9
Viral Infections of the Respiratory Tract
Viral infections of the respiratory tract are important causes of morbidity in all age groups, but especially in the young and the elderly, as well as in the immunocompromised. The most important respiratory viruses are shown in Table 9–1.
Although different viruses may predominantly damage different portions of the respiratory tract (Table 9–2), there is considerable overlap. Upper respiratory tract illnesses are those that cause any combination of nasal obstruction, nasal catarrh, sneezing, sinusitis, sinus pain, sore or scratchy throat, hoarseness, or loss of voice.1 Lower respiratory tract illnesses are those that produce cough, possibly with sputum production, or shortness of breath and wheezing, which is either new or significantly elevated above baseline. Laryngitis produces upper respiratory tract symptoms, whereas tracheobronchitis produces lower respiratory tract symptoms.
Viral infections may be categorized into several clinical syndromes.2 A “cold” may include tonsillopharyngitis, pharyngitis, sinusitis, otitis media, and epiglottitis, and is characterized by upper respiratory tract symptoms. The “influenza” syndrome, which may be caused by viruses other than influenza, includes abrupt fever, headache, myalgias, and malaise.3,4 Lower respiratory tract symptoms are found in only 10% of patients with this syndrome, usually the elderly or chronically ill. Acute bronchitis refers to productive cough without radiographic or clinical pneumonia. It is often accompanied by retrosternal tracheal pain.5 This syndrome is typically more severe and prolonged for patients with preexisting chronic bronchitis.6 Bronchiolitis is characterized by airflow obstruction, wheezing, and radiologic evidence of hyperinflation, atelectasis, or both.7 On high-resolution computed tomography (HRCT), the irregular hyperinflation results in a “mosaic” pattern. Viral pneumonia may occur with or without cough and is characterized by diffuse radiographic infiltrates. Viral pneumonia in immunocompetent adults is usually mild and is most commonly due to influenza. Life-threatening illness due to influenza, however, does occur, most commonly in the elderly or the chronically ill.8 Less common causes of viral pneumonia, with the exception of hantavirus and the coronavirus of severe acute respiratory syndrome (SARS), as indicated in Table 9–2, are usually seen in immunocompromised patients.9
The radiologic patterns of viral infections of the lower respiratory tract are variable and, like the clinical syndromes, overlap. These consist primarily of patchy or diffuse ground-glass opacities, with or without areas of consolidation.10 Computed tomography may show poorly defined centrilobular nodules, areas of ground-glass attenuation with or without a lobular distribution, or segmental consolidation.10 There is not a unique consistent correlation between the radiologic findings and a particular type of virus.11 In addition, the radiographic patterns of involvement cannot reliably distinguish between viral pneumonia and bacterial pneumonia, particularly that caused by Mycoplasma or Chlamydia.12
Traditionally, the “gold standard” for the identification of viruses has been by culture from respiratory secretions or tissue, but newer molecular techniques are changing the status quo significantly.13 Unfortunately, both traditional viral culture and the newer molecular techniques are often unavailable in many hospitals. When viral culture is not employed in a timely manner, false-negative cultures may occur secondary to the loss of the reproductive capability of the virus. This is commonly seen with respiratory syncytial virus (RSV), a somewhat labile virus. Although cultures may be negative, in some cases the direct immunofluorescent stain that detects stable antigens of the virus may be positive, for example, RSV.14,15 A variety of laboratory methods for the optimal detection of viruses have been and are being developed, and are described in detail elsewhere.14–17 These techniques include serologic diagnosis, and microscopy, often using immunofluorescently labeled antibodies, enzyme immunoassays (EIAs) for the detection of viral proteins, and nucleic acid amplification techniques, most notably real-time nucleic acid amplification methods.18 The rapid differentiation of viral versus bacterial infections is important for achieving the appropriate diagnosis, and by doing so allows for the appropriate use of antibiotics.19 Nucleic acid amplification tests, in addition to being useful for the detection of viruses in clinical specimens, have also proven useful for the diagnosis of viral pneumonitis from fixed lung tissue.20
| RNA Viruses | |
---|---|---|
Virus Family | Common Viral Agents | Less Common Viral Agents |
Orthomyxoviridae | Influenza A and B | Influenza C |
Paramyxoviridae | Parainfluenza 1–3, RSV | Measles, parainfluenza 4 |
Picornaviridae | Rhinovirus, enterovirus, Coxsackievirus, echovirus | |
Coronaviridae | Common coronavirus | SARS agent |
DNA Viruses | ||
Adenoviridae | Adenovirus | |
Herpesviridae | HSV 1 and 2, VZV, CMV |
CMV, cytomegalovirus; HSV, herpes simplex virus; RSV, respiratory synctial virus; SARS, severe acute respiratory syndrome; VZV, varicela-zoster virus.
The serologic diagnosis of a viral infection has traditionally required demonstration of a rise in the virus-specific antibody titers in paired acute and convalescent sera. This approach has been complicated to a certain degree by newer EIAs that do not provide titers. The EIAs, however, are often specific for either immunoglobulin M (IgM) or G (IgG), which provide comparable information regarding acute or remote infection. Serologic diagnosis, though one of the older technologies in the laboratory, remains important. For example, the West Nile virus currently spreading across North America is a very tissue-associated virus with a short viremic period. In our experience, the diagnosis of disease caused by West Nile virus has been more effectively achieved by serologic testing than by reverse-transcription polymerase chain reaction (RT-PCR).
Several microscopic techniques are available for the rapid detection of viruses, and are most commonly used routinely for respiratory viruses, adenovirus, and some of the herpes viruses. The demonstration of cells infected by virus in nasopharyngeal secretions by fluorescent antibody techniques is well established and remains a useful tool for the rapid diagnosis of viral infections.16,21 These methods provide more rapid results than culture, and may detect infection even when the virus cannot be cultured.7,8 Fluorescent antibody testing on touch preparations or frozen sections of lung tissue might theoretically be useful, but the technique presents difficulties in interpretation, particularly due to autofluorescence of lung tissue, and is not widely practiced. The detection of viral antigens in formalin-fixed, paraffin-embedded tissue by immunoperoxidase reactions is also possible. Cytomegalovirus (CMV) is readily demonstrated in the lungs of immunocompromised patients who develop CMV pneumonitis.22,23 The routine detection of other viruses by these methods, however, is limited, often by the commercial availability of antibody and the low clinical demand for such tests.
Radioimmunoassay, or now more commonly EIA, may also accomplish the detection of viral proteins. Many commercially produced EIAs are available for the rapid detection of the most common respiratory viruses (influenza and RSV). Although these are useful if positive (i.e., they have high specificity and, therefore, a high positive predictive value), they are less sensitive than the corresponding direct fluorescent antibodies and culture, and do not usually suffice as the sole means of diagnosis.24,25
A major criterion used to make a microscopic diagnosis of a viral infection of the respiratory tract in tissue sections or cytologic preparations is the presence of characteristic inclusion bodies. The various inclusions are discussed in this chapter under the specific viruses, but not all respiratory viruses have recognizable inclusions, and inclusion mimics such as prominent nucleoli may lead to erroneous diagnoses.26 Therefore, in equivocal cases the specific techniques described above can be very helpful.
Electron microscopy, although time-consuming, may occasionally be useful in confirming the presence of viral particles. The electron-microscopic appearance of the various respiratory tract viruses is discussed in detail in this chapter. Viral particles may be identified in negatively stained preparations from secretions or exudates, but these techniques are rarely used, given the widespread use of direct immunofluorescent stains. Electron microscopy remains an important tool, however, for the investigation of emerging pathogens, and was used recently in elucidating the infectious agent of SARS as a coronavirus.27,28 Electron microscopy may also be used to identify viruses from formalin-fixed, paraffin-embedded tissues; this means that analysis can be done even if a viral process was not considered in the differential diagnosis at the time of initial collection, when viral cultures could have been performed and fresh tissue made available for other studies.29 The drawbacks of using routing electron microscopy are cost, prolonged time to detection, the presence of “pseudoviral” particles in tissue and preparation artifacts that may cause false-positive results, as well as the inability to differentiate members of the herpesvirus family, such as herpes simplex virus HSV and varicella-zoster virus (VZV), without additional special techniques, for example, immuno-electron microscopy.
The diagnosis of viral infections using nucleic acid amplification technology is evolving rapidly. Older, more laborious assays, which were often not well suited to the clinical laboratory, are being replaced by newer methods, most notably real-time nucleic acid amplification.18,30 Real-time PCR or similar technologies combine the basic principles of PCR with more rapid temperature changes to reduce the cycling time. Perhaps more importantly, these technologies employ a variety of fluorescently labeled hybridization probes, which achieve detection of the amplified product (amplicon) in the same tube in which the reaction has occurred, thereby significantly diminishing the threat of amplicon contamination in the laboratory and the need for postamplification analysis (i.e., Southern blots or EIAs). Although RNA has been particularly difficult to detect in the past because of its lability, advances in total nucleic acid extraction methods and more efficient means of amplifying RNA have made the molecular detection of RNA viruses a reality.30–32
The most typical histologic appearance of viral pneumonia is diffuse alveolar damage, often with bronchitis and bronchiolitis (Table 9–3). The diagnosis of a specific viral pathogen can frequently be made by identifying one or more unique features as discussed below for each virus.
Influenza
The overwhelming majority of lower respiratory tract viral infections in the nonimmunocompromised adult are caused by the influenza virus.33 Influenza occurs as pandemics, regional epidemics, and local sporadic disease, the latter two forms of disease being most common.34 Influenza occurs predominantly during the winter, in relatively short-lived outbreaks (2 to 4 weeks) that have similar clinicopathologic features from outbreak to outbreak.34
The epidemiologic patterns, in many respects, are influenced by the external antigens of the virus.35 There are three major types of human influenza virus, A, B, and C, with the majority of disease caused by type A. Influenza B is less common than influenza A, but causes clinically similar disease. Influenza C predominantly causes a mild upper respiratory tract infection and is not considered a significant public health threat.34,36 The envelope proteins of the influenza viruses, hemagglutinin, and neuraminidase are critical for infection and replication, and are also the proteins used to type and subtype these viruses. Hemagglutinin is responsible for attaching to the host cell and initiating infection, whereas neuraminidase is responsible for the release of mature virions from infected cells. Blocking the neuraminidase activity of the influenza virus is the mechanism of action of the new therapeutic agents for influenza, zanamivir and oseltamivir.37
Mutations that occur during RNA replication and accumulate over time result in “antigenic drift” in the encoded hemagglutinin and neuraminidase proteins.38 This drift helps the virus evade the immune response, at least temporarily, and is responsible for yearly recurrences and epidemics.39 Influenza, with its segmented genome, is also capable of undergoing major recombination events, known of as “antigenic shift,” which some believe are responsible for pandemics of influenza.3
Influenza is unusual in neonates, presumably because of protection by maternal antibodies. Older children have a higher incidence of influenza infection than adults. Infected children also have a higher maximal temperature and more cervical adenopathy compared with adults.40,41 Disease limited to the upper respiratory tract is not uncommon in childhood influenza, and in fact ~40% of children requiring hospitalization from influenza infection present with convulsions, possibly secondary to high fever, rather than specific respiratory complaints.42 Occasionally childhood influenza cases may lead to serious postinfection sequelae such as bronchiolitis obliterans or, with aspirin use, Reye’s syndrome.43,44
Otherwise healthy adults ordinarily recover from typical influenza lower respiratory tract infection, but there is a serious risk of fatality in the elderly, particularly those who have underlying chronic cardiovascular or respiratory diseases; this risk is equivalent in epidemic and nonepidemic situations.45,46 Suffering and death secondary to influenza is especially tragic because most cases are preventable with the recommended use of current vaccines.47,48
The influenza virus infects and replicates in epithelial cells, as well as leukocytes; it regulates host cell transcription and translations pathways, downregulates apoptotic pathways, and results in the production of chemotactic, proinflammatory, and antiviral cytokines.49
Morphology
The gross appearance of the lung in influenza pneumonia is nonspecific, which is the case for all viral pneumonias. In fatal cases, the lungs are heavy, edematous, congested, hemorrhagic, often with increased firmness, and often with obvious tracheobronchitis.50
The pathology varies somewhat depending on the disease severity.51 In severe cases, there is conspicuous damage to the conducting airways, with necrotizing bronchitis and bronchiolitis within the first 4 to 10 days (Fig. 9–1), often accompanied by a hemorrhagic exudative diffuse alveolar damage with hyaline membranes (Fig. 9–2).52,53 By 2 to 3 weeks, the conducting system demonstrates organization of the necrotic epithelial and inflammatory debris, along with reparative changes of the mucosa, frequently with squamous metaplasia (Figs. 9–3 and 9–4). The parenchyma shows organizing diffuse alveolar damage with type II pneumocyte proliferation. The lesions may ultimately heal with a nonspecific pattern of interstitial fibrosis.53–55 Less severe patterns of involvement are manifest as mild acute lung injury with fibrinous alveolar exudates, alveolar septal edema type II cell reactivity, and small foci of early organization. A nonspecific pattern of organizing pneumonia (bronchiolitis obliterans organizing pneumonia, BOOP) may also be observed. A secondary bacterial pneumonia may be superimposed on either of these patterns.
FIGURE 9–1 Early influenza pneumonia with intraluminal debris [hematoxylin and eosin (H&E, × 180)].
FIGURE 9–2 Influenza pneumonia manifest as diffuse alveolar damage with robust hyaline membranes (H&E, × 130).
FIGURE 9–3 Superinfected influenza pneumonia. Note thickened interstitium and squamous metaplasia (arrowhead) (H&E, × 130).
In some instances, damage to the conducting airways may be more severe proximally than distally.52 This observation correlates with an experimental model of influenza A infection that demonstrated that less virulent influenza strains were more likely to remain confined to the upper respiratory tract, whereas more virulent strains were more likely to spread distally and produce bronchial and bronchiolar epithelial necrosis.56,57
Light microscopy of bronchial epithelial cells infected with influenza virus may show nonspecific intracytoplasmic basophilic inclusions, but the presence or absence of these is not diagnostically helpful. Ciliocytophthoria, or the detached, ciliated apical fragments of respiratory epithelial cells, may be present in cytologic preparations from persons with influenza or other respiratory virus infections, and have erroneously been mistaken for parasites.58 Although nonspecific, these provide a clue to the possibility of a viral infection. Negatively stained electron microscopic preparations of intact viral particles show generally rounded, sometimes pleomorphic particles 80 to 120 nm in diameter with surface projections of the surface glycoproteins.29 Thin sections examined by transmission electron microscopy may demonstrate viral particles, as well as filamentous nucleocapsid material in the cytoplasm, measuring ~10 nm in diameter. Finally, PCR methods have been used to identify the influenza virus in fixed lung tissue but, unfortunately, are not widely available.20
Measles
Pneumonia is the most common complication of measles in immunocompetent patients, although the cited incidence varies widely from less than 5% to almost 40%, with ~50% of the measles-related pneumonias due to bacterial superinfection.59–61 Although currently well controlled in developed countries through vaccination, measles remains an important cause of disease in developing countries, where it accounts for from 8 to 93% of the morbidity from acute lower respiratory tract infections.62 For example, a review of the records of the pediatric ward of Goroka Base Hospital, Papua New Guinea, in 1989 revealed that measles accounted for 11% of the admissions and 32% of the deaths.63
Asymptomatic chest film changes, both parenchymal and hilar, are frequently seen in otherwise uncomplicated cases of measles (rubeola).33,60 Although the majority of patients with measles completely recover from the disease, a minority develop complications, such as life-threatening pneumonia.61,64 Serious measles pneumonia is much more common in patients with immunodeficiencies, in whom it may appear without a rash or Koplik spots.9,64–67
FIGURE 9–4 Organizing influenza pneumonia with polypoid plugs of granulation tissue within airspaces forming a bronchiolocentric nodule.
The clinical course of complicated measles is variable. Patients may have respiratory complaints for a few days to several weeks. Early in the course of disease, the radiologic appearance is one of diffuse fine nodules that measure 1 to 3 mm. These become confluent with time and may eventually result in diffuse opacification.64 In immunocompetent patients with measles, inclusion-bearing giant cells may be seen in epithelium of a variety of organ systems, including the upper and lower respiratory tract during the prodromal stage, but these disappear as the rash develops.64,67 There is a relationship between development of rash, the presence of tissue giant cells, the presence of culturable or immunohistologically identifiable virus, and antibody response. The development of the rash is thought to be an expression of intact cellular immunity, and in general the better developed the rash, the fewer the number of giant cells in tissue.64 Absent or slowly developing rash, persistence of tissue giant cells, persistence of culturable virus, and poor antibody response are all indicators of inadequate reaction to virus and subsequent poor prognosis; one or more of these factors are characteristically found in fatal cases. This also supports the finding that severely immunocompromised patients with severe-to-fatal measles giant cell pneumonia often present without a rash. The persistence of tissue giant cells in fatal cases is of historic interest, because cases morphologically similar to Hecht’s giant cell pneumonia were subsequently proven to be due to measles infection in immunocompromised patients without the typical rash.68,69
Morphology
The gross appearance of the lungs ranges from normal, to finely nodular, to diffusely edematous and hemorrhagic similar to what can be seen with influenza. Microscopic features may include type II pneumocyte hyperplasia, squamous metaplasia of the bronchial epithelium, peri-bronchial and interstitial mononuclear cell infiltrates, and diffuse alveolar damage (Fig. 9–5).70 Occasional inclusions in the trachea and major bronchi may be seen, with a striking extension of this process into the submucosal glands. The terminal and respiratory bronchioles may show exuberant hyperplastic and meta-plastic changes that extend into the nearby alveoli, provoking a fibroblastic response and giving rise to the nodular quality of the early lesions.64 The remainder of the parenchyma often shows diffuse alveolar damage with multinucleated giant cells of alveolar epithelial derivation containing both intranuclear and intracytoplasmic inclusions (Fig. 9–6).
FIGURE 9–5 Diffuse alveolar damage in measles with hyaline membranes (arrowhead) and multinucleate giant cells (arrows) (H&E, × 115).
Intracytoplasmic inclusions precede the development of intranuclear inclusions, and they are often more difficult to identify.26 They develop in perinuclear locations, and with enlargement acquire an eosinophilic, hyalinized appearance. Intranuclear inclusions begin as tiny eosinophilic bodies that coalesce to a single, eosinophilic inclusion that occupies more than half the diameter of the nucleus and is surrounded by a inconspicuous halo and minimally marginated chromatin.70 Although multi-nucleated giant cells with inclusions are suggestive of measles, they are not absolutely specific and can be mimicked by other RNA viruses (discussed later). The presence of the measles virus in the multinucleate cells may be demonstrated using immunofluorescence, immunohistochemistry, in situ hybridization, or nucleic acid amplification.71–73
In negatively stained electron microscopic preparations, particles are generally rounded with pleomorphic forms that measure 100 to 300 nm in diameter. Tubular nucleocapsid material may be found in the nucleus or cytoplasm when thin sections are viewed with transmission electron microscopy, and tubules measuring 15 to 20 nm in diameter may be seen (Fig. 9–7).29
Atypical measles is a syndrome that occurs in patients who are exposed to wild measles virus years after immunization with killed measles vaccine, in use from 1962 to 1968.70,74 Fever, myalgias, cough, headache, an atypical rash, and extremely high measles hemagglutination inhibition antibody titers characterize the illness. The chest roentgenograms show patchy alveolar infiltrates with confluence, and these infiltrates may form nodules that simulate metastatic disease. Rapid clinical recovery is the rule, but the radiologic nodules may persist for several months. The histologic appearance of these nodules is presumably nonspecific, but few descriptions of them are available, because awareness of the syndrome obviates biopsy of the lesions. One study of 17 adolescents with atypical measles reported all 17 with peripheral dermatitis and 15 with pulmonary infiltrates; skin biopsies from 3 demonstrated an Arthus reaction.75
FIGURE 9–6 (A,B) Measles multinucleated giant cells with intranuclear inclusions (arrows).
Parainfluenza
Parainfluenza types 1 to 4 are all respiratory pathogens in humans and are second in frequency only to RSV in causing viral respiratory illness in children.33 Parainfluenza infections are most commonly noted in children from 6 months to 5 years of age, but mild upper respiratory tract reinfections occur in older children and adults.76,77 Parainfluenza virus types 1 to 3 are the most common cause of disease, and can be identified in respiratory specimens using direct immunofluorescent antibodies and cell culture.78 Antibody testing is of limited or no value given the ubiquitous nature of this virus. Parainfluenza type 3 produces the most severe disease, which may include bronchiolitis, and, importantly, is the only parainfluenza virus that commonly infects children less than 6 months of age.50 Parainfluenza type 4 is an uncommon cause of human disease and does not produce severe disease, and thus most clinical laboratories do not test for it.79
Parainfluenza viruses most commonly causes laryngotracheobronchitis (croup), and rarely bronchiolitis and pneumonia.35,50 The incidence of respiratory tract infection due to parainfluenza virus is no higher for immunocompromised patients than for persons with intact immune systems, but once infected, immunocompromised hosts have more difficulty limiting infection and destroying the virus.80 Parainfluenza pneumonia may occasionally be fatal, particularly in the immunocompromised.78,81,82
Morphology
The lungs from patients dying from parainfluenza are firm and heavy. Although the consolidation may be diffuse, the lungs may also show nodularity. The histologic findings in fatal cases include diffuse alveolar damage with or without giant cells.81,82 Interstitial pneumonia with multinucleate giant cells containing intracytoplasmic inclusions mimicking measles may also occur.83 Butnor and Sporn82 showed that the giant cells in such cases were reactive with antibodies to cytokeratins (CAM 5.2 and AE1/AE3), but were not decorated by antibodies directed against macrophages (CD68), confirming the epithelial nature of these cells. Parainfluenza type 2 pneumonia in dogs (“kennel cough”) causes denudation of terminal bronchiolar epithelium with a mononuclear cell infiltrate, but minimal parenchymal changes.84 No well-defined inclusions are seen by light microscopy; the ultrastructural characteristics are somewhat similar to measles virus (Figs. 9–7 and 9–8), except that virus particles are found in the cytoplasm and not the nucleus.
FIGURE 9–7 Electron micrograph of intranuclear measles inclusion (×61,700). (Courtesy of Dr. F.E. Cuppage, Kansas University Medical Center.)
Respiratory Syncytial Virus and Human Metapneumovirus
Respiratory syncytial virus (RSV) is the major case of serious lower respiratory tract infections in children under 1 year of age, and particularly prominent in those less than 6 months of age.85 The incidence of RSV infections, like influenza and parainfluenza types 1 and 2, is most common in winter and early spring.
The epidemiologic and immunologic characteristics of RSV are peculiar. Although there is no multiplicity of antigenic types, some have noted that the addition of anti-RSV immunoglobulin to cell cultures containing RSV does not block or prevent infection.86 Similarly, some believe the maternal antibodies have little effect on the incidence or severity of RSV infection in infants, whereas others believe that passive maternal humoral immunity does offer some degree of protection.86–89 Patients with RSV infection may shed the virus for prolonged periods, which can be a particularly serious infection control problem, because this infection may be fatal for children who are hospitalized with other serious illnesses.90 Reinfection is common with RSV, but subsequent infections are usually milder.42,91 Although most adults have antibodies to RSV, RSV may still cause protracted upper respiratory tract infections, especially in adults who work closely with infants during the winter.92,93 Occasionally, the virus may cause life-threatening or fatal pneumonia in adults, particularly the elderly.45,92 The poor correlation between humoral or cellular immunity and the clinical course of RSV infection implies that an as yet uncharacterized local immune mechanism may play a role in the response to infection.94
The clinical and radiologic patterns of RSV-related respiratory tract disease in infants are variable. The most common, and classic, pattern is bronchiolitis (Fig. 9–9). Dyspnea, wheezing, and radiographic evidence of air trapping are characteristic. Other patterns include croup, bronchitis with radiographic bronchial wall thickening and linear perihilar infiltrates, and pneumonia with rales and radiographic consolidation.33,95 Approximately one third of RSV infections are clinically apparent in children aged 0 to 5 years.94 Respiratory disease sufficiently severe to require hospitalization has been estimated at 1 to 2% of infections, and of hospitalized patients, ~1% die.43 In a study of incidence and clinical features of hospitalization secondary to RSV infection in rural Asia, Djelantik et al96 found that 23% of 2677 children hospitalized for lower respiratory tract infection had a positive test for RSV and the case fatality of this subset was 1.9%. Higher fatality rates are seen for infants with severe underlying disease, such as cardiac disease.97 RSV bronchiolitis has been associated with long-term pulmonary function abnormalities86 (see Relationship of Viral Infections to Chronic Airflow Obstruction, below).
FIGURE 9–8 Parainfluenza perinuclear cytoplasmic tubular appearing nucleocapsid material forming an inclusion (×72,009).
FIGURE 9–9 Respiratory syncytial virus (RSV) bronchiolitis. Note conspicuous involvement of bronchi and bronchioles with sparing of alveoli (H&E, × 30).
FIGURE 9–10 RSV bronchiolitis with metaplasia of columnar epithelium and mild intraluminal exudate. Note giant cells (arrows).
Morphology
The lungs of children who die of RSV infection demonstrate areas of hyperinflation that alternate with areas of consolidation and hemorrhage.98 Microscopic findings include plugging of the terminal bronchioles with a mixture of mucus, inflammatory cells, and cellular debris; large syncytial giant cells with globular eosinophilic inclusions are present in and line the alveoli.98 In addition, bronchiolar epithelial cell necrosis, purulent intraluminal exudates, and peribronchiolar mononuclear infiltrates may be seen (Figs. 9–10 and 9–11). RSV may also cause diffuse alveolar damage superimposed on bronchiolitis.99 Correlation with laboratory findings or the use of immunohistochemical or other techniques to confirm the etiology of the giant cell pneumonia is recommended, because these giant cells mimic those present in measles pneumonia (Fig. 9–12).98,100,101
The intracytoplasmic inclusions produced by RSV may not be discernible in all cases, and tend to occur early in the course of the disease.26 When present, the inclusions measure from 1 to 20 μm and are present solely in the cytoplasm, a feature useful for differentiating RSV inclusions from those of measles.98 The inclusions are rounded, eosinophilic, may stain positively with Giemsa stain, and when small may be surrounded by a clear halo. Electron microscopy of thin sections of RSV-infected cells shows an appearance similar to other paramyxoviruses, but the nucleocapsid tubules are slightly smaller (12–15 nm in diameter)29 (Fig. 9–13).
Human metapneumovirus is a newly recognized paramyxovirus of the genus Pneumovirus that has been shown to cause disease in humans.102,103 The clinical disease is similar to that of RSV, but less severe. Infection may occur in any age group, but serologic studies demonstrate that most children have evidence of prior infection by the age of 5 and that the prevalence of disease is high.102,104,105
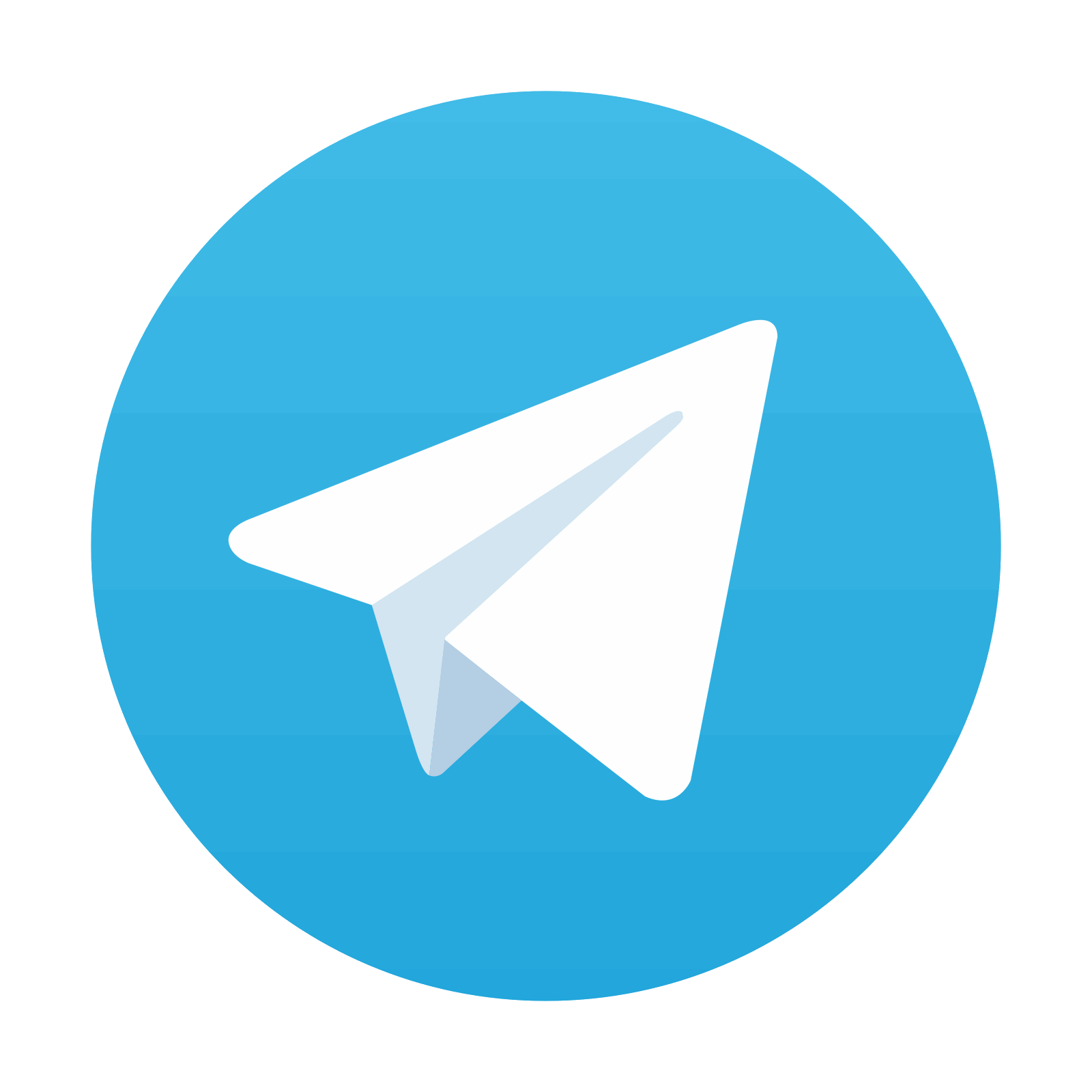
Stay updated, free articles. Join our Telegram channel

Full access? Get Clinical Tree
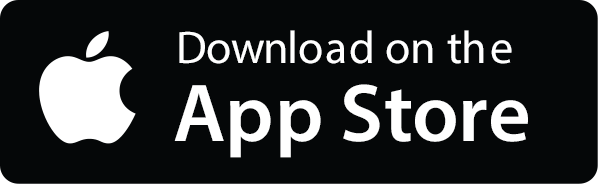
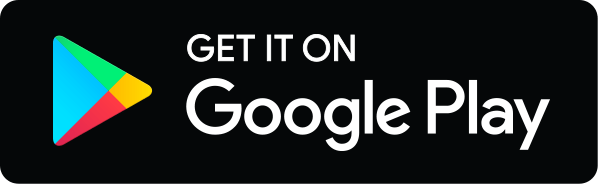