Chapter 58
Varicose Veins
Endovenous Ablation and Sclerotherapy
Lowell S. Kabnick, Mikel Sadek
The standard treatment of varicose veins for many years has been surgical ligation and stripping of the affected vein. Although outcomes have improved in recent years because of enhanced understanding of lower extremity venous anatomy, the failure rate with this approach is frequently reported to be between 20% and 30%.1,2 In addition, surgical ligation and stripping are invasive, usually requiring general anesthesia and several weeks’ recovery before return to normal activities. In response to the need for a less invasive approach, numerous endovenous treatments have emerged over the past several years. The most notable endovenous advancements are the now widespread techniques of radiofrequency ablation (RFA) and endovenous laser ablation (ELA). These methods have demonstrated clinical superiority to stripping and surgical ligation as well as significantly less postoperative pain and recovery time.3,4
When RFA was initially approved for use in the United States in 1999, the procedure was relatively lengthy and took place primarily in a formal operating room with use of conscious sedation.5
Catheter withdrawal speed during the procedure was difficult to judge because there were no markings or measurements on the catheter. The pullback segment of the procedure was also tedious inasmuch as the user was required to withdraw the catheter at a rate of 1 to 2 cm/min, for an overall treatment time of 15 to 20 minutes. Tumescent anesthesia was delivered blindly or under ultrasound guidance by hand injection. This novel technology has undergone several enhancements since its inception, and the latest iteration utilizes segmental ablation, which is discussed later in this chapter.
Boné6 first reported the use of endoluminal laser energy in 1999. Shortly thereafter, Navarro and colleagues7 published the first report of a minimally invasive method for treating an incompetent great saphenous vein (GSV). In 2002, ELA was approved for use in the United States for ablation of the GSV. Several improvements in this procedure and technology have been made since 2002 and are discussed further along with the latest techniques and devices.
Decades before the advent of the new endothermal ablation procedures, sclerotherapy was used as a means of treating the highest points of venous reflux, including the GSV and small saphenous vein (SSV). However, the mechanism of sclerotherapy is such that the sclerosing agent must make contact with the vein wall to cause endothelial damage. Consequently, treatment of larger veins with sclerotherapy historically has shown a lack of consistent success. New methods and procedures have greatly enhanced the outcomes of sclerotherapy, however. This chapter reviews these strategies as well as the most advanced methodology and current trends with new sclerosing agents.
Finally, cutaneous lasers and intense pulse light (IPL) devices are gaining popularity, largely because of convenience and ease of use. These technologies work by using a range of wavelengths to target different depths and diameters of vessels. Acting as a chromophore, hemoglobin within red blood cells of the target vein absorbs and converts the light to thermal energy, which causes vessel destruction.8 These devices are able to penetrate the skin to treat the vessel of choice without damaging the overlying skin or surrounding tissues.8 This theory of selective damage to target tissue, known as selective photothermolysis,8 was first conceptualized in the 1980s. Numerous devices are available, each of which is analyzed in this chapter.
Relevant Anatomy
The venous circulation in the lower extremities is a complex system composed of several levels of veins that function to return deoxygenated blood to the heart and lungs. Deep veins located within the deep leg compartment below the muscle fascia account for the majority of blood return, facilitated by valves within the veins and muscle pumps along the extremities. The superficial veins are predominantly suprafascial and function as the principal collecting veins for the lower extremity. In the absence of fascial support, the thin-walled superficial veins are highly expansible and are able to dilate significantly to accommodate large volumes of blood thereby acting as temporary storage.9
Great Saphenous, Small Saphenous, and Perforating Veins
For the relevant anatomy of the great saphenous vein (GSV), small saphenous vein (SSV), and perforating veins, please refer to Chapter 57.
Reticular Veins
Veins at the next level are known as reticular veins. These thin-walled blue venules lie within the superficial compartment10 and have diameters between 1 and 3 mm.11 Reticular veins may connect to the saphenous veins and are largely a network of vessels that form a system termed the lateral subdermic venous system (LSVS).12 The LSVS is located along the lateral aspect of the leg and extends above and below the popliteal area.13 Ultrasound studies have revealed that the reticular veins of the LSVS may connect to telangiectasias in up to 88% of patients.14 Reticular veins may become incompetent under high pressure and in turn can affect the connected telangiectasias.
Telangiectasias
Telangiectasias differ from reticular veins in both size and appearance; telangiectasias are defined as dilated venules, capillaries, or arterioles and measure 0.1 to 1.0 mm in diameter.15,16 The color of a telangiectasias depends on the origin of the vessel. Telangiectasias that stem from the arterial side of the capillary loop are flat and red, whereas those that arise from the venous side are raised and blue.16 These veins often appear in the thigh area near the LSVS. The reticular veins of the system are frequently “feeder” veins to telangiectasias.
Diagnostic Evaluation
Duplex ultrasonography of the extremities is one of the most critical studies for evaluating and diagnosing venous insufficiency and thrombosis; currently, this imaging method is the “gold standard” for superficial venous imaging 13 Proper evaluation includes assessment of both reflux and obstruction in the deep, superficial, tributary, and perforating veins.17 The primary goals of lower extremity ultrasound evaluation include precise mapping of abnormal pathways, identification of sources of reflux, accurate documentation of all target vessels to be treated, and preservation of normal veins.18
In diagnosing venous insufficiency, many clinicians have adapted a standardized system for evaluation, the CEAP (Clinical, Etiologic, Anatomic, Pathophysiologic) classification.19 This classification system was originally created in 1994 and contains two parts: a classification of chronic venous disease and a system to score the severity of such disease.19 The severity scoring system is based on disability, grading of symptoms and signs, and the affected anatomy.19
Most clinicians use the clinical scale, which consists of six levels of classification: complete absence of venous disease (C0), telangiectasias or reticular veins (C1), varicose veins more than 3 mm in diameter (C2), edema (C3), pigmentation or eczema (C4a), atrophie blanche or lipodermatosclerosis (C4b), healed venous ulcer (C5), and the most severe, active venous ulcer (C6) (Fig. 58-1A-H).19 Photographs of the target veins should be taken to document the clinical score and to compare with postoperative results.
Figure 58-1 CEAP (Clinical, Etiologic, Anatomic, Pathophysiologic) classification of venous insufficiency. A, C0: normal, asymptomatic leg. B, C1: telangiectasias or reticular veins. C, C2: varicose veins greater than 3 mm in diameter. D, C3: edema. E, C4a: pigmentation or eczema. F, C4b: lipodermatosclerosis. G, C5: healed venous ulcer; H, C6, active venous ulcer.
Treatment Selection
Accepted endovenous treatment modalities include endovenous thermal ablation, sclerotherapy, cutaneous lasers, and IPL devices. Although each treatment modality has its specific advantages and indications, there is significant overlap. When considering a treatment algorithm, the clinician must be selective and base decisions on the needs of the individual patient. The primary goal in treating venous insufficiency is to first treat the highest point of reflux. Additional goals include eradication of all diseased varicosities, minimization of complications, relief of symptoms, and attainment of satisfactory cosmetic results.20 In the selection of a treatment for a given venous problem, its risk-to-benefit ratio should weigh greatly in decision making.
The highest point of reflux in the vast majority of patients is in either the GSV or the SSV, and these veins are hence the focus of many endovenous treatments. Wong et al,21 studying 239 legs with primary reflux and 225 legs with recurrent reflux found saphenofemoral junction (SFJ) incompetence in 53% and 69% of the legs, respectively. In the same study, 21% of the primary reflux limbs and 25% of the recurrent reflux limbs demonstrated incompetence at the saphenopopliteal junction (SPJ).21 Similarly, another study found that 85% of limbs showed reflux within the GSV (68% at the junction), and 20% had reflux in the SSV.22 Patients with reflux in either of these primary superficial veins are candidates for an endothermal ablation procedure or ultrasound-guided endochemical venous ablation (sclerotherapy).
A much smaller percentage of limbs may have atypical varicosities, such as perforators or tributaries that demonstrate isolated reflux independent of the saphenous veins, commonly observed in junctional tributaries. The anatomy of these varicosities can fluctuate significantly in each patient; therefore, a treatment plan must be devised for each case. Patients with saphenous reflux may also exhibit concomitant atypical varicosities or telangiectasias. Currently, there is considerable controversy over whether adjunctive procedures to treat these varicosities and telangiectasias should be performed at the same time that truncal reflux is being eliminated. One study in which patients were simultaneously treated with ELA and ambulatory phlebectomy found that 94% of patients did not need to return for additional ambulatory phlebectomy procedures.23 Conversely, in a study of 181 patients who underwent RFA of the GSV, only 25% were able to avoid additional treatment with ambulatory phlebectomy.24 Because of the paucity of data on this topic, proceduralists must be selective about when to perform an adjuvant treatment such as phlebectomy or sclerotherapy.
Guidelines for choosing an appropriate endovenous option are listed in Table 58-1 and discussed in detail in the following sections.
The Society for Vascular Surgery (SVS) and the American Venous Forum (AVF) have compiled evidence-based recommendations for the care of patients with chronic venous disease.25 With regard to endovenous thermal ablation and sclerotherapy, the guidelines encompass recommendations 11.1 through 12.2. Per recommendations 11.1 and 11.2, both ELA and RFA are considered safe and efficacious and are recommended equally for the treatment of saphenous reflux. In addition, both treatments are recommended preferentially to open surgery because of reduced convalescence time and a decreased incidence of postprocedural pain and morbidity. Per recommendation 12.1, liquid or foam sclerotherapy is recommended for the treatment of telangiectasias, reticular veins, and varicose veins. Lastly, recommendation 12.2 recommends the use of endovenous thermal ablation over the use of foam sclerotherapy for the treatment of truncal reflux.
Radiofrequency Ablation
RFA is a minimally invasive technology that provides efficacious treatment of venous reflux with minimal discomfort and “downtime” for patients. One of the primary advantages of RFA is that the current procedure can be performed in an outpatient office setting with use of local tumescent anesthesia. As discussed earlier, the latest RFA technique includes several improvements over the original technology and features a “segmental ablation” method using the Covidien (formerly VNUS) ClosureFAST catheter26 (Covidien, Mansfield, Mass) that is designed for treating both the GSV and SSV (Fig. 58-2). The ClosureFAST catheter is constructed with a 7-cm bipolar electrode affixed to its distal end. The mechanism is such that the electrode must make direct contact with the vein wall to deliver radiofrequency energy. Contact with the wall results in destruction of the endothelium, occlusion by contraction of vein wall collagen, and thrombus formation.13 Eventually, fibrosis occurs within the vein as well as the formation of new collagen matrix, which further constricts the vein lumen and successfully occludes the vein.13
Figure 58-2 A, VNUS RFG Plus generator (VNUS Technologies, Inc., San Jose, Calif). B, AngioDynamics 980 Plus laser (AngioDynamics, Latham, NY). C, Vascular Solutions VariLase laser (Vascular Solutions, Inc., Minneapolis, Minn). D, Dornier Medilas D laser (Dornier MedTech, Munich). E, Sciton Pro-V laser (Sciton, Inc., Palo Alto, Calif). F, CoolTouch CTEV laser (CoolTouch, Inc., Rosevill, Calif). G, Biolitec ELVeS PL laser (biolitec AG, Jena, Germany).
RFA is indicated for superficial vein reflux of the lower extremity; contraindications include superficial venous thrombosis, deep venous thrombosis (DVT), aneurysm, and an ankle-brachial index of less than 0.9. Though having a pacemaker is not listed as a contraindication, there is concern regarding the use of RFA in the patient with a pacemaker. The patient’s cardiologist should be consulted before the procedure.
Preoperative Planning
On the day of the RFA procedure, the patient should be well hydrated to achieve maximum distention of the leg veins. In preparation for the procedure, duplex ultrasonography is performed to mark the skin overlying the target treatment vein.27 A surgical marking pen is used to avoid accidental tattooing of the skin. With use of duplex ultrasonography, the highest point of reflux is marked; it serves as a guide during the RFA procedure. It is advisable to heat the ultrasound gel before placing it on the patient’s leg because cold gel could cause venospasm. Similarly, the patient should be kept warm and comfortable in the procedure room to further avoid venospasm. Mild sedation such as alprazolam (Xanax), diazepam (Valium), or lorazepam (Ativan) may be prescribed for patients who exhibit anxiety.
Radiofrequency Ablation Procedure
An access site that will allow successful treatment of all possible diseased segments of the target vein is identified ultrasonographically. For ablation of the GSV, access is routinely initiated at or just below the popliteal area. With the patient in the reverse Trendelenburg position, lidocaine is administered at the selected site, and a percutaneous technique with a 21-gauge needle is used to gain access under ultrasound guidance. A small cutdown may be used but is not the preferred technique because of cosmetic concerns. A 0.018-inch guide wire is inserted into the GSV, and the needle is removed.
Next, a 7F × 7-cm or 11-cm sheath is advanced over the wire, the 0.018-inch wire is removed, and the 7F ClosureFAST catheter is inserted. The catheter is advanced to the predetermined point at which treatment will begin. If the catheter does not advance easily, a 0.025-inch guide wire may be back-loaded into the device to assist in passage. When the GSV is being treated, optimal positioning of the catheter tip is 2 cm peripheral to the SFJ.28 After correct placement of the catheter tip is confirmed by ultrasound, perivenous tumescent anesthesia is administered under ultrasound guidance along the entire target treatment length to create a fluid layer around the GSV. Proper administration of tumescent anesthesia is a critical component of the procedure, because it not only serves as an anesthetic but also compresses the vein around the catheter and protects the surrounding tissue from heat damage.28,29 Sufficient tumescent anesthesia should be instilled to create a 10-mm diameter around the vein, hence forming a distance of 10 mm between the targeted vein and the skin (Fig. 58-3). The amount of anesthesia required depends on the length of the vessel to be treated. A representative mixture includes 50 mL of 1% lidocaine with epinephrine in 450 mL of normal saline, neutralized with 5 to 10 mL of 8.4% sodium bicarbonate.27 Caution should be exercised to observe the safe limits for tumescent lidocaine administration, described by Klein30 to be 35 mg per kg body weight.
Figure 58-3 A, Delivery of tumescent anesthesia with the hand injection method. B, Delivery of tumescent anesthesia with a refillable syringe. C, HK Klein tumescent pump (HK Surgical Inc, San Clemente, Calif).
The type of needle used is based on user preference; popular choices include a 25-gauge hypodermic needle and a 22-gauge spinal needle. The needle is connected to a 20-mL syringe, 20-mL refillable syringe, or specialized tubing that is used in conjunction with an infusion pump. Many physicians prefer to use the pump delivery system because it decreases the time needed for administration of local anesthetic as well as the number of needle punctures. However, during the learning curve for the procedure, manual delivery of tumescent anesthesia enables the proceduralist to more effectively develop the skill set used for ultrasound guidance. Ideal needle positioning for delivery of the tumescent anesthetic is within the saphenous compartment to achieve maximum compression of the vein. After administration of the tumescent anesthetic, the patient should be placed in the Trendelenburg position to assist in vein collapse, apposition, and exsanguination.29 The sheath is withdrawn next, while catheter position is maintained, until the sheath is aligned with the index line on the catheter.29 Positioning of the catheter tip should be reconfirmed with ultrasound before treatment is commenced.
In addition to tumescent anesthesia, a technique that is crucial for success of the procedure is apposite compression.31 In the instructions for use of the Covidien ClosureFAST system, it is recommended that compression be applied with the ultrasound transducer positioned longitudinally over the heating element, as well as with two fingers distal to the transducer.29 When the proceduralist is prepared to begin, the generator is turned on and the unit is ready to use. With the segmental ablation technique of the ClosureFAST system, each 7-cm segment is treated independently for a 20-second treatment interval. Radiofrequency energy is delivered by a press of the button located on the handle of the catheter; once a 20-second interval is complete, delivery of energy automatically stops. The initial treatment segment 2 cm inferior to the SFJ requires two 20-second cycles to ensure successful ablation. Additionally, aneurysmal or large-diameter segments may be treated twice at the user’s discretion.28 For each 20-second cycle, the temperature must reach 120° C within 5 seconds after the initiation of delivery of the energy; if this temperature is not achieved within the stated time frame, the segment must undergo another 20-second treatment cycle.29 The generator monitors all parameters during the treatment cycle and alerts the user if the parameters are not successfully met. A segment should not undergo more than three treatment cycles.29
On completion of each 7-cm segment, the catheter is repositioned to the contiguous segment through the use of the 6.5-cm stepped markers on the catheter shaft. The 6.5-cm spacing allows 0.5-cm overlap of each pair of segments to ensure that no untreated areas are left between them. This process is repeated until the desired vessel length is treated, and the procedure can last from 1 to 5 minutes, depending on the length of the vessel and the number of repeated segments.28,32 When positioning the catheter for the final segment, the user should avoid pulling the heating element into the sheath because it may melt the sheath. After treatment, duplex ultrasonography is used to assess the treated vein for absence of reflux and evidence of occlusion.
Discharge and Follow-up
Postoperative compression with a 30– to 40–mm Hg graduated stocking is recommended for a period of at least 1 week.29,32,33 Patients are instructed to ambulate after the procedure before leaving the facility to assist in occlusion of the vessel.33 A duplex ultrasound follow-up examination is advised at 72 hours after the procedure to inspect for extension of thrombus into the deep system and to assess the treated veins.33 Further follow-up evaluations are performed according to physician preference; experts advocate examinations at 3 months, 6 months, and 1 year after ablation.28,34
Complications
Potential complications of RFA include vessel perforation, thrombosis, pulmonary embolism (PE), phlebitis, infection, nerve injury, and skin burns or discoloration.29 Proebstle et al28 initially reported comprehensive findings from 6-month data showing a low rate of side effects: ecchymosis 6.4%, paresthesia 3.2%, hyperpigmentation 2.0%, hematoma 1.6%, erythema 1.6%, and phlebitis 0.8%. Thermal skin injury and DVT were not observed in the trial28; however, during the first year of use of the ClosureFAST in 2007, numerous adverse events related to DVT were reported to the U.S. Food and Drug Administration (FDA).35 The FDA MAUDE Adverse Event Database contains 15 reports of DVT in 2007 from use of a ClosureFAST catheter for endothermal ablation.35 In addition, four PEs were reported along with one skin burn.34 It is unclear at this point whether the segmental ablation technique poses an increased risk for DVT over the original ClosurePLUS device. A 4-year prospective study of the ClosurePLUS catheter by Merchant et al33 revealed that 4 of 858 limbs (0.5%) had localized thrombus formation in the SFJ that did not extend more than 10% into the common femoral vein, as well as another 4 of 858 limbs (0.5%) in which DVT developed. Conversely, a 2004 study by Hingorani et al36 reported that DVT subsequently developed in 16% of limbs treated with the ClosurePLUS catheter.
The European Closure Fast Clinical Study Group reported on the 3-year European follow-up on the ClosureFAST device.37 The incidence of complications was low, the majority of which manifested and subsided within the first week of treatment. During the first week of treatment the following complications occurred: ecchymosis 5.8%, erythema 2.0%, hematoma 1.4%, and phlebitis 1.0%. Complications that persisted beyond the first week included paresthesias at an initial rate of 3.4% that declined to 0.4% at 36 months, and pigmentation at an initial rate of 2.4% that also declined to 0.4% at 36 months. There were no reported cases of DVT or PE.
Clinical Results
In addition to low complication rates, early data for ClosureFAST have demonstrated a high degree of efficacy during its first year of use. Before U.S. approval, an initial trial in Europe demonstrated a 100% success rate in 223 patients 6 months after the procedure31 and a 96.7% success rate at 1 year.38 The initial U.S. data used the Kaplan-Meier method to determine efficacy; ultrasonography at 3 days, 3 months, and 6 months showed successful closure in 99.6% of limbs.28 The results of an additional multicenter prospective study, presented by Dietzek,39 indicated a 96.2% vein occlusion rate 1 year after treatment. This result is comparable to that of several 1-year Closure studies, which have yielded success rates ranging from 86% to 99%.33,40–42 A large-scale study of the original ClosurePLUS device by Merchant et al33 demonstrated 2-, 3-, and 4-year efficacy rates of 86.2%, 84.2%, and 88.8%, respectively.
Once again, the European Closure Fast Clinical Study Group reported on the mid-term efficacy of the ClosureFAST device.37 The probability of continued occlusion of the treated truncal vein at 3 years was 92.6%. In addition to anatomic procedural success, the 3-year midterm analysis evaluated other surrogate variables in order to assess clinical efficacy. For example, the Venous Clinical Severity Score (VCSS) improved from an average of 3.9 before treatment to an average of 0.9 at 3 months after treatment (P < .0001), and the low average Venous Clinical Severity Score persisted for the duration of the study. With regards to the clinical component of the Clinical, Anatomic, Etiologic, Pathophysiologic classification system, 74.1% of patients demonstrated an improvement at 36 months. Substratification of the clinical score did demonstrate a differential in the clinical results such that patients with stages C3 and C4 disease accounted for 46% of the patient population before treatment but for only 8% of the population at the conclusion of the study. The proportion of patients with C2 disease dropped to less than 10% at 1 year; however, there was a linear increase such that at 3 years, 33.3% of the patients exhibited C2 disease.
Endovenous Laser Ablation
ELA has many similarities to RFA, with the primary differences related to the catheter and mechanism used to ablate the vein. ELA uses a bare-tipped or jacket-tipped fiber to deliver laser energy to a target area and form thermal energy within the vein lumen.43,44 The thermal energy generates heat and steam bubbles within the lumen of the target vessel, destroying the endothelial lining of the vessel. This causes an inflammatory reaction resulting in a thrombotic occlusion that effectively closes off the vein and eventually leads to fibrosis.43,45
Laser Wavelengths
Numerous different endovenous laser wavelengths have been used to generate endothelial destruction of varicose veins, including 810, 940, 980, 1064, 1319, 1320, and 1470 nm.46 Since the advent of endovenous lasers, several theories centered around the efficacy of wavelengths absorbed by water and hemoglobin have been offered. Research on the optical properties of blood has shown that different wavelengths have variable absorption characteristics47:
• The 810-nm wavelength is specific for hemoglobin absorption.48
• The 940-nm wavelength provides a balanced ratio between the light irradiated into tissue and its absorption by hemoglobin and water.49
• The 980-nm wavelength is specific for hemoglobin and water.48
• The 1319/1320-nm wavelength is specific for water absorption, and it targets collagen in the vein wall.47,50
• The 1470-nm wavelength features an absorption coefficient in water that is 40 times greater than that of the 810- and 980-nm wavelengths.51
A limited number of studies have been conducted to evaluate these various wavelengths for both efficacy and adverse events. The first, published by Proebstle et al52 in 2005, compared the 940- and 1320-nm wavelengths. The study concluded that the wavelengths were equally efficacious but that ecchymosis and pain occurred significantly less often in limbs treated with the 1320-nm laser.52 In a similar study, an analysis of the 810-nm wavelength versus the 980-nm wavelength also favored the higher wavelength.48 There was no significant difference in success of treatment between the two wavelengths; however, at 1-week and 4-month follow-up, less bruising and lower postprocedure pain intensity were noted with the 980-nm wavelength.48 Mackey et al53 performed a study comparing the 810-nm wavelength and the 1320-nm wavelength, with follow-up 3 days after the procedure. They reported analogous findings and concluded that the 1320-nm wavelength was associated with less postoperative pain and bruising. Mauriello et al54 performed a retrospective review in 50 patients treated using the 1470-nm wavelength, which demonstrated treatment efficacy with low rates of pain and bruising. Current research suggests that the various laser wavelengths are likely equally efficacious, but there is a trend toward less pain and bruising with the higher-wavelength lasers.
Preoperative Planning
Preoperative planning for ELA is identical to that for RFA.
Endovenous Laser Ablation Procedure
As with RFA, for the treatment of the GSV, the target access site is at or slightly below the popliteal area. Experienced physicians may attempt to gain access lower on the leg if the vein exhibits reflux distally; however, doing so adds risk to the procedure because the saphenous nerve lies adjacent to the GSV within the saphenous compartment in the lower part of the leg. Ablating the GSV in the lower leg region may increase the risk for paresthesia as a result of the proximity of the saphenous nerve. A micro-access set (21-gauge needle, 0.018-inch wire, and microsheath) is used to initiate access.48 An 18-gauge needle or a cutdown is also effective but not preferred because of the increased risk for trauma to the vessel or venospasm. Once the microsheath is inserted into the GSV, the distance from the access site to the SFJ is measured. The inner dilator of the microsheath is removed and a 0.035-inch guide wire is advanced through the microsheath to the SFJ under ultrasound guidance. The microsheath is removed and a long sheath is selected on the basis of the measured distance from the SFJ to the access site. The sheath selected, most commonly 45 cm in length, is exchanged over the guide wire to a point just distal to the superficial epigastric vein.48 Once in place, the inner dilator and guide wire are removed and a 600-µm bare-tipped or jacket-tipped fiber is advanced into the sheath. The fiber is then connected to the generator and the aiming beam turned on to help visualize the fiber tip under the skin. While the fiber is held in place, the sheath is withdrawn to the locking mechanism of the fiber and locked in place, with the tip of the fiber exposed approximately 2 cm past the end of the sheath. Positioning is reconfirmed with ultrasound, and preparation is made to administer tumescent anesthesia. As with RFA, tumescent anesthesia is injected perivenously48,55,56 with the goal to create a target diameter of fluid around the vein of 10 mm. Positioning of the laser fiber tip is then reconfirmed before the procedure is commenced.
Procedure Variations.
As the endovenous laser procedure has progressed and improved over the past few years, several variations in method have developed. A majority of the variations are related to catheter withdrawal time and the amount of energy delivered. After the continuous mode replaced the pulsed mode as the preferred mechanism, the initial pullback rates for the laser fiber were 3 mm/sec (18 cm/min).55 Another popular protocol was a pullback rate of 1 cm every 3 to 5 seconds, which equates to a relatively variable 12 to 20 cm/min. Accurately gauging the pullback rate was challenging because the first sheaths did not contain any type of dashes or marks. Pullback speed was estimated by an assistant calling out increments of time so that the physician could use best judgment. In 2003, AngioDynamics, Inc. (Queensbury, NY), introduced the first marked sheath, and many companies followed suit. With markings every centimeter on the sheath, the user is able to judge the rate of pullback with precision. Many of the sheaths available today also feature smaller, 1-mm marks at the distal tip to alert the user that the tip of the sheath is being approached.
From an available range of 1 to 15 W, early users of ELA advocated a relatively high treatment setting of 14 to 15 W.43,55 As data were published, several different opinions about the most suitable wattage developed. Some supported the use of lower wattage, in the 10- to 12-W range,48 whereas others set the wattage according to the diameter of the vein.57 In 2004, Timperman et al58 approached the procedure from a different perspective, evaluating the amount of energy (joules) delivered per centimeter of vein treated, otherwise known as the linear endovenous energy density (LEED).52 They found a direct correlation between the LEED and treatment efficacy. The mean energy delivered for successfully treated veins was 63.4 ± 26.6 J/cm, whereas in failed treatments, an average of 46.6 ± 13.8 J/cm was emitted.58 Further solidifying the study, no failures occurred in limbs receiving energy doses of 80 J/cm or greater.58 In a subsequent study by Timperman,59 veins treated with an average of 95 J ± 16 J/cm had a treatment success rate of 91% 9 months after the procedure. In an attempt to assess the efficacy of treatment using higher LEEDs tempered by the increased risk of complications associated with higher energy use, an evaluation of the 1470 nm laser was performed comparing an average LEED higher than 100 J/cm to LEED lower than 100 J/cm. Although the treatment was technically successful 100% of the time in patients treated using an LEED greater than 100 J/cm, there was an increased incidence of paresthesias in the group receiving the higher LEED (15.5% vs. 2.3% P < .05). In addition, postprocedural analgesia intake was significantly less in the lower LEED group.60 Currently, 50 to 80 J/cm is the average treatment energy most often used for ELA procedures.48,61,62 Continuous pullback is used while the user watches the real-time energy readout on the generator and gauges speed with the 1-cm marks on the sheath. This method has proved to be more consistent than using time to gauge pullback because the same amount of energy is delivered in each case, regardless of wattage.
Once tip positioning is confirmed, the laser is switched from standby to ready mode and the foot pedal is depressed to deliver energy. While the preferred method is used to gauge pullback, the laser fiber and sheath are drawn through the vessel simultaneously in a continuous fashion. To prevent skin burns or trauma to the entry site, the user stops treatment by removing the foot from the pedal when the tip of the laser fiber is approximately 1 to 3 cm above the entry site.55,59 Closure of the vein is visualized with duplex ultrasound, followed by removal of the fiber and sheath.
Discharge and Follow-up
Discharge and follow-up instructions for ELA are identical to those for RFA.
Complications
The incidence of procedure-related complications with ELA has been relatively low with the use of endovenous lasers in the continuous mode. Potential complications include but are not limited to vessel perforation, thrombosis, PE, phlebitis, hematoma, infection, skin pigmentation, neovascularization, paresthesia, complications from tumescent anesthesia, irradiation of nontarget tissue, hemorrhage, necrosis, skin burns, and pain.62 Min et al55 published results of the first large-scale study (499 limbs) using a laser with an 810-nm wavelength and noted that 24% of treated patients experienced bruising; notably absent were any cases of skin burns, paresthesia, and DVT. Another broad study using the 810-nm laser reported the following complications: DVT 0.3%, hematoma 0.3%, superficial phlebitis 1.8%, and local transient paresthesia 3.0%.63 Similarly, Kim and Paxton64 observed no DVT, PE, skin burns, or permanent nerve injury in their prospective study using a 980-nm laser. Minor complications in this study included hematoma at the entry site 0.3%, thrombophlebitis 0.3%, and ecchymosis 27%. With regard to postprocedural complications, the rates vary widely in the literature: DVTs 0% to 5.7%, skin burns less than 1%, nerve injury 0% to 22%, and superficial thrombophlebitis 0% to 25%.65,66 A rare complication that has been reported in both RFA- and ELA-treated patients is the development of an arteriovenous fistula, commonly occurring where the external pudendal artery crosses posterior to the GSV.67 The natural history of these arteriovenous fistulae is still being elucidated, but the majority of patients remain asymptomatic.
As discussed earlier, some evidence suggests that higher-wavelength lasers produce less pain and bruising; however, a prospective, large-scale study evaluating all the wavelengths is needed to further substantiate these initial findings. The mechanism of the ELA procedure that causes pain and bruising remains unclear, although some experts speculate that the complications are due to perforation of the vein wall by laser energy.52,68 Aside from laser wavelengths, a novel adaptation to laser fibers that has shown a beneficial effect on perforation of the vein wall is the newly introduced jacket-tipped fibers. This technology features either a stainless steel or a ceramic jacket that completely covers the tip of the fiber, with the end of the tip being recessed within the jacket.69,70 This design prevents the flat emitting face of the fiber tip from coming in contact with the vein wall.69,70 Kabnick et al68 carried out a pilot study to evaluate the efficacy and complications of jacket-tipped fibers versus bare-tipped fibers. At 72 hours after the procedure, both treatment groups demonstrated 100% success; however, in the group treated by jacket-tipped fiber, pain and bruising scores were lower.68 Because vessel perforation has been directly linked to bruising,52 the study concluded that the lower bruising scores achieved in the jacket-tipped fiber group could be attributed to fewer perforations.68 In order to assess the use of a jacket-tipped fiber in a higher-wavelength laser, Maurins et al71 evaluated a 1470-nm laser and compared treatments using a bare-tipped fiber, a jacket-tipped fiber, and a radial-emitting tip fiber. All demonstrated similar efficacy in ablation with corresponding reductions in vein diameter. It turned out that the radial-emitting fiber had the lowest LEED requirement, resulting in less postprocedural pain than in the other two groups.
Clinical Results
In addition to the low rate of complications demonstrated by ELA, treatment outcomes and success rates of ELA are at least equivalent to those of all other modalities. The paramount trial for establishing ELA as an efficacious treatment was published by Min et al55 in 2003. Initial treatment results with an 810-nm laser showed a 98.2% success rate and an ensuing closure rate of 93.4% at 2 years.55 Since this study, numerous trials have been conducted for all the wavelengths currently used. Proebstle et al52 performed a study evaluating 1320- and 940-nm (15- and 30-W) lasers and reported favorable efficacy rates at 3 months of 90.3% (940 nm, 15 W), 100% (940 nm, 30 W), and 97% (1320 nm).52 A large-scale prospective trial involving the use of a 980-nm laser on 500 limbs was published in 2007.61 A 98% closure rate was reported at the 1-week follow-up and remained constant; closure rate was 97.1% at the 4-year follow-up.61
More recently, the International Endovenous Laser Working Group evaluated long-term outcomes using ELA for the treatment of patients with truncal reflux. The international registry evaluated 1020 limbs that were treated using a 980-nm bare-tip laser. The durability of the procedure was confirmed, given that the treatment success rates were 92.3% at 1 year and 86.9% at 2 years with no additional treatment failures at 3 years.72 As the data indicate, ELA for the treatment of venous reflux is a well-tolerated, efficacious, and durable treatment option.
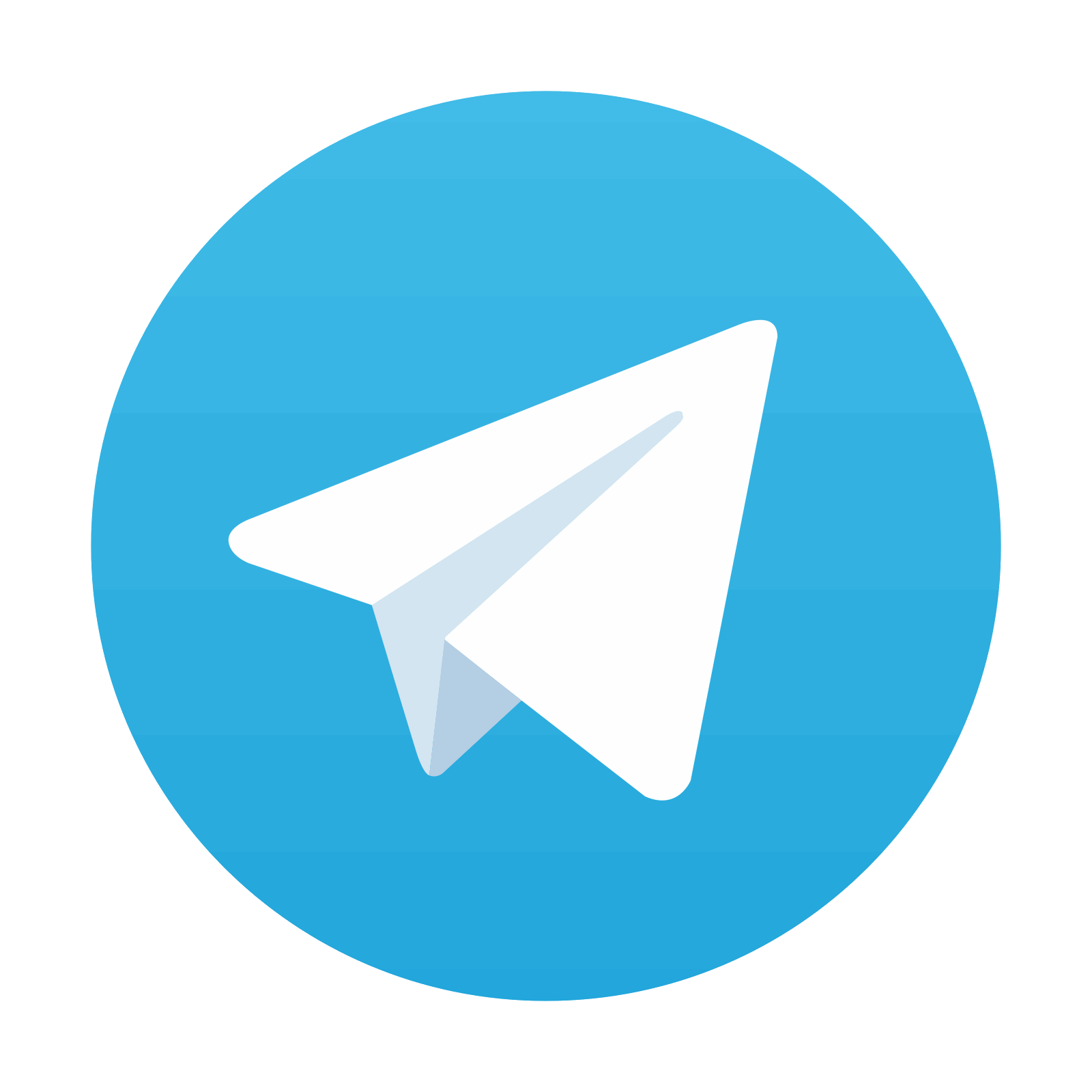
Stay updated, free articles. Join our Telegram channel

Full access? Get Clinical Tree
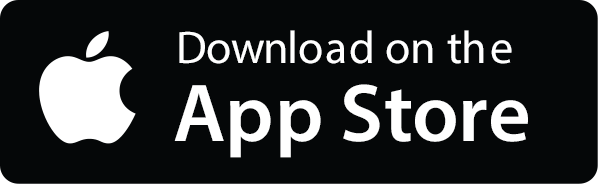
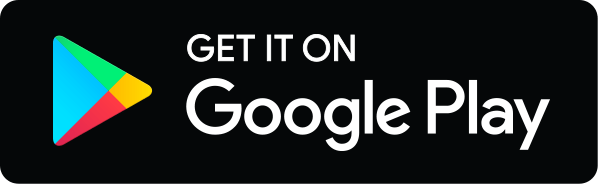