Background
Regional and global function can be measured by echocardiography using speckle-tracking, a technique that has previously been validated against crystal sonomicrometry. However, the application of Velocity Vector Imaging (VVI) to images obtained from cardiac magnetic resonance (CMR) imaging has never been validated against those values derived from VVI applied to two-dimensional echocardiographic images in the same patient group. The aim of this study was to validate for the first time the application of VVI to retrospectively acquired CMR data sets for the assessment of left ventricular strain and rotation, using echocardiographic strain assessment by VVI as the reference technique.
Methods
Cine steady-state free precession CMR data sets and two-dimensional echocardiographic images obtained on the same day in 36 adult patients with hypertrophic cardiomyopathy were analyzed retrospectively using VVI to quantify global longitudinal and circumferential strain and rotation parameters.
Results
The absolute differences in longitudinal strain between the two imaging modalities were −1.1 ± 3.3% (endocardial) and −2.2 ± 3.6% (full thickness). The absolute differences in circumferential strain were −4.7 ± 5.3% (endocardial) and −3.4 ± 3.8% (full thickness). CMR consistently resulted in higher strain values than echocardiography. The absolute differences in twist were −0.2 ± 5.6% (endocardial) and 0.1 ± 5.8% (full thickness).
Conclusions
The application of VVI to CMR data sets allows a feasible and reproducible method for strain analysis in HCM, demonstrating excellent agreement with two-dimensional echocardiography–derived values. Given the superior image quality obtained with CMR in a significant proportion of patients, this technique provides a method for strain assessment without the need for dedicated CMR acquisition and analytic techniques.
To date, numerous studies using both speckle-tracking echocardiography and myocardial tagging using cardiac magnetic resonance (CMR) imaging have demonstrated abnormalities in strain and rotation in patients with hypertrophic cardiomyopathy (HCM) compared with healthy controls. The assessment of myocardial mechanics not only describes function but also provides invaluable information regarding prognosis in a variety of disease processes, such as coronary artery disease and idiopathic dilated cardiomyopathy. Assessment of myocardial mechanics has been proven to be superior to conventional measures such as left ventricular (LV) ejection fraction in identifying abnormalities of cardiac function in both coronary artery disease and chemotherapy-induced toxicity. Both regional and global function can be measured from echocardiographic images using speckle-tracking echocardiography, and the Velocity Vector Imaging (VVI; Siemens Medical Systems, Mountain View, CA) software technique has previously been validated against crystal sonomicrometry. In addition, results derived from speckle-tracking methods have been shown to yield results comparable to those obtained from tissue phase mapping in cine phase-contrast velocity magnetic resonance imaging. In addition, other methods based on feature-tracking, particularly the use of TomTec software (TomTec Imaging Systems GmbH, Munich, Germany), have been applied to cine CMR images for the purpose of strain assessment in patients with ischemic cardiomyopathy and have been compared with harmonic phase imaging analysis in patients with Duchenne muscular dystrophy. Several speckle-tracking software systems are available for offline analysis, including EchoPAC (GE Healthcare, Milwaukee, WI), VVI, QLAB CMQ (Philips Medical Systems, Andover, MA) and TomTec, of which EchoPAC and VVI are the most common. At present, only VVI and TomTec feature-tracking applications have been used for the assessment of strain on CMR images, as both EchoPAC and QLAB CMQ are echocardiography-based software programs that are vendor specific. The assessment of strain parameters from echocardiographic images may be limited by image quality and suboptimal acoustic windows in a proportion of patients. CMR imaging more consistently provides high-quality imaging with not only complete anatomic coverage of the entire left ventricle but also superior endocardial and epicardial delineation. Although strain assessment on CMR-derived images can be performed using myocardial tagging methods such as harmonic phase, phase-contrast velocity imaging, displacement encoding, and strain encoding, these techniques necessitate prospective image acquisition and require special expertise and software for analysis. In contrast, VVI provides a potential method for analysis of routinely acquired CMR data sets from any vendor and retrospective analysis.
VVI has previously been applied to CMR images for the assessment of ventricular function in patients with congenital heart disease and has been compared against complementary spatial modulation of magnetization analysis in patients with HCM and normal controls. However, the application of VVI to images obtained from CMR has never been validated against those values derived from two-dimensional (2D) echocardiographic images in the same patient group.
The aim of this study was to validate VVI measurements derived from CMR against those derived from echocardiographic data sets performed in a population of patients with HCM who underwent both studies on the same day. Given that VVI provides a method that can be applied retrospectively to CMR images that were not prospectively tagged during image acquisition and can be used to assess myocardial mechanics from both echocardiographically and routinely acquired CMR images, it may prove most valuable in those patients with suboptimal echocardiographic windows.
Methods
Study Population
A retrospective cohort of 41 adult patients with HCM (defined as a maximal septal thickness ≥13 mm and a ratio of septal to posterior wall thickness ≥1.3, in the absence of another cardiac or systemic disease that could cause LV hypertrophy) was included in the study. All patients had undergone comprehensive transthoracic echocardiography and CMR <24 hours apart. In a subset of five patients, either complete longitudinal or circumferential strain was not available, because of incomplete data sets or suboptimal echocardiographic image quality, resulting in a final patient population of 36 patients for longitudinal and circumferential strain, respectively, and 34 patients for assessment of rotation parameters.
Clinical and 2D Echocardiographic Data
A retrospective chart review was performed to obtain demographic data for the patient cohort. Standard 2D echocardiographic data were obtained from the study performed on the same day as CMR. All studies were performed on a Toshiba Artida 4D System (Toshiba America Medical Systems, Tustin, CA) with a PST-30SBT transducer, according to the standards of the American Society of Echocardiography. Two-dimensional data sets for speckle-tracking analysis included three short-axis views (basal, midventricular, and apical) and three apical longitudinal views (four, two, and three chamber). Special care was taken to ensure the acquisition of optimal circumferential cross-sectional slabs at the basal (mitral leaflets visible without presence of papillary muscles), midventricular (papillary muscles visible without presence of mitral leaflets), and apical (toward the LV apex, absence of papillary muscles) levels. Adjustments in sector depth and angle yielded a temporal resolution of 80 to 100 frames/sec. A minimum of 40 frames/cycle was required for offline analysis (with a range of 40–82 frames/cycle achieved). LV outflow tract obstruction was defined by continuous-wave Doppler echocardiography as a peak instantaneous systolic gradient ≥30 mm Hg under resting conditions due to marked systolic anterior movement of the mitral valve leaflets. The study was approved by the Investigational Review Board at Tufts Medical Center and the Research Ethics Board of the Toronto General Hospital.
CMR Protocol
At Tufts Medical Center, CMR imaging was performed using a Philips Gyroscan ACS-NT 1.5 scanner (Philips Medical Systems, Best, The Netherlands). Cine steady-state free precession (SSFP) images were acquired in short axis (sequential 10-mm slices from the atrioventricular ring to the apex) and two-chamber, three-chamber, and four-chamber long axes. Scan parameters were as follows (1) retrospective electrocardiographic triggering; (2) spatial resolution, 1.6 × 1.6 mm/pixel; (3) field of view, 32 to 36 cm; and (4) temporal resolution, 35 msec. LV volumes, mass, and ejection fraction were measured using standard volumetric techniques and analyzed using commercially available software (MASS version 6.1.6; MEDIS Medical Imaging, Inc., Leiden, The Netherlands). Maximal end-diastolic LV wall thickness measurements for each of the 16 segments were provided automatically by the commercially available software. LV mass data were indexed to body surface area.
VVI
VVI is an angle-independent feature-tracking method that incorporates speckle-tracking and endocardial and epicardial contour-tracking. VVI quantifies myocardial motion from B-mode clips by automatically tracking user-defined endocardial and epicardial contours to define the inward and outward myocardial motion. On the basis of the motion of the tracked points between the frames and the knowledge of the time interval between frames, 2D tissue velocity is computed. Strain and strain rate are computed by the change in the relative distance between localized tracked trace points, combined with the difference in the relative displacement of the tissue motion behind the tracked points. Strain was defined as the instantaneous local trace lengthening or shortening and strain rate as the rate of lengthening or shortening. In the long-axis images, the software calculates longitudinal (systolic shortening) and radial (systolic thickening) velocity, strain, strain rate. In the short-axis images, it calculates circumferential and radial strain and strain rate, as well as radial and rotational velocities and angles relative to the ventricular centroid (center of the LV cavity).
The speckle-tracking program, VVI version 3.0.0, was applied to the echocardiographic and CMR cine SSFP images from archived studies, allowing endocardial, myocardial, and epicardial strain parameter assessment.
Cine SSFP data derived from CMR images were converted from Digital Imaging and Communications in Medicine to audio-video interleaved format, creating 30 cardiac phases. Archived echocardiographic images in Digital Imaging and Communications in Medicine format were used for analysis. Subsequently, myocardial motion was quantified by automatic tracking of user-defined points in both subendocardial and subepicardial regions, as previously described, to derive both endocardial and full-thickness strain. Default software settings were used with tracking of both endocardial and epicardial borders for analysis of both echocardiographic and CMR-derived images.
Myocardial Mechanics
Longitudinal strain was measured from the horizontal long-axis/four-chamber, vertical long-axis/two-chamber, and three-chamber/LV outflow tract views on CMR and from apical four-chamber, apical two-chamber, and apical three-chamber views on echocardiography. Circumferential strain was measured in three short-axis views. The basal level was selected as a short-axis view of the mitral valve (LV outflow tract no longer visible, papillary muscles not visible), the midventricular level at the level of the papillary muscles (mitral leaflets not visible), and the apical level at the true LV apex (papillary muscles not visible). In addition, radial strain was assessed from the short-axis views (global radial strain). Global longitudinal strain and strain rate values were obtained by averaging the segmental strain values (six segments in each of the four-chamber, two-chamber, and three-chamber views, for a total of 18 segments). Global circumferential strain and strain rate values were obtained by averaging the segmental strain values (six segments in the basal and midventricular short-axis views and four segments in the apical short-axis view, for a total of 16 segments). For each view, endocardial borders were manually traced in the end-diastolic frame, with the system automatically identifying the epicardial border. Manual adjustment of each border independently of the other could be performed before analysis and processing. The version of VVI used in the present study measures strain in three layers, with the myocardial layer automatically assigned midway between the endocardial and epicardial layers by the software program. Strain parameters were recorded after visual confirmation of best endocardial and epicardial motion tracking (by operator subjective visual assessment). Segments with poor tracking were excluded from further analysis. If more than two segments demonstrated poor tracking, the view was excluded from further analysis.
Averaged myocardial rotation angles (measured from the short-axis views) were used to calculate twist. Twist was defined as the maximal instantaneous basal-to-apical angle difference. To standardize the temporal evaluation of twist, all twist and untwist times were normalized and presented as a percentage of the R-R cycle length.
All echocardiographic images were analyzed in a random order by a single reader. CMR images were analyzed in a random order by a second independent reader who was blinded to the results of analysis of the echocardiographic images.
Intraobserver and Interobserver Variability
For intraobserver variability, 10 randomly assigned patients were reanalyzed by the same observer on both echocardiographic and CMR data sets. For interobserver variability, the same patients were analyzed by a second observer. We assessed the variability of global longitudinal and global circumferential strain, as well as total twist.
Statistical Analyses
Continuous data are reported as mean ± SD. Comparison of the values obtained from the two imaging modalities was made by means of Pearson’s correlation and Bland-Altman analysis. Intraobserver and interobserver variability was assessed using Bland-Altman analysis. All statistical analyses were performed using MedCalc version 11.6.0.0 (MedCalc Software, Mariakerke, Belgium). Statistical significance was defined as a P value < .05.
Results
Patient Demographics and Clinical Data
Clinical and demographic findings as well as basic echocardiographic and CMR characteristics are shown in Table 1 . All patients had LV ejection fractions ≥55%, with 46% of the cohort having evidence of either resting or provocable LV outflow tract gradients of ≥30 mm Hg.
Variable | Value |
---|---|
Age (y) | 42.8 ± 18 |
Men | 28 (68%) |
NYHA class | 1.5 ± 0.7 |
LVEF (%) | 67.4 ± 7 |
LVEDVI (mL/m 2 ) | 83.7 ± 17 |
LVESVI (mL/m 2 ) | 27.6 ± 10 |
LVMI (g/m 2 ) | 84.7 ± 29 |
Maximal wall thickness (mm) | 20.5 ± 4 |
LA size (mm) | 39.8 ± 8 |
Obstructive HCM | 19 (46%) |
Myocardial Mechanics
2D Strain Imaging
Acquired echocardiographic clips had 40 to 82 frames/cycle (median, 57 frames/cycle) and a temporal resolution ranging from 80 to 100 frames/sec. Cine SSFP CMR images were acquired at a fixed rate of 30 frames/cycle. Of the 1,296 potential segments available for longitudinal strain assessment, 1,188 (92%) were analyzable. Of the 1,152 potential segments available for circumferential and radial strain assessment, 1,062 (92%) were analyzable.
Longitudinal Strain
Representative longitudinal strain curves obtained from four-chamber, two-chamber, and three-chamber views are shown in Figure 1 . The values for global longitudinal strain derived from CMR and echocardiography and their correlations are shown in Table 2 . Overall the mean differences in global longitudinal systolic strain for the two imaging modalities (CMR − echocardiography) were −1.1 ± 3.3% for endocardial strain and −2.2 ± 3.6% for full-thickness strain ( Figure 2 ), with CMR consistently resulting in higher strain values than echocardiography.
Echocardiography | CMR | |||||
---|---|---|---|---|---|---|
Parameter | ( n = 36) | ( n = 36) | Correlation | P | Bias ± LOA (Bland-Altman) | Percentage difference |
Endocardial GLS | −16.3 ± 4 | −17.4 ± 3 | 0.87 | <.0001 | −1.1 ± 3.3 | 6.8 |
Myocardial GLS | −11.9 ± 3 | −14.4 ± 3 | 0.81 | <.0001 | −2.5 ± 3.4 | 19.3 |
Epicardial GLS | −8.1 ± 3 | −10.9 ± 4 | 0.52 | .001 | −2.8 ± 6.0 | 29.1 |
Full-thickness GLS | −12.1 ± 3 | −14.2 ± 3 | 0.81 | <.0001 | −2.2 ± 3.6 | 16.2 |
Circumferential Strain
Representative circumferential strain curves obtained from short-axis views at the level of the base, midventricle, and apex are shown in Figure 3 . The values for global circumferential strain derived from CMR and echocardiography and their correlations are shown in Table 3 . Overall, the mean differences in global circumferential systolic strain for the two imaging modalities (CMR − echocardiography) were −4.7 ± 5.3% for endocardial strain and −3.4 ± 3.8% for full-thickness strain ( Figure 4 ), with CMR consistently resulting in higher strain values than echocardiography.
Echocardiography | CMR | |||||
---|---|---|---|---|---|---|
Parameter | ( n = 36) | ( n = 36) | Correlation | P | Bias ± LOA (Bland-Altman) | Percentage difference |
Endocardial GCS | −29.4 ± 4 | −34.1 ± 4 | 0.80 | <.0001 | −4.7 ± 5.3 | 15.3 |
Myocardial GCS | −16.3 ± 3 | −20.4 ± 3 | 0.68 | <.0001 | −4.1 ± 4.3 | 22.7 |
Epicardial GCS | −10.1 ± 2 | −11.3 ± 2 | 0.42 | .01 | −1.3 ± 4.6 | 11.7 |
Full-thickness GCS | −18.6 ± 3 | −21.9 ± 3 | 0.76 | <.0001 | −3.4 ± 3.8 | 16.7 |
Global radial strain | 33.2 ± 10 | 64.2 ± 24 | 0.52 | .001 | 30.9 ± 40.1 | 60.3 |
Basal endocardial CS | −24.9 ± 4 | −29.6 ± 5 | 0.73 | <.0001 | −4.7 ± 7.1 | 17.1 |
Mid endocardial CS | −29.3 ± 5 | −32.5 ± 6 | 0.73 | <.0001 | −3.2 ± 7.9 | 10.3 |
Apical endocardial CS | −31.5 ± 7 | −40.3 ± 6 | 0.39 | .03 | −8.9 ± 14.8 | 12.9 |
Circumferential strain data by short-axis levels were also analyzed ( Table 3 ). The mean difference in global circumferential systolic strain at the basal level for the two imaging modalities (CMR − echocardiography) was −4.7 ± 7.1%, with a correlation of 0.73. At the level of the midventricle, the mean difference was −3.2 ± 7.9%, with a correlation of 0.73. There was a poor correlation (0.39) at the apical level, with a mean difference of −8.9 ± 14.8%.
Global radial strain values derived from CMR and echocardiography and their correlations are shown in Table 3 . CMR consistently resulted in higher radial strain values than echocardiography.
Rotation Parameters
The values for rotation at the three short-axis planes, total twist, as well as twist and untwist times derived from CMR and echocardiography and their correlations are shown in Table 4 .
Echocardiography | CMR | |||||
---|---|---|---|---|---|---|
Parameter | ( n = 34) | ( n = 34) | Correlation | P | Bias ± LOA (Bland-Altman) | Percentage difference |
Basal endocardial rotation (°) | −7.7 ± 2 | −7.1 ± 3 | 0.63 | .0001 | 0.6 ± 4.2 | 23.5 |
Basal full-thickness rotation (°) | −5.9 ± 2 | −6.0 ± 2 | 0.41 | .01 | −0.1 ± 4.1 | 28.7 |
Mid endocardial rotation (°) | 0.03 ± 6 | −0.6 ± 5 | 0.24 | NS | −0.6 ± 12.8 | — |
Mid full-thickness rotation (°) | 0.3 ± 4 | −0.9 ± 3 | 0.29 | NS | −1.1 ± 8.5 | — |
Apical endocardial rotation (°) | 8.6 ± 4 | 8.5 ± 4 | 0.70 | <.0001 | −0.1 ± 5.9 | 27.4 |
Apical full-thickness rotation (°) | 6.6 ± 3 | 6.5 ± 3 | 0.71 | <.0001 | −0.1 ± 4.2 | 27.5 |
Endocardial twist (°) | 14.7 ± 4 | 14.5 ± 4 | 0.73 | <.0001 | −0.2 ± 5.6 | 15.9 |
Full-thickness twist (°) | 11.4 ± 3 | 11.6 ± 3 | 0.56 | .001 | 0.1 ± 5.8 | 21.1 |
TTPT (%CL) | 37.6 ± 8 | 38.7 ± 9 | 0.67 | <.0001 | 1.0 ± 13.4 | 15.2 |
TRRT (%CL) | 62.3 ± 8 | 61.3 ± 9 | 0.67 | <.0001 | −1.0 ± 13.5 | 9.7 |
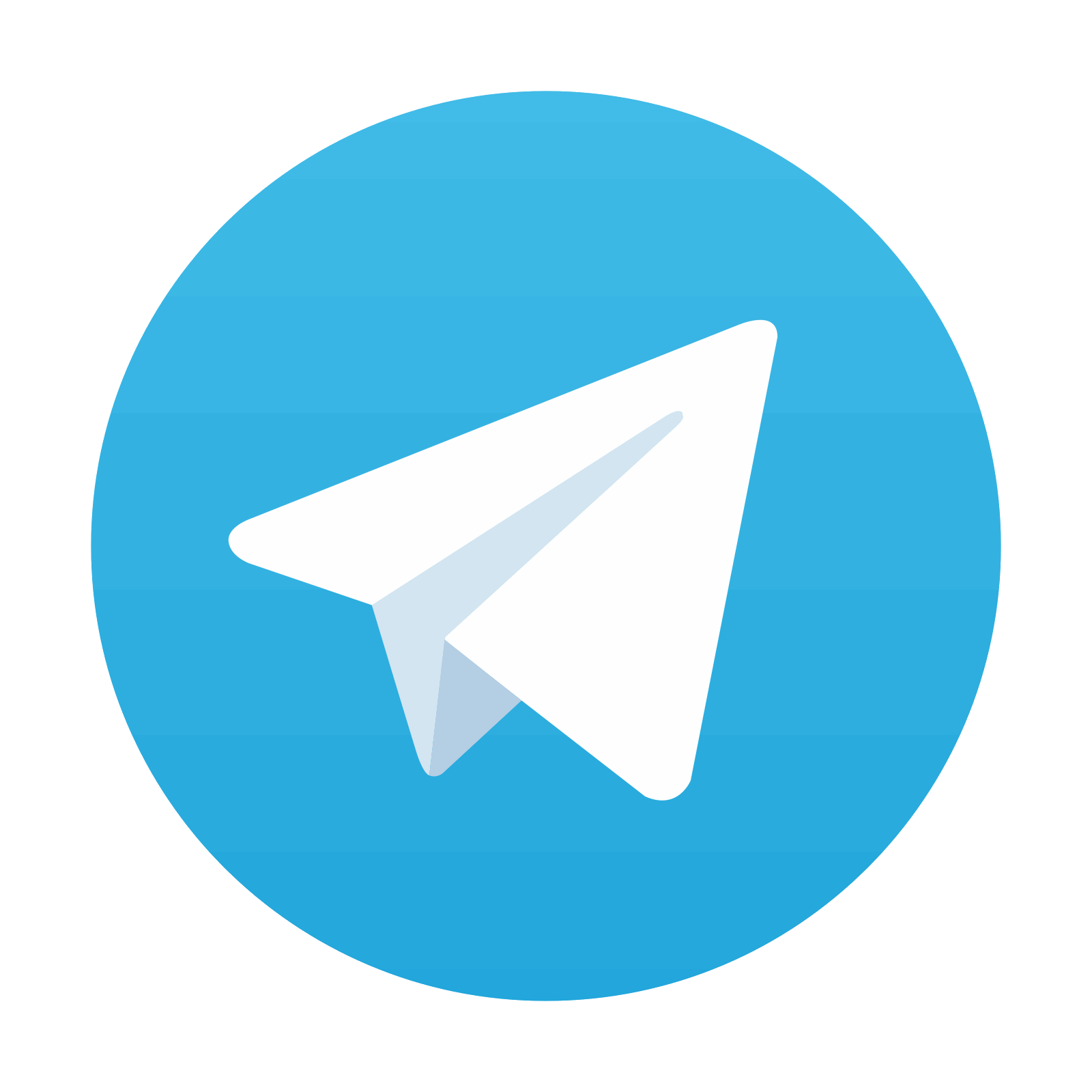
Stay updated, free articles. Join our Telegram channel

Full access? Get Clinical Tree
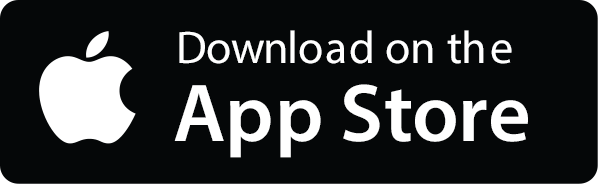
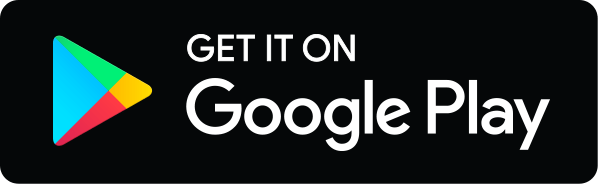
