In the absence of heart disease, premature ventricular complexes (PVCs) are generally benign and do not clearly portend an increased risk of sudden or cardiac death, regardless of their frequency (>1 per minute) or pattern (e.g., couplets). Frequent PVCs are relatively common in an apparently healthy population (prevalence 1–4% at a mean age of ~50).1,2,3 Benign PVCs generally occur at rest and improve with exercise; yet some idiopathic PVCs are triggered by exercise. Very frequent PVCs (>5–10% burden) warrant echo + stress test +/- MRI to assess for heart disease, particularly if induced by exercise or recovery. Even in the absence of underlying heart disease, very frequent PVCs may lead to a reversible PVC-mediated cardiomyopathy: in fact, among patients referred for PVC ablation, ~10–30% of those with PVC burden > 10% have reduced EF; EF typically normalizes with PVC ablation.4–8 In patients with heart disease, frequent or complex PVCs or non-sustained VT portend an increased mortality independently of other baseline variables, according to most post-MI studies. However, targeted therapy of PVCs or non-sustained VT is not indicated. In fact, the use of class I antiarrhythmic drugs to suppress PVCs worsens outcomes (as a result of the proarrhythmic side effects of these drugs) and should be avoided in patients with underlying heart disease (CAST trial: in patients with a history of MI and normal or low EF, PVC suppression with class I antiarrhythmics increases mortality).9 β-Blockers and verapamil are the safest treatment for symptomatic idiopathic PVCs but have reduced efficacy (10–24%) when PVCs are very frequent.8 PVCs that originate from the same focus have a monomorphic appearance and may be treated with catheter ablation. In the particular case of frequent PVCs (>5–10% burden on 24-hour Holter) with LV systolic dysfunction, there are three possible diagnoses: (1) ischemia causing both PVCs and LV dysfunction; (2) LV dysfunction causing PVCs; (3) frequent PVCs leading to a PVC-mediated or PVC-aggravated cardiomyopathy. Once ischemia is ruled out, it is reasonable to suppress PVCs with amiodarone or catheter ablation and see if EF improves. In fact, EF substantially improves in over 80% of these patients.4–8 Figure 9.1 Wide premature complexes occurring in a trigeminal pattern. These are typically PVCs but could be PACs with bundle branch block (aberrancy). They fall after normally occurring, non-premature P waves with a shorter PR interval than the sinus beats, which is typical of PVCs. Sinus P waves keep marching out through the PVCs. Figure 9.2 Interpolated PVC. Unlike the common PVC, an interpolated PVC does not prevent the conduction of the preceding or following sinus P wave (arrows) and there is no pause. It is mainly seen when the sinus rate is slow. Instead of being blocked, the P wave that follows the PVC is conducted with a longer PR interval (double arrows). In contrast to ventricular parasystole, typical PVCs are due to a reentrant circuit that is initiated by the supraventricular rhythm, and thus show fixed-interval coupling. Also, by definition, the ventricular rate must be > 100 bpm. A ventricular rhythm slower than 100 bpm is accelerated idioventricular rhythm (AIVR); a ventricular rhythm slower than 40 bpm is a ventricular escape rhythm. Sustained VT is typically secondary to an underlying heart disease: Like sustained VT, non-sustained VT typically occurs in patients with underlying heart disease; however, it occurs occasionally in healthy individuals, more so than sustained VT (prevalence of NSVT in the healthy population, ~1%).1 Approximately 1% of asymptomatic individuals without apparent heart disease develop NSVT during exercise testing; in the absence of ST changes on the sinus beats, NSVT does not affect long-term prognosis and is typically a form of monomorphic idiopathic VT.1,12 NSVT is likely an independent predictor of mortality in patients with cardiomyopathy. In the SCD-HeFT trial of ischemic and non- ischemic cardiomyopathies with EF < 35%, and in the MERLIN-TIMI trial of ACS with predominantly preserved EF, NSVT was independently associated with increased mortality.13,14 PROCANO study randomized patients with ongoing stable VT to acute procainamide vs acute amiodarone. VT terminated within 40 min in 67% of procainamide vs 38% of amiodarone patients. Most patients had structural heart disease and mean EF was 40%; despite that, procainamide was associated with less hypotension and pulmonary edema than amiodarone.15 As a result, procainamide is preferred over amiodarone for acute VT in ACC guidelines 2017 (class IIa for procainamide, IIb for amiodarone).11 Figure 9.3 Tachycardia that seems narrow but has a different morphology than the native QRS and is associated with secondary ST/T abnormalities → VT or SVT with aberrancy. To differentiate, see how the tachycardia starts. It does not start after a premature P wave, but rather starts after a regularly occurring sinus P wave (third arrow) with a shorter PR interval: this means it starts with a PVC, and has the same morphology as this PVC → the tachycardia is VT. Also, one can regularly march out sinus P waves scattered within the tachycardia and dissociated from the QRS complexes, which is diagnostic of VT. These P waves are marked by arrows. One P wave falls within a QRS (marked by an interrupted line). No acute therapy is indicated. Underlying heart disease is sought (echo, stress testing). Long-term treatment: NSVT does not dictate ICD by itself. ICD is dictated by the underlying cardiomyopathy (EF ≤ 35%). AIVR is defined as a slow VT that has a rate of 60–120 bpm. It usually competes with the underlying sinus rhythm with alternation of ventricular and sinus-originated complexes (Figure 9.4). AIVR may be seen in: (1) acute ischemia (including STEMI, whether reperfused or not), (2) up to 8% of HF cases and cardiomyopathies (including hypertensive or valvular cardiomyopathy), (3) digoxin or antiarrhythmic drug toxicity, and (4) electrolyte abnormalities (hyperkalemia). It may also be seen in patients with sinus node disease, where AIVR is a form of accelerated ventricular escape. Treatment: there is no convincing evidence linking AIVR to VT/VF, and thus no specific treatment for AIVR is necessary. AIVR suppression is not indicated, particularly when AIVR is thought to be an escape rhythm. Only in symptomatic patients with sinus node disease and coexisting pauses or chronotropic incompetence is pacemaker therapy indicated. Rule out the following diseases by ECG, exercise ECG, family history, and cardiac MRI: If none of the above diagnoses is evident, and if VT is monomorphic, the patient is diagnosed with idiopathic VT and is treated with β-blockers or CCBs (idiopathic VT is the only VT in which CCBs are effective). Alternatively, the ventricular focus may be ablated. Figure 9.4 Wide complex rhythm at a rate of 70 bpm. P waves are initially seen after every QRS (blue arrows). This could be consistent with a ventricular rhythm with 1:1 VA conduction. However, further down the strip, those P waves start coming sooner and fall onto the QRS complexes (two complexes under the bar), then appear before the QRS complexes and get conducted (black arrows). Thus, these P waves are actually sinus P waves dissociated from the ventricular rhythm. This dissociation is an isorhythmic AV dissociation, wherein the ventricular and sinus rhythms have close rates: when the sinus rhythm speeds up, it takes over and P waves get conducted. When it slightly slows down, the ventricular rhythm takes over. This is AIVR in a patient with anterolateral STEMI. While classically seen after reperfusion, it may very well be seen in non-reperfused infarcts such as this one. ST elevation is seen on both the ventricular and sinus-originating complexes. ST elevation that is concordant to QRS is indicative of STEMI, even in ventricular complexes. Polymorphic VT is a VT that has two or more QRS morphologies: Acutely, polymorphic VT is treated similarly to any sustained VT. The underlying metabolic abnormalities are treated, and urgent ischemic evaluation and revascularization are performed. As opposed to TdP, amiodarone may be used; quinidine is used for pseudo-TdP. There are two types of TdP: Figure 9.5 Torsades de pointes initiation, via short-long-short sequence: (1) PVC, (2) long post-PVC pause which creates a long and dispersed refractory period, (3) another PVC hits this dispersed refractory period, leading to TdP. The PVCs, per se, arise from early afterdepolarizations. Figure 9.6 QT interval is markedly prolonged (QTc = 730 ms). T wave is wide and ample in lead V3, is deeply inverted in the inferior leads, and demonstrates beat-to-beat alternation in morphology and amplitude, the so-called macroscopic T- wave alternans. In addition, alternation in T-wave polarity is seen in lead V5. T-wave alternans may be seen with any cause of prolonged QT and implies severe heterogeneity of ventricular repolarization and an imminent risk of TdP; it is more characteristically seen in congenital long QT syndromes. While this patient has hypokalemia, the wide and ample T-wave morphology without ST depression is not consistent with hypokalemia. The shape is consistent with long QT syndrome or ischemia. Arrows of two different lengths point to the two different T-wave morphologies. Figure 9.7 Torsades de pointes that degenerates into VF (same patient as Figure 9.6) Figure 9.8 Example of TdP in a patient whose baseline QTc is 530 ms. Note the twisting QRS polarity. TdP is initiated by a short-long-short sequence (double-arrows) As opposed to a steady-state bradycardia, acute bradycardia that occurs after a period of tachycardia is associated with a more striking prolongation of repolarization that facilitates the induction of polymorphic VT.23 For instance, this is seen when AV nodal ablation is performed in patients with AF. As a result, the latter patients are paced at a rate of 80–90 bpm for ~1 week to prevent VT. Acutely, three lines of therapies are applied: Pacing may not be effective for the patient in Figure 9.6 because he is tachycardic. Congenital long QT’s torsades, as opposed to acquired long QT, are often triggered by a catecholamine surge and may be associated with sinus tachycardia; in fact, acute IV β-blockade is used to prevent the TdP runs.24 In addition, offending agents are withheld and K is corrected to 4.5–5.0 mEq/l. The most common LQT syndromes are LQT1 (~35%), LQT2 (25%), and LQT3 (~5–10%). LQT1 and 2 are related to loss of function of K channels (IKs and IKr respectively), leading to prolongation of repolarization with a broad and long T wave in LQT1, and a broad and notched T wave in LQT2 (Figure 9.9).25 LQT3 is related to a gain of function of Na channels, leading to increased depolarization and thus prolongation of the plateau phase of repolarization, i.e., prolongation of the ST segment with a normal-width T wave. The prolongation of repolarization (QT) elicits two untoward processes: (i) afterdepolarizations, and (ii) increased transmural dispersion of repolarization, which allows afterdepolarizations to propagate and sustain reentry and arrhythmias. In LQT1 and 2, TdP is triggered by catecholaminergic activity, which increases the amplitude of early afterdepolarizations. In particular, swimming or physical or emotional stress may trigger TdP in LQT1. Sudden noise or emotion as well as postpartum may trigger TdP in LQT2 (not exercise), whereas sleep may trigger TdP in LQT3. LQT is mainly autosomal dominant with variable penetrance (Romano–Ward syndrome). A prolonged QT is defined as a QTc > 460 ms in women and > 450 ms in men. In the absence of reversible metabolic, drug, or ischemic causes, LQT syndrome is suspected, particularly if QTc > 480 ms. Up to 10% of normal individuals have QTc that is mildly prolonged (up to 480 ms), and 10–15% of patients with long QT syndrome have normal baseline QTc (mainly 400–460 ms).26 Furthermore, 24-hour ambulatory monitoring of QTc has shown that the QTc of healthy individuals varies throughout the day by an average of 76 ms and may reach 480–490 ms at night or in the early morning.27 In patients with QTc prolongation, rule out the transient causes (e.g., drugs) and perform an echo to rule out underlying cardiomyo- pathy (any cardiomyopathy may prolong QT). If QTc > 480–500 ms without any reversible cause, the diagnosis of LQT is virtually established. In patients with borderline QTc prolongation of 450–480 ms, further testing may need to be performed, particularly in case of syncope or family history of sudden death: further prolongation of QTc with exercise testing (measurement performed a few minutes into recovery, as the heart rate slows down), epinephrine infusion, Valsalva, immediate standing, or post-PVC implies LQT1. QTc >445–470 ms at 2–4 min of recovery is predictive of long QT syndrome.11 In normal individuals, QTc is reduced with exercise or epinephrine infusion.28 In addition, the presence of macroscopic T wave alternans supports the diagnosis of LQT. Other supportive features in case of borderline QTc prolongation: syncope, family history of LQT or sudden death, familial screening with ECG (marked prolongation of QTc in a family member may confirm the diagnosis in the index patient). Figure 9.9 Typical ST–T morphologies in hypokalemia, hypocalcemia, and congenital long QT syndromes (LQT). In hypokalemia, ST segment is depressed and U wave is large while T wave is flattened. In hypocalcemia and LQT3, QT interval is prolonged as a result of ST-segment prolongation and, as opposed to other congenital LQT or QT prolongation secondary to drugs, there is no significant widening of the T wave. In LQT1, T wave is wide and ample without ST-segment depression, similar to Figure 9.5. In LQT2, T wave is wide and notched (double hump). LQT1 may have a morphology similar to LQT3 and is actually the most common LQT with this morphology. In all long QT cases, particularly congenital LQT, the T wave may become notched after a pause, the notch representing the early afterdepolarization (EAD) wave that triggers TdP. QT interval is best measured in the lead that shows a distinct T-wave termination with the best separation of T and U waves.29 The QT interval may be artificially shortened in some leads, because an isoelectric segment may be recorded at the beginning of the QRS complex or at the end of the T wave in those leads. On the other hand, QT is often longest in leads V2–V3, but those leads show the least separation between the T wave and the normal U wave and may therefore overestimate the length of the QT interval. Leads I, aVR, and aVL do not have the normal diastolic U wave but do not always show a distinct T wave. Thus, QT interval is often best measured in leads II and V5 or V6. A normal U wave (<25% of T wave) or an abnormal U wave that is not merging with the T wave should not be used in QT calculation. In practice, a wave that exceeds 25% of the T wave and that merges with the T wave giving a “bifid” T-wave appearance should be taken into account; this is a part of the T wave (TU wave) rather than a true diastolic U wave. QT interval shortens with increasing heart rate (17.5 ms for every 10 beats). The corrected QT corresponds to the patient’s predicted QT interval had the heart rate been 60 bpm; it is often calculated using the Hodges formula: QTc = QT + 1.75 × (rate – 60). The long QT syndrome phenotype is more common in women than men (~1.5:1). Sudden death and syncope mainly occur in childhood or early adulthood before the age of 40 (Table 9.1). QTc > 500 ms, female sex with LQT2, or male sex with LQT3 confer the highest lifetime risk.30 Female sex confers a higher risk of sudden death in adulthood, including LQT1, whereas male sex is a risk factor for sudden death in childhood.31 Overall, ~ 50% of LQT syndrome patients experience TdP with syncope or sudden death during their lifetime. Table 9.1 Risk of sudden death and cardiac events in patients with long QT syndrome before the age of 40. Data from Priori et al. (2003).30 a Same risk in men and women in reference 30, higher risk in women in reference 31, where only adult patients were considered. QTc shortens with adult age in men (testosterone effect), and adult men are thus at lower risk of sudden death than women. Overall, the yearly risk of sudden death averages < 0.5–1% per year. Patients with long QT syndrome genotype but normal phenotype (normal QTc) have a real risk of sudden death, albeit smaller than patients with prolonged QTc, and should be treated with β-blockers. While the risk of sudden death attributed to long QT syndrome is highest in the first 40 years of life, this syndrome remains associated with a 2.65-fold increased risk of sudden death in patients 41–60 years of age, particularly with LQT2 or 3, according to an LQT registry. The risk beyond the age of 60 is attenuated, partly because the risk of death from other illnesses increases at this stage.32 Genetic testing is negative in 20–25% of patients with LQT1–3, because of unidentified mutations of identified genes. Thus, testing is not useful to rule out the diagnosis, but may rule it in when positive in a patient with borderline clinical criteria. Testing is useful for two more reasons: (i) identify the type of LQT, which has prognostic implications; (ii) once a mutation is identified in a proband, family members are checked for that same mutation with a very high rule-out yield. The mainstay of therapy of congenital LQT1 is β-blockade, the efficacy of which is assessed by blunting of the exertional heart rate and mild reduction of QTc (~20 ms). β-blockers should be prescribed to all patients, including silent carriers. β-blockade reduces the risk of cardiac events by ~70% in LQT1 and LQT2, but is less effective in LQT3, especially men with LQT3.33,34 In patients with prior syncope, β-blockers reduce the 5-year risk of recurrent syncope to 30%, and the 5-year risk of sudden death from long QTc to 3%.34 In addition to β-blockade, long-term therapy of symptomatic or asymptomatic congenital LQT includes the maintenance of a normal electrolyte balance, avoidance of drugs that prolong the QT interval, and restriction from participation in athletic activities. LQT type is identified by genetic testing or by the effect of exercise or epinephrine on the QTc (in LQT1, QTc prolongs with exercise or epinephrine, while in LQT2 or 3, QTc shortens). Mexiletine, a class Ib antiarrhythmic, shortens QT and may be considered in all types of LQT when β-blocker is ineffective, particularly LQT3. Left cardiac sympathetic denervation, consisting of removing the left paravertebral stellate ganglion, may also be used for recurrent cardiac events despite β-blockade.11 ICD is indicated in patients with LQT syndrome and prior cardiac arrest (class I indication). In patients with syncope, β-blockade therapy may be enough, with the use of ICD if syncope recurs. Note that TdP is self-terminating, so ICD may not be very helpful in patients with syncope; in fact, the ICD shock triggers anxiety and further catecholamine surge, which may provoke more TdP and VF. Hence, ACC gives a class I ICD recommendation for syncope only in case of recurrence despite β-blockade, intolerance to β-blockade, or other high-risk features (QTc >500, LQT 2-3, age <40).11 ICD implantation may be considered in asymptomatic patients with the highest risk of sudden death, such as those with QTc > 500 ms, or LQT 2 (women) or 3, the latter two being less responsive to β-blockade and having a high residual risk of cardiac events despite β-blockade (class IIb recommendation). Women with LQT2 have a particularly high risk of sudden death in the 9 months post-partum, 7 times higher than their pre-pregnancy and pregnancy risks, and may receive Lifevest during that period, if not ICD.11,35 ICD is indicated for any sustained VT, regardless of EF (AVID, CIDS trials),36,37 except: VT occurring in a patient with CAD but without acute MI or with only low-level troponin elevation qualifies for ICD regardless of EF and of the potential for complete revascularization.38 VT that is monomorphic, or even polymorphic with no acute MI, usually signals an underlying scar and its inducibility is unaffected by revascularization.39 According to the ACC guidelines: “In patients with ischemic heart disease and sustained monomorphic VT, coronary revascularization alone is an ineffective therapy to prevent recurrent VT.”11 It is indicated in ischemic or non-ischemic cardiomyopathy if EF is ≤ 35% and the patient is symptomatic, NYHA functional class II or III, despite optimal medical therapy (ACC class I recommendation). The following waiting periods are required before implanting the ICD: Note that, in ischemic cardiomyopathy with EF ≤ 30%, ICD is indicated even if the functional class is I, i.e., if the patient is asymptomatic (class I recommendation). Also, in ischemic cardiomyopathy, ICD is indicated if EF is 36–40%, NSVT is present, and VT is inducible on EP study (class I recommendation). Those recommendations are based on the MADIT II and SCD-HeFT trials*.20,40 The absolute yearly reduction in mortality was 3.5% in MADIT II, and 2% in SCD-HeFT. In these two trials, the survival curves of ICD therapy and placebo progressively diverged with time; how- ever, in both trials, the curves did not begin to diverge until 12 months, indicating that the benefit from ICD in primary prevention is a long-term benefit that is seen in patients healthy enough to survive several years. The yearly number needed to treat (NNT) to prevent one death is 25 in the MADIT II trial. While the risk of sudden death is highest in the first 40 days after MI, ICD implantation is not beneficial during this time frame. One hypothesis is that, during this early period, patients at highest risk of VT/VF are patients with large infarctions and severe heart failure who may eventually succumb to pump failure even if resuscitated from VF (DINAMIT, IRIS trials).41,42 Thus, ICD simply converts arrhythmic death into pump failure death or pulseless electrical activity (PEA) in many of those patients and does not prevent the eventual mortality. Also, the untoward effects of the periprocedural stress of ICD implantation may hinder the benefit. Building on this data, an analysis of the MADIT II trial has suggested that the benefit of ICD is mainly seen in patients whose MI is older than 1.5 years; while sudden death is highest in the early MI period, pump failure death, HF events, and recurrent acute coronary events are also highest during this period, reducing the effect of ICD on the overall mortality.43 ICD is mainly effective in patients who have been stable for a prolonged duration after their MI, wherein the risk of isolated arrhythmic death from the arrhythmogenic scar becomes a relatively bigger concern.
9
Ventricular Arrhythmias: Types and Management, Sudden Cardiac Death
I. Premature ventricular complexes
i) right vs. left:
right ventricular origin → LBBB morphology
left ventricular origin → RBBB morphology
ii) anterior vs. inferior:
anterior origin→QRS (+) in the inferior leads (vertical axis)
inferior origin → QRS (–) in the inferior leads (left axis)
iii) basal vs. apical:
basal origin → QRS complex is upright in all precordial leads, and (–) in I-aVL if lateral origin
apical origin → QRS complex is negative in all precordial leads, as it looks away from them, particularly V3–V6
II. Ventricular tachycardia (VT)
A. Types (Figure 9.3)
B. Causes of sustained VT
C. Causes and prognosis of non-sustained VT
D. Acute management of sustained VT
E. Chronic management of sustained VT
F. NSVT management
G. Accelerated idioventricular rhythm (AIVR)
H. Workup of VT occurring without obvious heart disease on echo and coronary angiography (see Sections VI-VIII)
III. Polymorphic ventricular tachycardia
A. Morphology and types
B. Treatment
Long-term treatment:
IV. Congenital long QT syndrome (LQT)
A. Types
B. Problems with the definition of long QT and with the diagnosis
C. Epidemiology and risk of sudden death
LQT1
LQT2 or LQT3
Risk of sudden death before the age of 40
10%
Same risk in men and womena
20%
Highest risk if woman LQT2 or man LQT3 (~25%)
Risk of sudden death or syncope before the age of 40
Highest risk when QTc > 500 ms, regardless of LQT type (70 % risk)
30%
~50%
D. Genetic testing
E. Therapy
V. Indications for ICD implantation
A. Secondary prevention
B. Primary prevention means ICD implantation in a patient without a history of sustained VT or sudden death
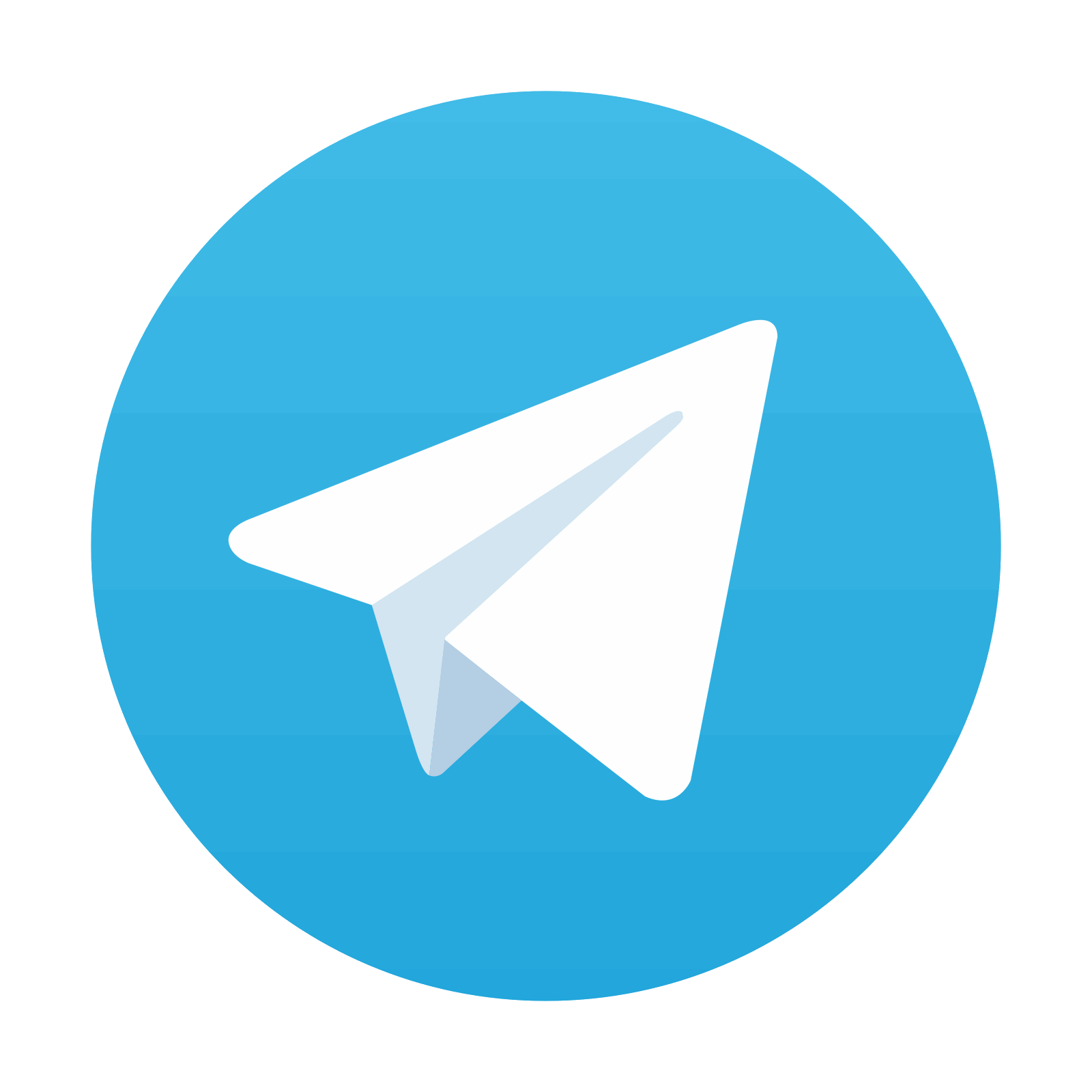
Stay updated, free articles. Join our Telegram channel

Full access? Get Clinical Tree
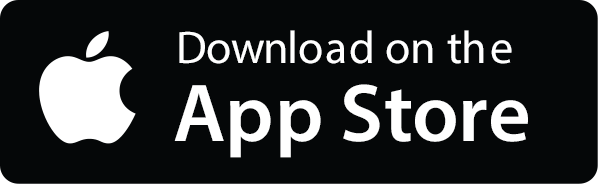
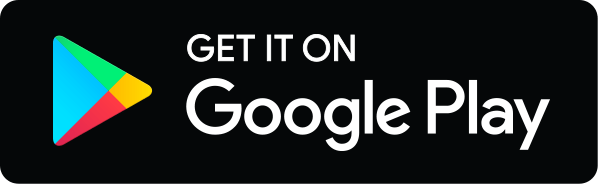