ruptured AAA died without ever reaching the hospital, 51% died in the hospital without undergoing surgery, 13% died within 30 days of surgery (46% operative mortality rate), and only 11% survived beyond 30 days.27,28 The short-term mortality (30 days) associated with elective surgical repair is 2.7% to 5.8%, based on ADAM and UK Small Aneurysm Trial28,29. Aneurysm rupture is associated with a mortality rate of 60% to 90%.30 The risk of rupture is independently associated with female gender, larger initial aneurysm diameter, current smoking, and higher mean blood pressure. Although aneurysms are less frequently observed in women, AAA in women has a threefold increased risk of rupture compared to men with similar sized aneurysms. According to the UK Small Aneurysm Trial, women with a 4 to 5.5 cm AAA are at four times higher risk of rupture compared to men. The reason most often cited for this phenomenon relates to the smaller aortic diameter and the classical recommendations for repairing aneurysms when they reach 5 to 5.5 cm.
The annual risk of rupture of an AAA with a diameter of 5.5 to 5.9 cm is 9.4%, 10.2% for an AAA between 6 and 6.9 cm, 19.1% for an AAA between 6.5 and 6.9 cm, and 32.5% for an AAA of 7 cm or larger.27,28,31 The rate of expansion is also an important determinant of rupture. The mean rate of expansion in ruptured aneurysms is 0.82 cm/year compared to 0.42 cm/year in nonruptured aneurysms. Effective control of blood pressure and cessation of smoking are likely to diminish the risk of rupture.
The routine screening of asymptomatic patients has not been shown to be cost-effective for patients younger than 65 years of age because of the low prevalence of AAA. Patients with a family history of AAA or men older than 65 years of age with history of smoking or hypertension may benefit from aneurysm screening. This has been addressed by major trials like the Multicenter Aneurysm Screening Study (MASS). A single normal ultrasonographic scan at age 65 years in men virtually rules out significant aneurysm disease for life. Presently, Medicare has approved US screening for high-risk individuals when they enter the program at the age of 65 years.
All patients with AAA should have aggressive cardiovascular risk factor modification. Arguably, blood pressure should be controlled with ACE inhibitors and possibly beta-blockers. Patients should undergo routing surveillance of AAAs to determine aneurysm morphology, growth rate, and absolute sizes. After the initial diagnosis, a follow-up imaging study at 6 months is often indicated to determine the growth curve for the aneurysm. If there is no rapid expansion and the aneurysm is under 4 cm in diameter, then annual surveillance is reasonable. Beyond 4 to 4.5 cm, the AAA should be followed every 6 months. Surgical intervention is recommended when the size of the aneurysm is above 5 to 5.5 cm in diameter, when it is expanding rapidly at a rate of more than 0.5 cm in 6 months, or when symptomatic. Options for surgical repair include the traditional transperitoneal route or a retroperitoneal approach. The transperitoneal approach is the most widely used approach for infrarenal aortic disease. The advantages being faster opening and closing of the incision, the ability to explore the concomitant abdominal organs, and better access to the right iliac and right renal arteries, which facilitates repair of these vessels. The retroperitoneal approach is preferred in patients with scarring from intraabdominal inflammation or previous surgery. It can also be used to access the aorta through the contralateral retroperitoneum in patients with colostomy or ileostomy. The operative steps are detailed in Figure 30.4(A–C).
The mortality associated with elective surgery is 2.7% to 5.8% and increases to 19% for urgent aortic repair and at least 50% for repair of a ruptured aortic aneurysm.28,29 According to the ACC/AHA guidelines, open surgical repair is recommended for patients with low or average risk of operative complications. High-risk patients need to be evaluated closely to determine their fitness for repair.
Although endovascular aneurysm repair (EVAR) is recommended for patients with high risk of complications with open repair, it is gaining popularity because of its lower morbidity, quicker recovery times, and lower early mortality. The EVAR-1 trial is a randomized controlled trial of 1082 patients enrolled between 1999 to 2003 comparing 30-day mortality between elective endovascular repair or open AAA repair. The study results demonstrated a 30-day mortality in the EVAR group of 1.7% vs. 4.7% in the open repair group (p = 0.009), but more secondary interventions were required with endovascular repair (9.8% vs. 5.8%). The EVAR group also had a significantly shorter hospital stay (7 vs. 12 days), compared to open repair.32
Further benefits for EVAR over open AAA repair were demonstrated in the Dutch Randomized Endovascular Aneurysm Management (DREAM) trial. The DREAM trial was a multicenter, randomized trial comparing open repair with endovascular repair in 345 patients with at least 5 cm aneurysms. The study outcomes were operative (30-day) mortality and severe complications. The operative mortality rate in the endovascular repair group was 1.2% vs. 4.6% in the open-repair group. The combined rate of operative mortality and severe complications in endovascular group was 4.7% vs. 9.8% in the open-repair group. The study concluded that the endovascular repair is preferable to open repair in patients with AAA of at least 5 cm in diameter.33
Despite the benefit shown for EVAR, its use in those unfit for open repair was brought into question by the EVAR-2 trial. EVAR-2 was a randomized controlled trial of EVAR outcomes in patients unfit for open repair of AAA. The study randomized 338 patients aged 60 years or older with at least 5.5 cm AAAs to receive either EVAR or no intervention. The primary endpoint of the study was all-cause mortality. Secondary endpoints were aneurysm-related mortality, health-related quality of life (HRQL), postoperative complications, and hospital costs. The 30-day operative mortality in the EVAR group was 9% (13 of 150, 95% CI 5–15) and the no intervention group had a rupture rate of 9 per 100 person years (95% CI 6.0–13.5). There was no significant difference between the EVAR group and the no intervention group for all-cause mortality (hazard ratio 1.21, 95% CI 0.87–1.69, p = 0.25). Moreover, there was no difference in aneurysm-related mortality or HRQL scores. The mean hospital costs per patient over 4 years in the no intervention group were £8649 less than in the EVAR group. The study concluded that EVAR repair or observation has no difference in all-cause or aneurysm-related mortality after 4 years in this high-risk surgical group.34
The major advantages of EVAR include reductions in major morbidity, intubation time, hospital stay, blood loss, and a faster return to normal activity after the repair. Despite the apparent early advantages to EVAR, long-term outcomes are equivalent to open surgery in both observational studies and randomized trials. The survival advantage associated with endovascular repair has been shown to be lost after 1 year. As shown in the DREAM trial, the cumulative survival at 2 years was not different with EVAR or open repair yet EVAR was associated with increased repeat interventions.35 The EVAR-2 trial also did not show survival difference between EVAR or no intervention at 4 years in those patients unfit for open repair.34,36 These observations have led many vascular specialists to temper their initial enthusiasm for endovascular treatment of AAA.
Endovascular repair involves femoral artery access, placement of an endoluminal stent-graft via the iliac arteries, and deployment of an aortobiiliac stent graft, excluding the aneurysmal lumen from pressurized blood flow and thus minimizing the risk of rupture. A variety of endografts are commercially available (Figure 30.5). Prior to EVAR, thin cut (≤3 mm) contrast-enhanced spiral CT is the only imaging modality necessary to plan the placement of a stent graft. Intraoperative angiography, and in selected cases intravascular ultrasound (IVUS), are essential to determine the appropriate size and length of endograft needed. Anatomic considerations for preoperative EVAR planning include proximal neck length/diameter, distal neck length/diameter, total length, neck angulation, femoral/iliac artery diameters, mesenteric and accessory renal artery locations, as well as occasionally an aberrant artery of Adamkowicz. (Figures 30.6 and 30.7). Follow-up of patients after endovascular repair is typically done at 1 and 6 months, and annually thereafter with CT angiography though US maybe used in selected cases. Abdominal plain films can be utilized to look for graft migration. Post-EVAR evaluation includes aneurysm diameter, presence of an endoleak, or graft migration/integrity.
FIGURE 30-5.
Commercially available endografts. (A) AneuRx graft.
Courtesy of Medtronic, Inc, Minneapolis, MN.
(B) Zenith flex AAA endovascular graft.
Courtesy of Cook Medical, Bloomington, IN.
(C) Gore excluder bifurcated endoprosthesis—assembled bifurcated graft.
Reproduced, with permission, from Kinney TB, Rivera-Sanfeliz GM, Ferrara S. Stent grafts for abdominal and thoracic disease. Appl Radiol. 2005;34:9-19.
(D) Endologix graft.
Courtesy of Endologix, Irvine, CA.

Post-EVAR complications include endoleak, endotension, endograft limb occlusion, endograft migration, and postimplantation syndrome. An endoleak is defined as a persistent flow of blood into the aneurysm sac after EVAR and is classified into types I to IV. Endoleak can lead to pressurization of the aneurysm sac with aneurysmal expansion and rupture. About 20% to 40% of patients develop endoleaks after EVAR.
A type I endoleak is caused by inadequate seal at either the proximal (Ia) or distal (Ib) attachment site, and has an incidence up to 10%. Appropriate oversizing of the proximal portion of the endograft by at least 10% to 20%, as recommended by the graft manufacturers, should reduce the rate of type I endoleaks. Risk factors for type I leaks include over- or undersizing of the proximal/distal attachment sites, short seal zones, and significant angulation at the fixation zones. The classic dogma is that during implantation, if a type I (or type III) endoleak is encountered, the procedure is not complete until this is corrected.
Type II endoleaks are secondary to retrograde flow of blood into the aneurysm sac from aortic branch vessels (the inferior mesenteric artery or lumbar arteries). Type II endoleaks are the most common, occurring after 10% to 25% of all EVARs. It is rare for type II leaks to lead to significant pressurization or expansion of an aneurysm sac. Most patients with type II endoleaks can be followed closely with serial imaging studies. If aneurysm sac expansion occurs on surveillance imaging, several treatment options are available to eliminate retrograde flow into the sac. Embolization of branch arteries, thrombogenic packing of the aneurysmal sac, laparoscopic branch ligation, or conversion to open repair are methods to treat type II leaks. The timing of the occurrence of type II endoleak (early vs. late) as well as sac enlargement (either in diameter or volume) is a predictor for subsequent intervention.
Type III endoleaks are caused by graft tear or a leak from attachment sites of modular components (“modular disconnect”). Like type I endoleaks, type III leaks result in systemic pressurization of the aneurysm sac and should be repaired. The repair is often accomplished by sealing of the leak with the placement of another endograft component, such as a cuff proximally or extension limb distally.
Type IV endoleaks are caused by graft porosity. The incidence of type IV endoleak is diminishing as a result of modifications in graft materials. Angiographically, this appears as a diffuse blush from the endograft into the aneurysm sac. Most type IV endoleaks, when identified at the time of surgery, can be followed and will show resolution on follow-up CT imaging.
Classically, endotension is defined as the persistent pressurization of the aneurysm sac in the absence of an endoleak. It likely results from aortic pulsatility against thrombus or undetectable endoleaks.31 Endotension can lead to sac rupture and enlargement. The clinical significance of endotension needs further study. There are ongoing newer modalities including multidimensional CT angiography measurements or implantable pressure transducers, which are being examined to measure endotension, identify endoleaks, and aneurysm sac expansion.37
Postimplantation syndrome is an acute inflammatory response developing within the first few weeks following endovascular stent placement. Its etiology is unknown but is thought to be related to AAA thrombus formation and is associated with the development of fever, leukocytosis, and elevated C-reactive protein (CRP). Most symptoms related to postimplantation syndrome will resolve within 6 weeks.
Medical therapy is beneficial in patients with aneurysms of all sizes, though no significant single therapy has been clearly shown to inhibit the progression of aneurysm growth. The risk of aneurysm formation increases by 20% to 25% with continued smoking and is a major risk factor for aneurysm growth and rupture. Hence smoking cessation should be strongly emphasized. The current ACC/AHA guidelines recommend the control of lipids and blood pressure similar to patients with atherosclerotic disease (coronary heart disease equivalent). All patients with AAA should be on statin therapy for secondary prevention of cardiovascular events. There is experimental and limited retrospective data suggesting statin therapy might suppress aneurysm growth possibly via an anti-inflammatory effect, but more human studies will be needed to prove this observation. ACE inhibitors have also been suggested to reduce the likelihood of aneurysm growth/rupture, however there is no level I evidence to support its use.38
Inflammatory AAAs are a subset of AAAs representing less than 5% of all aneurysms. They are a distinct clinical entity marked by thickening of the aneurysm wall anteriorly and laterally, perianeurysmal and retroperitoneal fibrosis. This extensive inflammatory reaction frequently involves the vena cava, renal vein, duodenum, and ureters. Inflammatory aneurysms are thought to have an inheritance of specific HLA alleles with a higher incidence of HLA-DRB1. Traditionally, they present with a triad of abdominal/flank pain, fever, and constitutional symptoms. On CT scan, a “halo” sign is often seen with an anterior predominance of inflammatory tissue, which will enhance with intravenous contrast administration. The operative risk associated with inflammatory aneurysms is higher with an operative mortality of 7.9 versus 2.4% for traditional AAAs. Preoperative recognition of an inflammatory aneurysm may facilitate the selection of a left retroperitoneal approach to avoid the most inflamed portion of the aneurysm. Although not extensively studied, an endovascular approach maybe used for this subset of aneurysms. Meta-analysis of EVAR in inflammatory aortic aneurysms of the abdominal aorta showed a primary technical success rate of 95.6% with regression of the aneurysm sac median diameter by 11 mm over a mean follow-up of 18 months after endovascular repair. Of 43 patients with periaortic fibrosis prior to the stent graft, 22 (51.2%) patients showed complete regression, 18 (41.8%) remained unchanged, and 3 (7.0%) showed inflammatory progression after EVAR. Renal impairment resolved in 11 (45.8%) of 24 patients. Reinterventions were required in 8 patients. The procedure-related and follow-up (18 months) mortality rates were 0% and 13.0%, respectively. The study concluded that EVAR of inflammatory aortic aneurysm is feasible and excludes the aneurysm effectively, reduces periaortic fibrosis, and improves renal impairment in most patients with very low periprocedural and midterm mortality (18 months).6
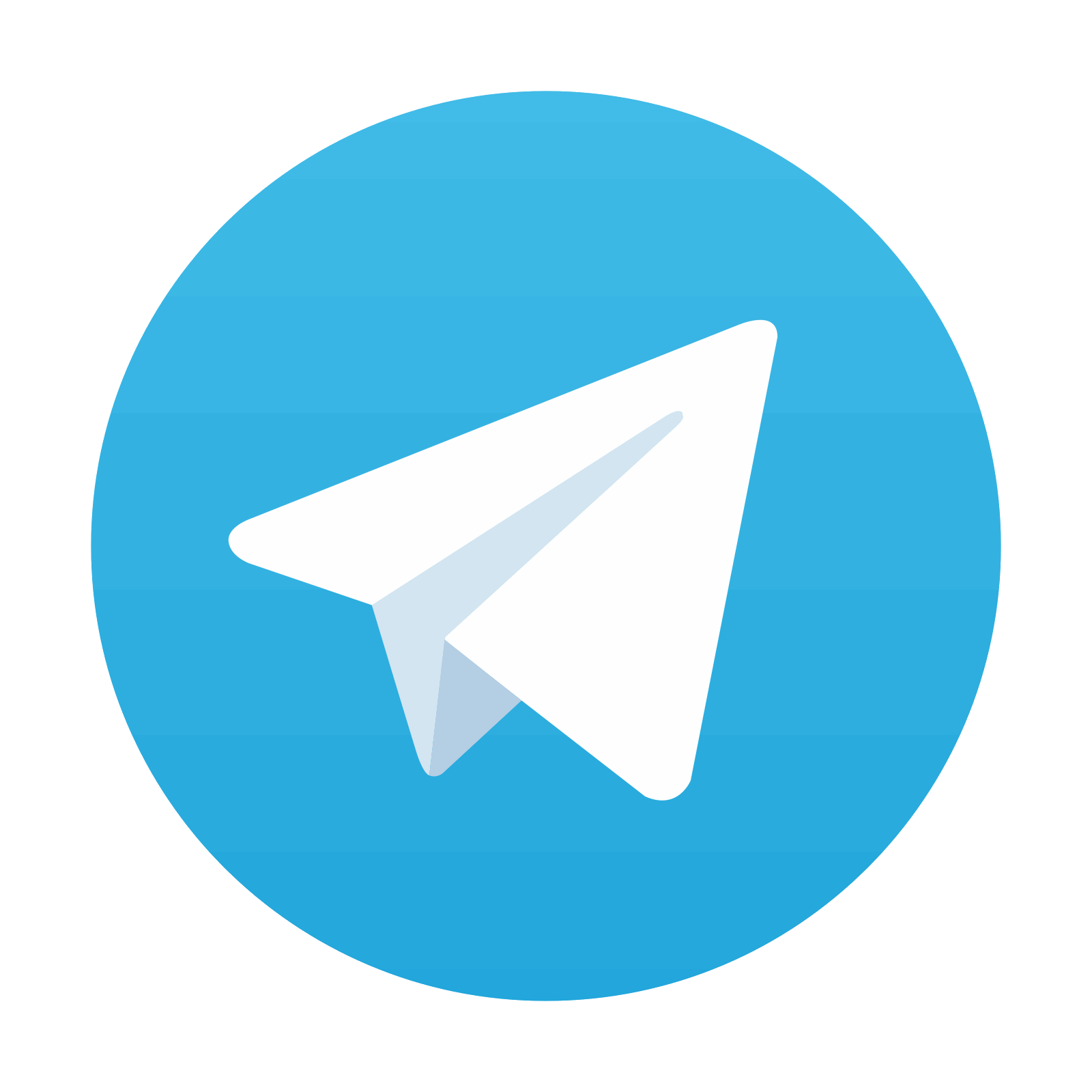
Stay updated, free articles. Join our Telegram channel

Full access? Get Clinical Tree
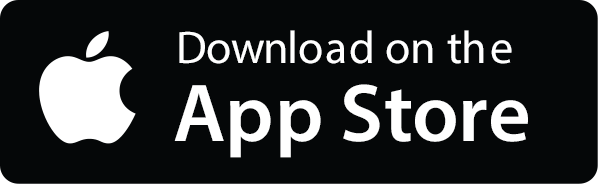
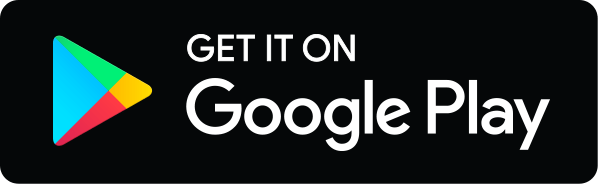