Background
The aim of this study was to investigate the value of three-dimensional speckle-tracking echocardiography for the detection of subclinical left ventricular dysfunction in patients with aortic valvular disease (AVD).
Methods
Fifty-nine patients with AVD in New York Heart Association functional class I or II as well as 48 controls were recruited. Patients with AVD were divided further into those with aortic stenosis (AS; n = 34) and those with aortic regurgitation (AR; n = 25). All patients underwent conventional echocardiography and three-dimensional speckle-tracking echocardiography.
Results
Analysis of variance showed global longitudinal strain to be compromised in the AR group (−16.9% vs −19.3%, P = .015) and more dramatically decreased in the AS group (−14.3% vs −19.3%, P < .001) compared with healthy controls. Impairment of global circumferential strain was observed in patients with AR (−15.9% vs −18.5%, P = .009) but not in those with AS (−18.2% vs −18.5%, P = .768). Global area strain and global radial strain were decreased in patients with AS and those with AR compared with controls, but the difference between the two subgroups was not significant.
Conclusions
Strains measured by three-dimensional speckle-tracking echocardiography are useful indices of early-stage heart dysfunction caused by AVD. Longitudinal strain is more vulnerable to pressure overload caused by AS, whereas circumferential strain is more sensitive to volume overload due to AR.
Aortic valve disease (AVD) is a major cause of heart failure and cardiac death. Patients with AVD undergo extensive left ventricular (LV) remodeling, which can lead to hypertrophy and dysfunction of the left ventricle. LV ejection fraction (LVEF) is one of the most important prognostic factors in patients with AVD. However, LV dysfunction as evidenced by decreased LVEF usually occurs at a relatively late stage. Thus, establishing a more sensitive index for early-stage LV dysfunction is of great importance.
Accumulating evidence has shown that speckle-tracking provides sensitive detection of subclinical cardiac dysfunction. Recent studies have suggested that two-dimensional (2D) speckle-tracking can be used to detect subtle LV systolic dysfunction in patients with AVD with normal LVEFs. However, there are several inherent shortcomings of conventional 2D speckle-tracking: long examination times, geometric assumption of LV morphology, and mistracking if speckles move out of the scanning plane.
Recently, three-dimensional (3D) speckle-tracking echocardiography (STE) has been developed. Instead of artificially dividing the myocardial displacement vector into three directions, 3D STE tracks genuine 3D motion of the acoustic speckles. As a result, 3D STE is free of geometric assumptions and speckles’ moving out of the scanning plane. In addition, 3D STE is less time-consuming than conventional 2D STE. Three-dimensional STE provides accurate and convenient assessment of LV function and has been shown to be useful in the detection of subclinical LV dysfunction in patients with early-stage heart failure and hypertension. In the present study, we aimed to investigate the value of 3D STE for the detection of early-stage LV dysfunction in patients with AVD.
Methods
Seventy-five patients with moderate to severe AVD in New York Heart Association functional class I or II were recruited. Sixteen patients were excluded because of concomitant AVD or impairment of the mitral valve ( n = 5), LVEF < 50% ( n = 5), irregular arrhythmia ( n = 1), or poor acoustic windows ( n = 5). Ultimately, 59 patients were enrolled. To investigate if pressure and volume overload had different effects on LV deformation, patients with AVD were divided further into those with aortic stenosis (AS; n = 34) and those with aortic regurgitation (AR; n = 25) according to the most predominant defect of the aortic valve. Forty-eight age-matched healthy controls with good acoustic windows were also recruited. All patients underwent conventional echocardiography and real-time 3D echocardiography. Three-dimensional strain analyses were undertaken using 4D Auto LVQ software (Vivid E9; GE Vingmed Ultrasound AS, Horten, Norway).
The study protocol was approved by the Ethics Committee of Sichuan University (Sichuan, China). Written informed consent was obtained from all participants.
Conventional Echocardiography
Conventional echocardiography was conducted using a commercially available ultrasound diagnostic system (Vivid E9). AS severity was determined using the mean pressure gradient calculated from the jet velocity using Bernoulli’s equation. A mean gradient > 40 mm Hg was considered to reflect “severe AS,” whereas a gradient of 25 to 40 mm Hg was classified as “moderate AS.” The severity of AR was determined using the vena contracta width method, with vena contracta width ≥ 0.7 cm considered to be severe AR and width between 0.3 and 0.69 cm classified as moderate AR. LVEF was measured using the biplane Simpson’s method using images acquired in the apical four-chamber and apical two-chamber views. LV mass was measured using 3D speckle-tracking echocardiographic analysis software.
Real-Time 3D Echocardiographic Analyses
Real-time 3D echocardiographic full-volume images were recorded using a 4V (GE Vingmed Ultrasound AS) 3D probe in the apical view. To achieve optimal imaging quality for subsequent 3D speckle-tracking echocardiographic analyses, the volume rate was set to ≥40% of the heart rate (mean, 32.8 Hz). The full-volume image was stitched from six subvolumes acquired in six consecutive cardiac cycles. Patients were told to hold their breath during image acquisition. Three-dimensional speckle-tracking echocardiographic analyses were undertaken using 4D Auto LVQ software. The procedure for 3D strain analysis is described in Figure 1 . On 3D STE, all strain parameters were measured simultaneously to allow direct comparison between different strains.

Statistical Analyses
All statistical analyses were undertaken using SPSS version 17.0 (SPSS, Inc, Chicago, IL). Continuous data are expressed as mean ± SD. Independent-samples t tests were used for comparisons between the AVD and control groups. In the subgroup analyses, one-way analysis of variance was used to compare the clinical and echocardiographic parameters among groups. Categorical data were compared using χ 2 tests.
To test the dependency of real-time 3D echocardiographic strains on both preload (AR) and afterload (AS), the relationship between the strain parameters and LV end-diastolic volume (LVEDV) in patients with AR and relative wall thickness (RWT) in patients with AS were evaluated using linear regression analyses.
To evaluate the interobserver variability of 3D speckle-tracking echocardiographic measurements of deformation parameters, image acquisition and analysis in all subjects were repeated by a second sonographer the same day. The second analysis was performed independently, blinded to the previous measurements. The absolute difference between the two measurements was calculated and expressed as mean ± SD. Percentage interobserver variability was calculated as the absolute difference divided by the average of the two measurements. Agreement between the repeated measurements was assessed using Bland-Altman analyses and intraclass correlation coefficients. In all analyses, P values < .05 were considered statistically significant.
Results
Baseline Clinical and Echocardiographic Characteristics
The baseline clinical and echocardiographic characteristics of all participants are summarized in Table 1 . The independent-sample t test showed the AVD group to have significantly higher LVEDVs (113.4 vs 88.8 mL, P < .001), LV mass indices (122.2 vs 82.2 g/m 2 , P < .001), and RWTs (0.46 vs 0.37, P < .001) than the control group. To ascertain if there was a differential impact of pressure and volume overload on LV function, patients with AVD were divided further into those with AS (mean pressure gradient, 53.7 ± 22.9 mm Hg; 23 patients with severe stenosis, 11 patients with moderate stenosis) and those with AR (vena contracta width, 0.63 ± 0.16 cm; 9 patients with severe regurgitation, 16 patients with moderate regurgitation). In general, patients with AVD had higher LVEDVs, and the most obvious change was discovered in patients with AR. The LV mass index was significantly increased in the two subgroups, but there was no significant difference between the AS and AR groups. LVEFs were preserved in the AS and AR groups, but the difference was not significant. RWTs were obviously increased in the AS subgroup and slightly diminished in the AR subgroup compared with controls. Age, sex, blood pressure, and heart rate were comparable between those groups ( P > .05).
Variable | Control ( n = 48) | AVD ( n = 59) | AS ( n = 34) | AR ( n = 25) |
---|---|---|---|---|
Age (y) | 50.7 ± 15.8 | 53.7 ± 15.3 | 55.6 ± 16.7 | 51.7 ± 13.7 |
Men/women | 26/22 | 33/26 | 19/15 | 14/11 |
Systolic BP (mm Hg) | 122.1 ± 14.6 | 121.5 ± 7.8 | 120.9 ± 7.3 | 122.1 ± 8.4 |
Diastolic BP (mm Hg) | 79.4 ± 9.7 | 78.3 ± 4.3 | 78.5 ± 4.6 | 77.9 ± 4.1 |
HR (beats/min) | 69.7 ± 10.9 | 71.6 ± 11.9 | 70.9 ± 12.5 | 72.2 ± 11.7 |
Mean pressure gradient (mm Hg) | NA | NA | 53.7 ± 22.9 | NA |
VC width (cm) | NA | NA | NA | 0.63 ± 0.16 |
LVEDV (mL) | 88.8 ± 20.4 | 113.4 ± 39.9 ∗ | 105.3 ± 32.4 ∗ | 124.8 ± 46.8 ∗,† |
LV mass index (g/m 2 ) | 82.2 ± 13.7 | 122.2 ± 32.1 ∗ | 123.8 ± 33.8 ∗ | 119.9 ± 30.3 ∗ |
LVEF (%) | 62.2 ± 6.5 | 58.9 ± 7.2 ∗ | 59.1 ± 7.5 ∗ | 58.7 ± 6.9 ∗ |
RWT | 0.37 ± 0.03 | 0.46 ± 0.16 ∗,† | 0.58 ± 0.09 ∗ | 0.29 ± 0.04 ∗,† |
Impairment of Real-Time 3D Echocardiographic Strain Parameters in Patients with AVD
Global longitudinal strain (GLS; −15.4% vs −19.3%, P < .001), global radial strain (GRS; 46.0% vs 54.0%, P = .004) and global area strain (−28.2% vs −33.3%, P = .004) were significantly impaired in the AVD group ( Table 2 ). However, a significant difference was not observed in global circumferential strain (GCS) between the AVD and control groups (−17.2% vs −18.5%, P = .151). Differential impairment of strain parameters in the AS and AR groups is also summarized in Table 2 . GLS was significantly lower in the AS and AR groups, but the most appreciable difference was seen in the AS group. Impaired GCS was observed only in patients with AR. Global area strain and GRS were lower in the AS and AR groups compared with healthy controls, but the difference between the two subgroups was not significantly different.
Strain (%) | Control ( n = 48) | AVD ( n = 59) | AS ( n = 34) | AR ( n = 25) |
---|---|---|---|---|
GLS | −19.3 ± 3.3 | −15.4 ± 4.4 ∗ | −14.3 ± 4.1 ∗ | −16.9 ± 4.4 ∗,† |
GCS | −18.5 ± 3.0 | −17.2 ± 4.5 | −18.2 ± 4.5 | −15.9 ± 4.1 ∗,† |
GAS | −33.3 ± 4.4 | −28.2 ± 10.1 ∗ | −29.1 ± 6.2 ∗ | −27.0 ± 13.7 ∗ |
GRS | 54.0 ± 13.0 | 46.0 ± 13.8 ∗ | 46.1 ± 13.8 ∗ | 45.9 ± 14.1 ∗ |
Relationship between the Real-Time 3D Echocardiographic Strain Parameters and LVEDV in Patients with AR and RWT in Patients with AS
Table 3 showed the correlations between strain parameters and LVEDV in patients with AR. Among all four strains, GCS had the highest correlation with LVEDV ( Figure 2 ). In contrast, in patients with AS, GLS had the best correlation with RWT ( Figure 3 ).
Strain | r | P |
---|---|---|
LVEDV (patients with AR) | ||
GLS | 0.41 | .02 |
GCS | 0.81 | <.001 |
GAS | 0.70 | <.001 |
GRS | −0.65 | <.001 |
RWT (patients with AS) | ||
GLS | 0.62 | <.001 |
GCS | 0.22 | .11 |
GAS | 0.46 | .003 |
GRS | −0.43 | .006 |
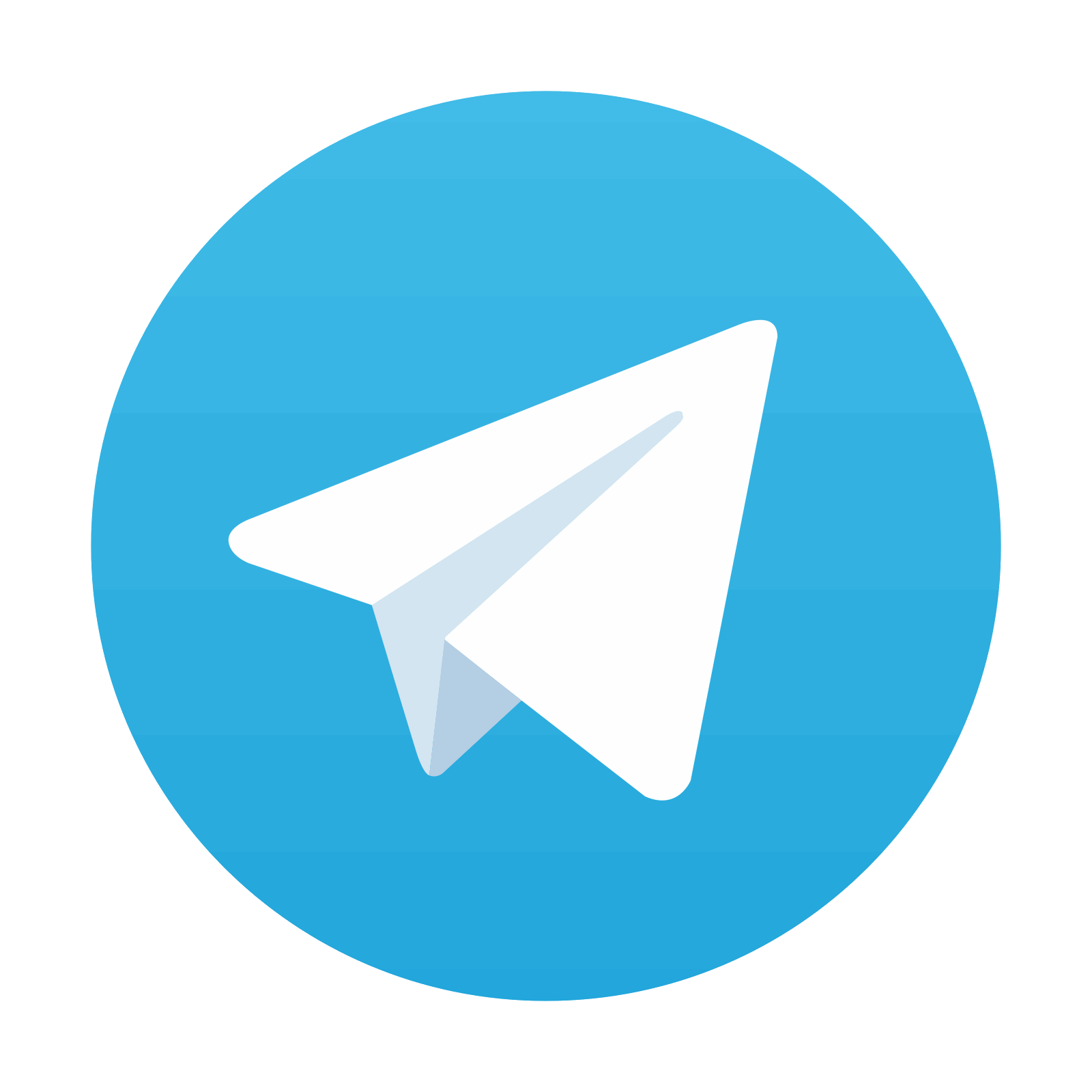
Stay updated, free articles. Join our Telegram channel

Full access? Get Clinical Tree
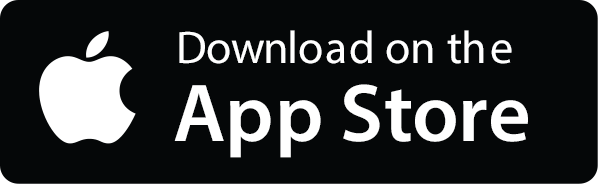
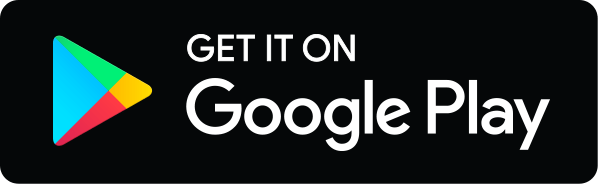
