Keywords
Single ventricle heartHypoplastic left heart syndromeTricuspid atresiaDuct-dependent diseaseBidirectional Glenn shuntFontan circulationHemi-FontanFontan repairFontan paradoxA group of congenital heart malformations exist that present with markedly decreased blood flow to the lung. They are invariably associated with incomplete development of the right or left heart complex. Historically, the notion that a single, often weakened ventricle could “pump” the blood through pulmonary and systemic circulations went beyond expectation of experimentalists and clinicians. Yet, in spite of over four decades of clinical experience with patients with Fontan circulation “the adaptive mechanisms allowing such extreme pathophysiology to stably sustain the circulation for decades are still poorly understood” [1] and present a compelling argument in support of the new circulation model.
20.1 Cavopulmonary Bypass
While it has been recognized for some time that a significant damage to the left ventricular wall results in acute pulmonary congestion due to backing-up of the blood before the “pump,” a controversy reined in the 1940s, whether the failing right ventricle (RV) is the actual cause of the peripheral venous congestion? To clarify the issue, Starr and coworkers conducted experiments on dogs in which the RV free wall was destroyed by cautery. Surprisingly, this radical intervention caused relatively minimal symptoms save for an increase in the central venous pressure (CVP) [2]. Similar experiments by others [3, 4], and those in which parts of the RV were excised and replaced by prosthetic material, were tolerated without apparent long-term harm to the experimental animals [5]. The conclusion was therefore drawn that a mere increase in CVP is sufficient to propel the blood through the low resistance pulmonary circulation. Since the RV depends for most of its pumping power on synergistic action of the interventricular septum, it serves merely as a “passive conduit for blood” [6] and can be considered “dispensable” [7].
A more dramatic, but nevertheless survivable clinical scenario transpired when an attempt was made to surgically bypass the RV. Rodbard and Wagner were the first to publish a study on dogs in which the pulmonary artery was occluded at its exit from the right ventricle and connected directly to the atrial appendage, bypassing the right heart. They noted that increased venous pressure was adequate to “drive venous return through the lungs without benefit of the pumping action of the right ventricle.” The dogs were observed up to 2 months without significant side effects [8]. Glenn and coworkers performed a series of experiments with cavopulmonary anastomosis on dogs and were one of the first to report a successful outcome after surgical palliation on a 7-year-old boy with transposition of the great arteries [9]. In the following years, cavopulmonary anastomosis (Glenn shunt) became a standard operation for a number of conditions associated with decreased pulmonary flow. By mid 1970s, Glenn’s group operated on a total of 63 patients with severe malformations of the right heart, including the tricuspid atresia, with “gratifying” results for up to 7 years, only to be followed by signs of cyanosis and hypoxemia [10]. (For review of history of cavopulmonary shunt, see Ref. [11].)
Of note is the fact that complete bypass of the right heart was pursued by several groups at the time, but was fraught with failures in animals and yielded poor results in infants [11]. While a connection between the SVC and PA was tolerated well, direct connection of the IVC to the pulmonary artery resulted in large amount of pooling in lower parts of the body, in particular, in the splanchnic bed, and was found to be incompatible with long-term survival. In spite of the fact that, at the time, the complete cavopulmonary anastomosis was known as “a procedure for which a disease was yet to be found,” it nevertheless became the cornerstone of Fontan repair [11].
20.2 The Fontan Procedure

Anatomical features of “single ventricle” hearts. Tricuspid atresia (a) is marked by complete absence of tricuspid valve and a rudimentary right ventricle. Communication between the atria (ASD) enables the blood to reach the left ventricle, which subserves the pulmonary and systemic circulations. The salient features of the hypoplastic left heart syndrome (HLHS) include an aberrant left ventricle and hypoplastic aorta (b). The pulmonary and systemic circulations are placed in parallel and are supplied by the only functional (right) ventricle. A mixture of arterial and venous blood reaches the systemic circulation via the patent ductus arteriosus (“duct-dependent disease”). Arrows indicate the direction of blood flow. ASD atrial septal defect, VSD ventricular septal defect, PDA patent ductus arteriosus, RA right atrium, LA left atrium, RV right ventricle, LV left ventricle, PA pulmonary artery, PV pulmonary veins, LPA left pulmonary artery, IVC inferior vena cava, Ao aorta
It is evident from their report that the dilemma continued in the early 1970s when Fontan and Baudet initially attempted the right ventricular bypass in three patients with tricuspid atresia. “We were of the opinion,” they wrote, “that the right atrium of a normal heart could not provide the required work, whereas a hypertrophied atrium, as in tricuspid atresia could supply the additional work represented by pulmonary arterial pressure higher than the left atrial pressure” [14]. In other words, will the right atrium be able to supply enough pressure to overcome greatly increased pulmonary resistance and effectively “drive” the systemic venous return to the left atrium in spite of adverse pressure gradients?
It transpired that Fontan and Baudet had, in fact, misplaced their misgivings about the importance of the right atrium for the success of their novel procedure. Circulation model experiments by de Leval and coworkers in the 1980s showed that interposition of a compliant atrial chamber between the systemic venous and pulmonary compartments dissipates, rather than enhances the propulsive force (generated by the single, i.e., systemic ventricle) needed to overcome pulmonary resistance [15, 16]. This, together with frequent observations of chronic atrial distension and long-term arrhythmias, led to the conclusion that the hemodynamic importance of the right atrium (in atriopulmonary connection) has been overestimated, and by the mid-1990s the technique of total cavopulmonary connection was widely adopted in most patients with functionally univentricular hearts [17].
It is generally accepted that surgical correction of the HLHS will have a better outcome if the pulmonary vascular resistance is low and the left atrial pressure can be maintained at low levels [18]. The ultimate goal of staged repair of any HLHS defect is the separation of pulmonary and systemic circulations and bringing their flow ratios (Qp:Qs) as close to unity as possible. The repair typically proceeds in three stages. The initial aim is to achieve unrestricted flow from the heart, i.e., by reconstructing the stenotic part of the aorta (Norwood repair). Excessive blood flow to the lung, if present, is optimized by pulmonary artery banding. In case of a deficient flow, say, due to a stenotic pulmonary artery, a systemic to pulmonary artery shunt (Blalock–Taussig) is placed. As a rule, the heart is now subjected to chronic volume overload which, incidentally, improves the development of pulmonary vasculature.

Two-stage Fontan repair. Optimization of pulmonary and cardiac blood flows, i.e., the first stage repair (hemi-Fontan) is achieved by connecting the superior vena cava to the right pulmonary artery (Glenn shunt) (a). Chronic volume overload results in remodeling (strengthening) of the (single) ventricle. The final stage of Fontan repair consists of connecting the inferior vena cava to the pulmonary artery. The extrapericardial cavopulmonary connection by means of a vascular graft, schematically depicted in (b), is generally used in children older than 3 years. Fenestration (marked by arrowhead in b), a 3–6 mm communication between the extension of inferior vena cava (the baffle) and the atrium, is often added to vent excessive systemic venous pressures. Arrows indicate direction of blood flow. SVC superior vena cava, IVC inferior vena cava, RA right atrium, LA left atrium, RPA right pulmonary artery, Ao aorta, SV single ventricle
The final, crucial stage of repair consists of the complete separation of pulmonary and systemic circulations achieved by joining the inferior vena cava to the pulmonary artery. In addition, any existing anastomoses between the aorta and the pulmonary are ligated. A dramatic reversal of hemodynamics follows in which the previously chronically overloaded ventricle now operates at a fraction of its previous load (in the range of 25–75%) [18]. The combination of a hypertrophied ventricular wall and reduced filling can result in enhanced systolic thickening (contractility–afterload mismatch) with early-diastolic dysfunction, markedly reduced CO [21], and increased venous pressure immediately after completion of the Fontan circuit. During the following 2–3 weeks, the CO gradually increases and generally matches the increase in blood volume found to be in the range of 17% in an experimental model [22].
With an increasing pool of survivors [23], the understanding of the complex Fontan physiology is evolving (see [24] for recent review). The long-term outcome in single ventricle Fontan repair patients is characterized by decreased cardiac output (range of 60–70% of normal controls) and mildly to moderately reduced exercise capacity. It is not clear whether the exaggerated fall in BP during exercise is primarily due to reduced CO or due to decreased vasomotor tone [25]. It has been observed that during exercise Fontan patients employ a strategy of taking more frequent, shallow breaths to harness the beneficial effect of negative inspiratory phase and thus improving the pulmonary blood flow and, in turn, CO by up to 30% [26]. Furthermore, the minute ventilation at the same given intensity of exercise is greater in Fontan patients than in normal controls [27]. Studies of pulmonary blood flow patterns at rest reveal an exaggerated flow response during the respiratory cycle and point to the importance of the “respiratory pump” as an important auxiliary source of venous return. Doppler flow studies by Redington and coworkers demonstrated a cessation of blood flow in the PA and flow reversal in SVC during short periods of forced expiration (Valsalva maneuver) and a considerable augmentation of forward flow during forced inspiration (Mueller maneuver) [21, 28].
During early years after palliation there is partial regression of ventricular hypertrophy with persisting ventricular dysfunctions which, in the absence of residual rhythm and conduction disturbances, rarely presents significant cardiovascular limitation (New York Heart Association class I or II) [29, 30].
The systemic complications of Fontan circulation are mostly related to increased venous pressure and congestion, and include protein loosing enteropathy (3–15%), hepatomegaly with ascites and peripheral edema, interstitial pulmonary edema, pleural effusion, and predisposition to thromboembolic events (pulmonary embolism, stroke, myocardial infarction) [29, 31]. The dynamics of the lymphatic circulation is profoundly altered and an increased drainage via the lymphatic system is an important adaptation in Fontan patients. There is evidence that lymphatics are grossly abnormal in the thoracic cavity, the mesentery, and the gut, and resemble those of patients with chronic heart failure in whom thoracic duct pressures and flows can increase up to three times [32].
It is of interest that (as mentioned in Chap. 11) ontogenetically, the left ventricle is a relatively late evolutionary gain of adaptation from the gill-based water respiration to lung respiration. The amphibians already have two atria, but a single ventricle, which serves systemic and pulmonary circulations (cf. Fig. 11.7b). The parallel arrangement of systemic and pulmonary circuits and “pulmonary hypertension” is physiological in amphibians where, in addition, the excessive lymph production is cleared by accessory (lymph) hearts. It has been suggested that the HLHS can be viewed as a “developmental arrest” at the stage of amphibian circulation [33] with far-reaching consequences affecting not only the macrocirculation but also the microcirculation [34].
Chronic effects of total systemic to pulmonary drainage (in the absence of the right heart) on the pulmonary circulation are less well understood and appear to be related to steady, rather than pulsatile flow, to loss of normal ventricular-vascular coupling and to abnormal endothelial reactivity, possibly related to reduced endothelial NO production [21, 34]. Overt and silent pulmonary embolic episodes may contribute to increase in pulmonary vascular resistance and adversely affect long-term survival [35]. Notwithstanding the radical deviation from what is considered a “normal” circulatory anatomy, the vast majority (about 90%) of hospital survivors with Fontan circulation nevertheless enjoy remarkably normal lives [36].
20.3 The Fontan Paradox
As previously mentioned, the Fontan “correction” consists of placing the systemic and pulmonary circulations in series, as is normally the case. According to the conventional model, the pressure generated by the single ventricle is now considered the sole source of blood propulsion for systemic as well as the pulmonary circulations. In terms of the hydraulic (or Ohmic) analogue, the circuit resistances are now the sum rather than the reciprocal of their values. The question naturally arises whether the weakened ventricle, operating at reduced preload, can generate a high enough pressure to overcome the vastly increased peripheral resistance? The answer, of course, is affirmative, or these patients would never survive the radical anatomical re-arrangement of their circulations. Paradoxically, rather than presenting with signs of left ventricular failure with pulmonary congestion and increased LV filling pressures, the Fontan patients exhibit signs of right ventricular failure (hepatic congestion and peripheral edema) in the absence of the right ventricle! In other words, too much blood is being “pushed” (by the single ventricle!) into the systemic venous reservoir. Unlike in the bi-ventricular circulation, where CVP are normally low (0–5 mmHg), patients with Fontan circulation have significantly higher central pressures (in the range of 15–20 mmHg) and placing a “relief valve,” by creating a communication between the systemic and pulmonary circulations (fenestration) (see Fig. 20.2), is an effective way to improve survival and reduce congestion-related complications [37, 38]. In addition, placement of fenestration has been shown to improve ventricular preload and augment CO [39]. Evidently, the ensuing cyanosis due to R to L shunting is tolerated better than complications related to increased venous pressure.
No doubt, the Fontan circulation presents one of the biggest challenges to the conventional pressure-propulsion model of circulation and some interesting suggestions have been proposed to solve it, such as, for example, “The paradox of the Fontan circulation is that it imposes a caval hypertension and a pulmonary arterial hypotension. In terms of hemodynamics, a mechanical device capable of a step down in pressure energy of 5 mmHg in the inferior vena cava and producing a step up in pressure energy of 5 mm Hg in the pulmonary arteries would reverse the Fontan paradox” [17, 40].
While it remains doubtful whether “the paradox” will yield to a simple mechanical measure, several related phenomena have been described which, it is believed, form a rational basis for its understanding. They can be conveniently grouped into those related to the heart and to pulmonary circulation and have been summarized in a recent review by Gewillig and coworkers [39]. It has been frequently observed by clinicians, for example, that in the acute post-operative period, the “Fontan operation leaves the child’s cardiac output nearly totally dependent upon pulmonary blood flow” [41]. This view is also echoed by Veldtman and coworkers in a recent review on the subject [1]. In addition, in spite of reduced ventricular contractility Fontan patients fail to increase CO in response to inotropes, a situation observed in several other forms of acute or chronic heart failure [42]. Similarly, the indices of ventricular performance do not improve by decreasing systemic vascular resistance (by administration of vasodilators) and may even worsen exercise performance [43].
Apart from a few exceptions, pulmonary vascular resistance will control the cardiac output. The circuit runs on “autopilot”, with little interference by the clinician. This has frustrated many cardiologists as they have experienced that “their organ” no longer controls cardiac output. Cardiac output can be increased by improving flow to and into the lungs, or by bypassing the lung with a fenestration. The effect of pulmonary vasodilators is usually very modest [36].
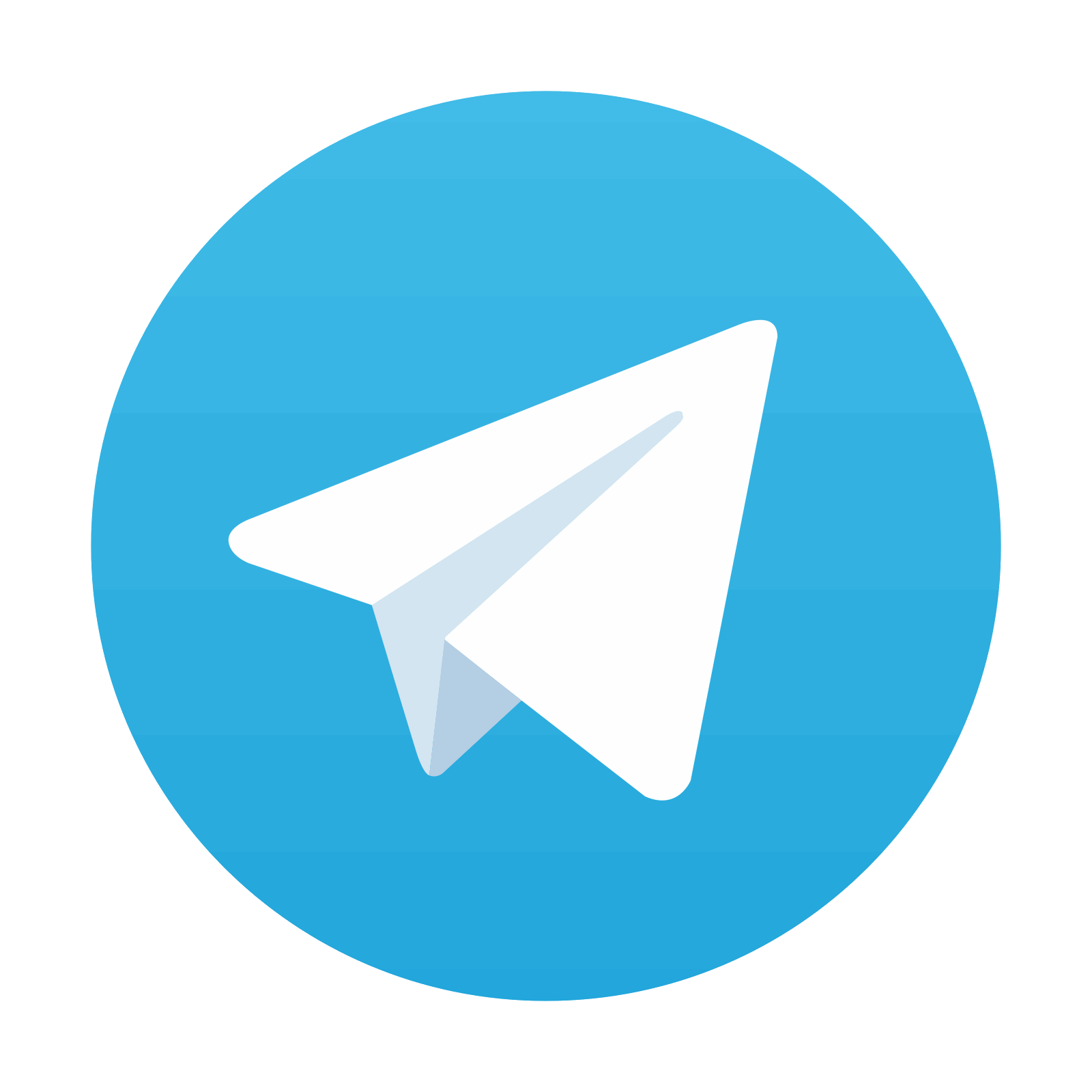
Stay updated, free articles. Join our Telegram channel

Full access? Get Clinical Tree
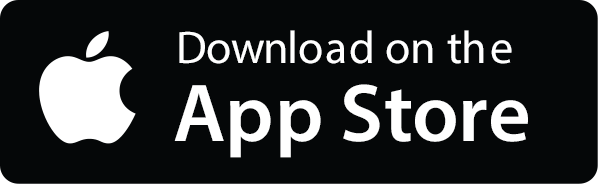
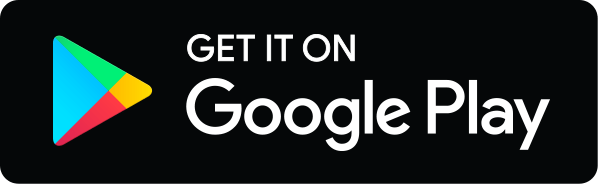