John W. Brown and Jeremy L. Herrmann Riley Hospital for Children, Indiana University School of Medicine, Indianapolis, IN, USA Valve pathology in infants and children poses numerous challenges to the pediatric cardiologist and cardiovascular surgeon. Valve reconstruction should be the goal of surgical intervention, because restoration of anatomy and physiology employs native tissue that allows for growth and potentially better long‐term outcome. When repair fails, or is not feasible, valve replacement becomes ineviTable [1]. The valve pathology, patient size, and requirement for growth all interrelate, making valve replacement in neonates, infants, and children a challenging surgical procedure. The pulmonary valve is the most commonly replaced valve in the congenital heart population. Reconstruction of the right ventricular outflow tract (RVOT) is performed in patients with congenital heart disease when there is discontinuity between the right ventricle (RV) and the branch pulmonary arteries (PA) or in whom significant pulmonary valve stenosis or insufficiency is present. In 1963, Herb Sloan and coworkers inserted a pericardial nonvalved tube as the first right ventricle to pulmonary artery (RV–PA) conduit in a child with common arterial trunk [2]. In 1964, Giancarlo Rastelli and coworkers reported on a pericardial nonvalved tube as the first RV–PA conduit in a child with pulmonary atresia [3]. Ross and Somerville [4] introduced the aortic valved allograft for RVOT reconstruction in 1966. The extracardiac conduit has permitted routine repair of congenital anomalies that involve pulmonary atresia or hypoplasia of the RVOT. Such lesions include complex forms of tetralogy of Fallot (with or without pulmonary atresia and major aortopulmonary collateral arteries [MAPCAs]), common arterial trunk, pulmonary atresia with intact ventricular septum, transposition of the great arteries with ventricular septal defect (VSD) and pulmonary stenosis or atresia, certain subtypes of double‐outlet right ventricle (DORV), as well as pulmonary autograft replacement of the aortic root in the Ross operation. Many of these anomalies are routinely corrected in neonates – the most challenging environment for any prosthesis selected for RVOT reconstruction. The porcine valve Dacron conduit was favored through the 1970s and early 1980s, but late obstructive complications, particularly the development of neointimal peel formation within the Dacron tube graft, led to near abandonment of this prosthesis for RVOT reconstruction [5]. The cryopreserved allograft (aortic and pulmonary) subsequently became the conduit of choice for RVOT reconstruction from the mid‐1980s to the late 1990s. Development of cryopreservation techniques considerably improved the availability and durability of allografts, resulting in increased clinical use. Numerous valved conduits have since been introduced, including aortic xenografts in Dacron tubes, stented bovine pericardial or porcine xenografts in pericardial tubes, glutaraldehyde‐fixed aortic and pulmonary roots, aortic and pulmonary allografts, and bovine jugular vein conduits [6–11]. Stentless valves have gained favor by avoiding the 20–35% reduction in effective orifice area seen with stented bioprosthetic valves. At our institution, more than 1230 patients have undergone a valved RVOT reconstruction in the past 35 years, and a listing of the types of procedures is given in Table 23.1. In this chapter, we will review polytetrafluoroethylene (PTFE) RVOT reconstruction (with or without valves), the various RV–PA conduits, isolated pulmonary valve replacement in older patients including transcatheter pulmonary valves, and finally the indications for intervention and reintervention on the RVOT in older patients who have had previous surgical and nonsurgical intervention. Table 23.1 Summary of right ventricle to pulmonary artery conduit replacement including pulmonary valve replacement at Indiana University from 1980 to 2016. Patients with tetralogy of Fallot with significant pulmonary valve annulus and/or leaflet hypoplasia/dysplasia historically have been treated in one of two ways: transannular patch, or valved conduit insertion. The transannular patch immediately relieves RV hypertension and enhances RV growth disproportionally with patient growth, but reoperation for RVOT stenosis is generally uncommon. The disadvantage of transannular patching is acute pulmonary insufficiency (PI) resulting in sudden hemodynamic conversion of an obstructed pressure‐loaded RV to a volume‐loaded RV, which causes temporary and/or delayed RV dysfunction. Chronic RV volume overload can lead to late biventricular dysfunction and tricuspid insufficiency, necessitating the need for pulmonary valve insertion [12, 13]. Conduit insertion is particularly useful in patients who have peripheral unrepaired pulmonary stenosis or elevated pulmonary vascular resistance (PVR >4 Wood units). The disadvantages of currently available valved conduits are their structural deterioration secondary to calcification, shrinkage, and lack of growth, resulting in early and late valve dysfunction. These unsolved problems are manifested by the allograft conduit (AC) that became popular in the United States during the mid‐1980s. Pulmonary allografts remain popular at present, despite freedom from reoperation of only 50% at 5 years in patients undergoing a non‐Ross RVOT conduit insertion [11, 13]. An attractive alternative strategy is the monocusp RVOT patch reconstruction, which in certain patient populations offers potential advantages over a transannular patch without pulmonary valve reconstruction or placement of a valved conduit. The main advantage of a monocusp valve is the ability to construct the monocusp in patients of any size when the monocusp leaflet is tailor‐made to fit the RVOT that is being enlarged by a transannular outflow patch. A PTFE monocusp is also less expensive and usually lasts longer than a valved conduit in an infant in our experience. The monocusp RVOT patch can be constructed with autologous or bovine pericardium [14, 15], allograft pulmonary valve cusp [16], or a PTFE membrane (0.1 mm PTFE cusp; W.L. Gore, Flagstaff, AR, USA; off‐label use) traditionally employed as a pericardial substitute [17–21]. Each of these materials has demonstrated good immediate postoperative valve competency and reduced early PI [18, 20–22]. In 1993, Yamagishi and Kurosawa [19] and Oku and colleagues [20] independently introduced 0.1 mm PTFE pericardial membrane as a readily available material with good handling characteristics for monocusp valve construction, and found it superior when compared to biologic leaflet materials. Our animal studies suggest that 0.1 mm PTFE functions as well as or better than fresh or glutaraldehyde‐treated pericardium, and we adopted this technique in 1994. Clinical application of this material in our patients with tetralogy of Fallot in whom transannular patching was necessary resulted in improved perioperative RV function, as evidenced by a lower postoperative central venous pressure, less need for postoperative inotropic agents, less chest tube drainage, and decreased intensive care unit (ICU) and hospital stays [23]. The PTFE membrane is now our material of choice for a monocusp construction and has been used in more than 250 patients with a wide range of RVOT obstructive and regurgitation defects. We particularly value its use in the small infant undergoing primary tetralogy of Fallot repair with or without pulmonary atresia in whom transannular patching is necessary. Construction of a pericardial or PTFE monocusp is simple, relatively inexpensive, and reproducible. Allograft pulmonary valve cusps are considerably more expensive in the United States and may be difficult to custom fit into the RVOT. The potential disadvantage of a monocusp patch in comparison with insertion of a pulmonary valve or valved conduit is that PI may recur more quickly in some patients if the monocusp leaflet sticks in an open position, as has been reported with some biologic monocusps [18, 21, 22]. Recurrent stenosis at the level of the monocusp valve is rarely seen with monocusp leaflet material of any type. Another major advantage of the 0.1 mm PTFE is that it does not allow tissue ingrowth and remains mobile (functional) for a much longer period than biologic monocusps. The approach to monocusp insertion at our institution has remained relatively unchanged over the last 20 years. Patients with defects that include an intact pulmonary valve annulus are evaluated following pulmonary valvotomy. The annulus diameter is measured with a Hegar dilator and if the annular z‐value was ≥–2, then transannular patching is not performed. In patients with moderate to severe annular hypoplasia (z‐score <–2), or postvalvotomy RVOT gradient >30 mmHg and/or RV pressure >80% of systemic pressure, transannular patch repair with a monocusp valve insertion is used. The length and width of the monocusp leaflet are determined and the 0.1 mm PTFE membrane is sewn into the RVOT (Figures 23.1–23.3). To ensure monocusp leaflet coaptation to the conal septum and/or residual pulmonary leaflet tissue, the width and length of the patch are kept mildly redundant. A second patch of 0.4 mm PTFE is sewn across the entire RVOT onto the main PA with the second suture line. Figure 23.1 Technique for polytetrafluoroethylene monocusp insertion (monocusp inserted before right ventricular outflow patch). Source: Reproduced by permission from Indiana University School of Medicine. Copyright © 2012 Indiana University Trustees. The PTFE monocusp with separate RVOT patch has been useful for our patients with tetralogy of Fallot or pulmonary atresia with VSD who require a transannular patch (25–30% of our tetralogy series) and in patients requiring a second RVOT procedure after failed initial conduit reconstruction. The PTFE monocusp can be expected to retain adequate function in the early to mid‐postoperative period. The only anticoagulant used is aspirin (10 mg/kg day, up to a limit of 80 mg/day). The monocusp valve has functioned well in most patients for the first 3–4 years and for up to 10–12 years in some patients. While most patients developed some pulmonary regurgitation as the RV outflow tract grows, recurrent outflow obstruction at the leaflet level has not been observed. Another important indication for PTFE monocusp reconstruction is primary AC failure, because inserting a second AC has been associated with accelerated structural failure [13]. In our previously published experience of RVOT reconstruction, 192 patients with a mean age of 3.3 years were analyzed, including 35 who received a PTFE monocusp [24]. Overall freedom from increased PI greater than moderate was 86% at 1 year, 68% at 5 years, and 48% at 10 years, and the overall freedom from reoperation was 96% at 1 year, 89% at 5 years, and 82% at 10 years following RVOT reconstruction with a PTFE monocusp. We recently updated this series to include the past 20 years of experience with PTFE monocusp reconstruction of the RVOT [25]. From 1994 to 2014, 171 patients (mean age 1.5 years) underwent initial PTFE monocusp reconstruction, and 145 (85.3%) completed full follow‐up. The mean follow‐up duration was 10.9 years, and there were 5 late deaths and 1 early death. Of these patients, 42 (24.5%) required replacement of the monocusp at a mean 10.1 years after the initial procedure. The indications for reoperation included right heart failure, moderate or greater tricuspid regurgitation (TR), severe pulmonary stenosis (PS) or pulmonary regurgitation (PR), severely dilated RV (RV end‐diastolic volume/left ventricle [LV] end‐diastolic volume >2 : 1), RV dysfunction, arrhythmias, poor clinical performance, or a combination of any of these. Echocardiographic follow‐up revealed that 74.5% of patients had moderate or greater PI, and 16.6% of patients had moderate or greater PS. When evaluating cardiac magnetic resonance imaging (CMR) parameters of cardiac function and size, only severe PI was associated with reoperation. Based on our experience, we believe the PTFE monocusp is an excellent short‐ and medium‐term option for RVOT reconstruction when the pulmonary valve cannot be preserved. Several variations of PTFE RVOT reconstructions have been introduced, including a bicuspid PTFE valve, conduits, and a folding monocusp PTFE leaflet [23–31]. Most incorporate a 0.1 mm pericardial PTFE membrane that is used “off‐label” for leaflet construction, as already described [23–25]. Early results with these new modifications seem promising, but longer follow‐up will be necessary to evaluate their durability. Quintessenza and colleagues described a bicuspid RVOT outflow reconstruction [26]. Their early results (3 years) with the 0.1 mm PTFE are considerably better than those obtained with the 0.6 mm PTFE followed for up to 8 years. Nunn and colleagues described a folding monocusp using 0.1 mm PTFE and demonstrated less regurgitation across the RVOT at the expense of a higher gradient at mid‐term follow‐up than obtained with a simple horizontal monocusp [27]. Morell and colleagues have described a PTFE tube graft with a bicuspid PTFE valve constructed in the conduit [28]. Their initial results have been satisfactory in spite their short follow‐up (<1 year). Figure 23.2 Lateral view of right ventricular outflow tract with polytetrafluoroethylene monocusp. Inserts show polytetrafluoroethylene monocusp in closed and open positions. Source: Reproduced by permission from Indiana University School of Medicine. Copyright © 2012 Indiana University Trustees. Figure 23.3 Technique for polytetrafluoroethylene monocusp insertion (complete right ventricular outflow tract patch). Source: Reproduced by permission from Indiana University School of Medicine. Copyright © 2012 Indiana University Trustees. Lee and colleagues from South Korea reported mid‐term results of their use of a PTFE bicuspid pulmonary valve construct in patients presenting for pulmonary valve replacement [29]. In their group of 119 patients (mean age 16.9 years), freedom from reoperation was 99.1%, though 6 patients (5%) required percutaneous balloon dilatation. They also observed significant slowing of RV dilatation. A valved PTFE conduit has been developed in Japan as an RVOT reconstruction option, and the experience with this conduit has been reported by the group in Arkansas [30, 31]. In all, 120 implantations were performed with sizes ranging from 12 to 24 mm in patients with a median age of 6.9 years (range 0–36.5 years). Their 5‐year follow‐up results included freedom from reoperation of 92.7%, from reintervention of 83.7%, and from conduit malfunction of 74.8%. Of the 10 conduit reinterventions performed, 6 involved surgical replacement (all with concomitant procedures) and 4 were catheter based. No instances of endocarditis occurred. Cryopreserved pulmonary allografts were the “gold standard” in the United States from the mid‐1980s through the late 1990s [6, 7, 11]. In most centers, pulmonary ACs are favored over aortic allografts as they are less prone to dysfunction and calcification [32]. The higher elastin content of aortic allografts increases their propensity for early dense calcification and adherence to adjacent structures, making conduit removal or revision challenging. In some patients, extracardiac pulmonary ACs developed significant valve regurgitation within months of insertion and developed shrinkage leading to late conduit stenosis. However, pulmonary allograft performance and longevity is significantly improved in the Ross operation, because many of the patients are older and an oversized pulmonary allograft is inserted in an orthotopic fashion, which alleviates the turbulent flow seen in extracardiac valved conduits. The evaluation of AC durability is critical in determining the choice of prosthesis for pulmonary valve replacement. Forbess and colleagues [6] evaluated 185 consecutive pulmonary ACs implanted at a single institution over 14 years in three separate age groups. Their univariate analysis demonstrated that smaller allograft size (<14 mm), younger patients (<1 year), and diagnosis of common arterial trunk were all risk factors for conduit failure, while allograft size was the only predictor of failure in the multivariate analysis. Ross aortic valve replacement (AVR)‐ related AC implants had the best outcome with a 5‐year AC survival of 94%, while non‐Ross AC implants in children >10 years of age had a 5‐year graft survival of 76% [6]. Dearani and colleagues published the largest series of pulmonary valve replacement and RV–PA conduits from the Mayo Clinic [7]. They described 1270 RVOT procedures in 1095 patients undergoing RV–PA conduit or pulmonary valve replacement over a 37‐year period. The operative mortality was 3.7% in the most recent decade. Mean follow‐up for the entire series was 10.9 years, with a maximum of 29 years. Three types of conduits were implanted: 730 porcine‐valved Dacron conduits, 239 homografts, and 126 nonvalved conduits. Risk factors for conduit failure included pulmonary allografts, younger age (<4 years) at initial operation, and smaller conduit size (<19 mm) [7]. Survivorship free of reoperation for conduit failure was 84.1% at 5 years, 55.5% at 10 years, 39.6% at 15 years, and 31.9% at 20 years. In their series comparing the AC with the Dacron tube with a porcine xenograft, they found the AC was less durable. At our institution, 117 non‐Ross patients received cryopreserved ACs (94 pulmonary and 23 aortic) between January 1985 and December 2003 for RVOT construction [33]. Ages ranged from 6 days to 43 years (mean 8 months), and there were 57 children (49%) younger than 12 months of age. There were no device‐related deaths. The overall patient survival was 80% at 15 years. Freedom from AC failure (valve explantation, balloon dilatation) was 60% at 5 years and 43% at 15 years; freedom from AC failure was worse in infants (42% at 5 years and 34% at 15 years). Freedom from AC dysfunction (AC stenosis >40 mmHg and AC insufficiency more than moderate) was 40% at 5 years and 23% at 15 years; freedom from dysfunction was worse in infants (21% at 5 years and 16% at 15 years). Univariate analysis identified younger age, smaller AC, diagnosis of common arterial trunk, and presence of aortic AC as risk factors for AC dysfunction and failure. Multivariate analysis identified smaller AC size and the presence of common arterial trunk as risk factors for AC dysfunction and failure. A comparison of series with early results of AC used for RVOT reconstruction is shown in Table 23.2 [8, 9, 11, 33–40]. One approach for extending allograft durability that has gained increasing clinical interest is decellularization of the allograft to minimize immunologic reaction against the foreign material. One such process currently under investigation is SynerGraft (SG) processing (CryoLife, Kennesaw, GA, USA) [41]. This proprietary processing technique seeks to mitigate the humoral immune response to human leukocyte antigens of the implanted graft. Early clinical experience with implantation of SG allografts demonstrated a reduction or prevention of panel‐reactive antibodies in recipients of SG allografts compared to patients who received standard AC, at least in the short term [41, 42]. The initial experience with the SG allografts has been encouraging. The first multi‐institutional report of 342 patients who received either an SG allograft or standard allograft as part of either a Ross or RVOT reconstruction procedure demonstrated similar rates of survival and adverse events, with a mean follow‐up of 4 years [43]. There was less pulmonary insufficiency with the SG allografts in both procedure groups, and a lower gradient was observed with the SG allografts in the Ross group. Furthermore, when we evaluated the performance of the SG allografts compared to standard allografts in patients undergoing a Ross procedure at our institution, the SG allografts performed as well as the standard allografts at a mean follow‐up of 4.9 years [44]. With the most recent mid‐ to long‐term results (mean follow‐up 5 years) from this study, no difference in survival between groups was observed, but the freedom from conduit dysfunction was significantly lower in the standard group (58%) than the SG allograft group (83%) [45]. Similarly, 10‐year follow‐up data from the SynerGraft FDA Post‐Clearance Study revealed a 10‐year patient survival of 97%, freedom from reoperation/reintervention of 81%, freedom from any adverse event of 75%, mean peak allograft gradient of 21.4 ± 11.5 mmHg, mean allograft gradient of 12.1 ± 6.5 mmHg, and moderate pulmonary insufficiency or less in 94% of enrolled patients (unpublished data). Based on these ongoing studies, the additional expense of the SG preparation may well translate into improved long‐term valve durability and freedom from reintervention. Table 23.2 Early results of surgical treatment in patients with right ventricular outflow tract allograft implantation. NA, not available. Source: Adapted from Brown JW et al. Ann Thorac Surg. 2005;80:655–664. Aortic allografts calcify more quickly than pulmonary ACs when placed in the RVOT, possibly due to the higher elastin content of the aortic AC wall [9, 11, 12]. The AC size has been found to be a significant mechanical risk factor that influences durability (particularly late stenosis) in many studies [6, 9, 46]. This is especially true in the pediatric Ross AVR population, in that children younger than 5 years of age have accelerated growth and can quickly outgrow their valve conduit. For this reason, we aggressively oversize the implanted pulmonary allograft in Ross AVR patients, regardless of the patients’ pulmonary annulus size, because size rather than patient age is the most important predictor for pulmonary allograft failure (defined as the need for graft replacement) [46]. Table 23.2 provides a summary of reported series of RVOT allograft implantation. Between June 1993 and May 2007, 183 consecutive patients at our institution underwent Ross AVR with RVOT reconstruction using a cryopreserved pulmonary allograft (n = 156), decellularized pulmonary homograft (n = 22), and bovine jugular vein conduit (n = 5) [47]. Ages ranged between 1 month and 61 years (mean age 23.3 ± 15.2 years). There were three deaths (2%), two early and one late. Twenty‐four patients (13%) had a peak systolic RVOT gradient exceeding 20 mmHg, 5 (3%) had a gradient exceeding 40 mmHg, and 7 (4%) had more than 2+ PI. Eight patients (4%) underwent conduit replacement for RV dysfunction. Freedom from RVOT reoperation at 10 years was 96%. Freedom from RV conduit failure and dysfunction was 98% and 96% at 5 years and 96% and 93% at 10 years, respectively. Independent predictors of pulmonary allograft RV–PA conduit dysfunction were smaller (<14 mm) RVOT conduit size (p = .03) and follow‐up exceeding 5 years (p = .05. In our experience the durability of RVOT reconstruction with a pulmonary AC in patients undergoing the Ross AVR was improved secondary to older age at implant, orthotopic positioning, and the opportunity to oversize the AC [47]. Since 1993 at our institution, pulmonary allografts have been the RV–PA conduit of our choice for RVOT reconstruction in the Ross procedure, except for children younger than 6 years of age, where the Contegra® conduit (Medtronic, Minneapolis, MN, USA) is favored. Since 2008 we have adopted the decellularized pulmonary allograft, because reports have demonstrated that they compare favorably to standard cryopreserved allografts [43, 44]. During the last two decades, demand has increased for an RV–PA conduit more durable than ACs for non‐Ross RVOT reconstruction. In late 1999, we began implanting the bovine jugular vein conduit (Contegra BJVC), porcine stentless aortic root xenograft (Freestyle™, Medtronic), and the newer decellularized pulmonary allograft (SynerGraft®, CyroLife, Kennesaw, GA, USA) for non‐Ross RVOT reconstruction [48–50]. The limited availability and durability of ACs, especially in smaller sizes, drove the search for an alternative prosthesis to reconstruct the RVOT in young patients with congenital heart disease. In 1999, the Contegra BJVC was introduced as a potential alternative to allografts for RVOT reconstruction. The BJVC is a low‐pressure, buffered, glutaraldehyde‐fixed section of bovine jugular vein (12–15 cm in length and 12– 22 mm in diameter). The three‐cusp, naturally occurring venous valves are in the center of the conduit, which possesses natural sinuses. The conduit wall is compliant with excellent suturability. Early animal studies by Ichikawa and colleagues [51] and by our group [52] reported excellent results demonstrating good leaflet preservation and freedom from significant structural degeneration and valve regurgitation at 3 years. Herijgers and colleagues reported resistance of the Contegra BJVC to calcification when implanted in juvenile sheep, albeit for only 5 months [53]. These animal studies were confirmed in human clinical trials with up to 27 months (mean 1.2 years) of follow‐up reported by Breymann and colleagues [54], suggesting that the Contegra BJVC might represent a promising alternative to allografts. A report by Bové and colleagues comparing modified bicuspid pulmonary allografts and Contegra BJVC supported these findings in 41 patients (mean age 1.9 years) undergoing RVOT reconstruction [55]. The BJVC demonstrated favorable hemodynamics compared to allografts. Other advantages of the Contegra BJVC include greater shelf availability, natural continuity between valve and conduit, and no requirement for proximal extension given its natural length. The principles of Contegra BJVC insertion include rinsing the conduit for 5 minutes with continuous manual agitation in four 500 mL baths of normal saline or Ringer’s lactate before implantation; and removing most of the distal conduit tissue and positioning the conduit valve at the junction of the main and branch PAs to avoid sternal compression [8]. The inflow end of the conduit is spatulated and sewn with continuous monofilament suture to the edges of the right ventriculotomy, without the use of additional prosthetic material. The mid‐term results of RVOT reconstruction using the Contegra BJVC in 153 patients at our institution were retrospectively analyzed [56]
CHAPTER 23
Ventricular to Pulmonary Artery Conduits
Type
No. of patients
Polytetrafluoroethylene monocusp
259
Nonvalved
30
Aortic allografts
27
Pulmonary allografts (decellularized/SynerGraft)
426 (260)
Stented porcine (Hancock)
47
Stentless porcine (Freestyle)
103
Mechanical valve
3
Bovine jugular vein (Contegra)
301
Stented bovine pericardial
34
Total
1230
Polytetrafluoroethylene Ventricular Outflow Tract Reconstruction
Allograft Conduits
Freedom from allograft conduit failure
No. of patients
Early death
Late death
Follow‐up (mean)
Explant Conduit
Freedom from allograft conduit dysfunction
Hawkins 1992 (Cincinnati, OH, USA) [34]
89 (non‐Ross)
7 (8%)
9 (11%)
31 mo
8 (10%)
–
Bando 1995 (Mayo, Rochester, MN, USA) [11]
326 (non‐Ross)
22 (6%)
24 (7%)
3.2 yr
32 (11%)
–
Yankah 1995 (Berlin, Germany) [35]
53 (non‐Ross)
15 (28%)
1 (3%)
26 mo
5 (13%)
–
Niwaya 1999 (Oklahoma, OK, USA) [36]
369 (all)
38 (10%)
13 (4%)
3.8 yr
30 (9%)
62% at 8 yr
53% at 8 yr
Tweddell 2000 (Milwaukee, WI, USA) [9]
178 (all)
22 (11%)
6 (4%)
3.6 yr
42 (27%)
54% at 10 yr
22% at 10 yr
Bielefeld 2001 (Denver, CO, USA) [37]
223 (non‐Ross)
31 (14%)
3 (9%)
6.0 yr
38 (20%)
–
Sinzobahamvya 2001 (St. Augustin, Germany) [8]
76 (non‐Ross)
7 (9%)
3 (4%)
54 mo
14 (20%)
65% at 10 yr
10% at 10 yr
Gerenstein 2001 (Rotterdam, Netherlands) [39]
297 (all)
12 (4%)
15 (5%)
4 yr
24 (8%)
–
Brown 2005 (Indianapolis, IN, USA) [33]
117 (non‐Ross)
16 (14%)
7 (6%)
6.1 yr
43 (46%)
43% at 15 yr
23% at 15 yr
Askovich 2007 (Salt Lake City, UT, USA) [38]
140 (all)
NA
NA
5.1 yr
77 (64%)
–
Boethig 2007 (Hanover, Germany) [40]
79 (non‐Ross)
NA
14 (18%)
6.6 yr
27 (34%)
–
Pulmonary Allografts in Ross Aortic Valve Replacement Patients
Bovine Jugular Venous Valved Conduit
Stay updated, free articles. Join our Telegram channel

Full access? Get Clinical Tree
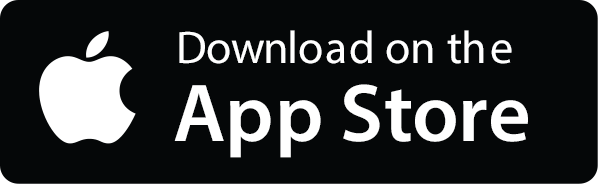
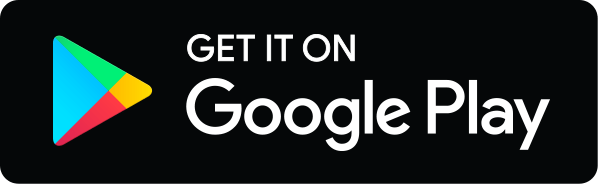