Background
The reproducibility of echocardiographic measurements of myocardial strain, performed in a community-based setting, has not been reported previously.
Methods
The reproducibility of left ventricular strain measurements was examined in two samples of 20 participants each from the Offspring Cohort of the Framingham Heart Study (mean age, 63 ± 9 years; 59% women). Two-dimensional speckle-tracking-based measurements of global peak left ventricular strain in systole were performed in the apical four-chamber, apical two-chamber, and midventricular parasternal short-axis views.
Results
Interobserver intraclass correlation coefficients were ≥0.84 for all global strain measurements, with average coefficients of variation of ≤4% for global longitudinal and circumferential strain and <8% for global transverse and radial strain. For left ventricular strain measurements performed in each of the three views, intraobserver intraclass correlation coefficients were ≥0.91 among time points spanning a total 8-month period. The average coefficients of variation were <6% for global longitudinal and circumferential strain and <9% for global transverse and radial strain. Interobserver and intraobserver reproducibility findings were similar in analyses adjusting for frame rate.
Conclusions
Excellent reproducibility of global longitudinal and circumferential strain measurements and very good reproducibility of global transverse and radial strain measurements were observed. Taken together, these findings demonstrate the reproducibility of performing echocardiographic strain measurements in a large, epidemiologic community-based setting.
Noninvasive assessments of left ventricular (LV) systolic function have traditionally relied on the measurement of fractional shortening or, more commonly, estimations of ejection fraction. However, it is well known that both ejection fraction and fractional shortening have limited sensitivity for characterizing subclinical abnormalities in LV systolic function. Therefore, advanced image analysis techniques have been developed and validated to assess cardiac tissue deformation in multiple planes and, in turn, provide a more sensitive measure of LV performance. When applied to two-dimensional echocardiography, speckle-tracking-based measures of LV deformation (i.e., myocardial tissue strains) have been shown to add incremental prognostic information beyond ejection fraction in a variety of settings, including clinical centers and trial cohorts of patients with overt heart failure. Prior studies have examined the reproducibility of echocardiography-based LV strain measures in predominantly small-sized or referral samples. Accordingly, comprehensive data regarding the reproducibility of LV strain measures in a community-based setting are lacking. Therefore, the present study was designed to evaluate the reproducibility of speckle-tracking-based LV strain measures in the Offspring Cohort of the Framingham Heart Study.
Methods
Study Sample
The selection criteria and sample selection for the Framingham Offspring Study have been described previously. From 2005 to 2008, a total of 2,888 study participants attended their eighth examination cycle and underwent routine M-mode, two-dimensional, and pulsed-wave Doppler echocardiography as part of the standardized Framingham Heart Study clinic visit, using an HP Sonos 5500 ultrasound machine (Philips Medical Systems, Andover, MA). Images were acquired according to a protocol that did not specify any procedures for optimizing images for speckle-tracking analyses. Because the ability to perform strain-based image measurements is dependent on image quality, all digital images were reviewed for appropriateness for speckle-tracking-based strain analyses. A total of 2,844 echocardiographic studies were considered appropriate for strain-based image measurements on the basis of the following criteria: images were excluded if more than one segment of dropout was observed for any of the predefined two-dimensional views (apical two-chamber [A2C], apical four-chamber (A4C), and parasternal short-axis (SAX) views at the level of the midventricle) or if the presence of any arrhythmia (e.g., atrial fibrillation, premature atrial or ventricular contractions) was noted during image capture.
From the larger pool of echocardiographic studies deemed appropriate for speckle-tracking-based strain analyses, two separate sets of 20 studies were randomly selected for reproducibility analyses. The selection of studies for reproducibility was performed by investigators blinded to clinical information. Consecutive echocardiographic studies were reviewed for appropriateness for reproducibility on the basis of the criteria described above, in addition to visualization of the endocardial border in at least five of the six anatomic segments throughout the cardiac cycle in at least two of the three predefined views for each echocardiographic study: A2C view (basal anterior, mid anterior, apical anterior, basal inferior, mid inferior, and apical inferior wall segments), A4C view (basal anterolateral, mid anterolateral, apical lateral, basal inferoseptal, mid inferoseptal, and apical septal wall segments), and parasternal SAX view at the midventricular level (mid anteroseptal, mid anterior, mid anterolateral, mid inferolateral, mid inferior, and mid inferoseptal wall segments). As part of a general quality assessment performed before the selection of images for strain analyses (at the time of image acquisition), and using a simplified ordinal scale (including four grades, ranging from inadequate to good), the total set of echocardiographic studies selected for reproducibility analyses included 21% that were considered to have good quality two-dimensional images of the left ventricle, 69% fair quality, and 10% poor quality.
All strain measurements were performed by observers blinded to measures performed previously and/or by other observers. The first set of 20 consecutive studies was designated for performing baseline reproducibility analyses, and the second set of 20 consecutive studies was designated for performing follow-up reproducibility analyses over the course of 8 months. We used two separate sets of 20 studies to minimize reader recognition of tracings and values (despite being blinded to identifiers) over the course of this reproducibility study. The first set of 20 studies was used to assess interobserver reproducibility at baseline, and the second set of 20 studies was used to assess intraobserver reproducibility at three separate occasions over the follow-up period.
All participants provided informed consent before attending the examination cycle, and the Institutional Review Board of Boston University Medical Center approved the study protocols.
Strain Analyses
Using an offline analysis program (2D Cardiac Performance Analysis version 1.1, TomTec Imaging Systems GmbH, Unterschleissheim, Germany), previously validated speckle-tracking-based analyses of LV function were performed according to a standardized protocol. We selected this software package (2D Cardiac Performance Analysis) because it allows application to images acquired by any type of echocardiographic machine and has been shown in prior studies to produce strain values that are comparable with those obtained using other frequently used software packages. After a 3-month period of training, analyses were performed by three sonographers (E.O., B.T.L., and P.S.) in each of the three predefined two-dimensional views: A2C, A4C, and midventricular parasternal SAX views. The training program involved initial practice on a training set of only good-quality images, followed by practice on a training set that included good-quality, fair-quality, and poor-quality images. The standardized measurement protocol prespecified that tracings be performed to optimize myocardial tracking first and foremost and generation of appropriate strain curves only secondarily. Several tracing techniques were used to optimize myocardial tracking, including (1) performing traces in the earliest frame with the most optimal visualization of the endocardial border across the maximal number of anatomic segments (this frame was most often end-diastolic for the apical views and most often midsystolic for the SAX view), (2) ensuring that the width between endocardial and epicardial tracking lines was not inappropriately narrow or wide at any point in the cardiac cycle, and (3) anchoring basal portions of tracings to the mitral annulus in the apical views, to prevent inappropriate “straying” of tracing lines over the cardiac cycle.
The primary measurements of interest included the following LV peak systolic strains: global longitudinal and transverse strain in the A2C view, global longitudinal and transverse strain in the A4C view, and global circumferential and radial strain in the SAX view. Figure 1 shows representative examples of strain curves generated by performing image analyses in each of the three views. Frame rates for all analyses were ≥30 frames/sec and not >70 frames/sec for all views: mean, 38 ± 5 frames/sec for A2C images; mean, 40 ± 7 frames/sec for A4C images; and mean, 39 ± 2 frames/sec for SAX images.

Secondary measurements of interest included the regional basal, midwall, and apical strains assessed in the longitudinal views. As in prior investigations, basal regional strain was calculated as the average of basal peak strains measured in the A2C (basal anterior and basal inferior) and A4C (basal anterolateral and basal inferoseptal) views, midwall regional strain was calculated as the average of midwall peak strains measured in the A2C (mid anterior and mid inferior) and A4C (mid anterolateral and mid inferoseptal) views, and apical regional strain was calculated as the average of apical peak strains measured in the A2C (distal anterior and distal inferior) and A4C (distal lateral and distal septal) views.
Reproducibility Analyses
Reproducibility analyses were performed in two stages over the course of the total measurement period of 12 months ( Figure 2 ). In the first stage, baseline interreader reproducibility analyses were performed on the first set of 20 echocardiographic studies. During this first stage, each reader performed measurements in all three views for each of the 20 studies. Interobserver variability for strain measurements was assessed among the three readers for all measurements in all three views. In the second stage of follow-up measurements, we conducted formal intrareader reproducibility analyses using a second set of 20 echocardiographic studies. For this second set of echocardiographic studies, each observer performed repeated measurements for the same view: reader 1 for the A4C view, reader 2 for the A2C view, and reader 3 for the SAX view. Measurements were repeated on three separate occasions: the first and second measurements were performed 2 months apart, and the second and third measurements were performed 6 months apart ( Figure 2 ). Intraobserver variability for strain measurements was assessed among occasions (i.e., time points of measurements) for each reader.

Statistical Analysis
We used several different statistical methods to analyze measurement reproducibility and the sources of variability. In primary analyses, we calculated intraclass correlation coefficients (ICCs) for each strain measure, where each ICC represents the consistency of measurements made by all three readers (for interobserver analyses) or at all three occasions (for intraobserver analyses). We also calculated coefficients of variation for each strain measure, where each coefficient of variation represents variability of measurements among all three readers for interobserver analyses or among all three occasions for intraobserver analyses. For each strain measure, we also tested the equality of measurement means between readers for interobserver analyses and between occasions (i.e., time points) for intrareader analyses, using linear mixed models with fixed effects for reader and for occasion, respectively.
In secondary analyses, we also performed pairwise analyses of interobserver and intraobserver reproducibility and plotted pairwise differences in strain measurements against pairwise average values in Bland-Altman plots. Because frame rate has been reported to potentially influence reproducibility of strain measurements, particularly at low values of frame rate, we repeated interobserver and intraobserver reproducibility analyses adjusting for frame rate. We also repeated analyses adjusting for systolic blood pressure and LV mass, considered surrogates of hemodynamic load. The main reproducibility analyses performed for measures of global strain were also repeated for measures of regional strain, as defined above.
All analyses were performed using SAS version 9.3 (SAS Institute Inc., Cary, NC), and a two-tailed Bonferroni-adjusted P -value threshold of .01 (.05/6) was considered statistically significant.
Results
The participants whose echocardiographic images were selected for inclusion in the present study were middle-aged to older adults with an average age of 63.4 ± 9.2 years, and 59% were women. The mean systolic and diastolic blood pressures of the participants were 124 ± 19 and 73 ± 9 mm Hg, respectively; overall, 28% were hypertensive, 13% were smokers, 10% were obese, none had diabetes, and 3% had prevalent cardiovascular disease.
Interobserver Reproducibility
The interobserver reproducibility of global strain measures at baseline for each of the three readers and each of the three views is shown in Table 1 . The average coefficients of variation were ≤4% for the longitudinal and circumferential strain measures and <8% for the global transverse and radial measures. The interobserver ICCs were ≥0.84 for all analyzed measures. Mean values of global strain were not significantly different among readers for measurements in any of the three views. In pairwise analyses, coefficients of variation were also better for longitudinal and circumferential strains than for transverse and radial strains ( Supplementary Table 1 ; available at www.onlinejase.com ). Figure 3 displays the results of Bland-Altman analyses for all global strain measurements in pairwise interobserver comparisons. Although interobserver reproducibility was similar across the range of strain values in the study sample ( Figure 3 ), agreement between observers tended to be best for the worst values of strain (i.e., least negative values of longitudinal and circumferential strains and least positive values of transverse and radial strains). Notably, the range of values in our sample was largest for radial and transverse strains and smallest for longitudinal strains.
Global strain measure | Measurement (%) | Within-subject CV | Overall ICC | Tests of equal means among readers, P value ∗ |
---|---|---|---|---|
A2C longitudinal strain | −23.5 ± 2.4 | 3.3 ± 1.9 | 0.89 | .15 |
A2C transverse strain | 31.6 ± 7.2 | 6.0 ± 2.6 | 0.91 | .42 |
A4C longitudinal strain | −22.7 ± 2.3 | 4.0 ± 1.9 | 0.84 | .04 |
A4C transverse strain | 32.1 ± 7.5 | 7.3 ± 5.0 | 0.89 | .67 |
SAX circumferential strain | −31.2 ± 3.3 | 3.0 ± 2.2 | 0.90 | .03 |
SAX radial strain | 43.6 ± 8.2 | 4.7 ± 8.0 | 0.91 | .85 |
∗ P values < .01 are considered statistically significant, accounting for multiple tests.
Intraobserver Reproducibility
The intraobserver reproducibility of strain measures performed by the three readers over the follow-up period is shown in Table 2 . Results of analyzing intraobserver reproducibility of global strain measures at baseline were similar (data not shown). Over the follow-up period, the intraobserver coefficients of variation were ≤6% for the global longitudinal and circumferential strain measures and <9% for the global transverse and radial strain measures. For all analyzed measures, intraobserver ICCs were ≥0.91. Mean values of global strain were not significantly different among occasions for measurements performed by any of the three readers. In pairwise analyses, coefficients of variation were also better for longitudinal and circumferential strains than for transverse and radial strains ( Supplementary Table 2 ; available at www.onlinejase.com ). Figure 4 shows Bland-Altman analyses for intraobserver comparisons of global strain measurements performed at different occasions. Although intraobserver reproducibility was similar across the range of strain values in the study sample ( Figure 4 ), reproducibility tended to be best for the worst values of strain (i.e., least negative values of longitudinal and circumferential strains and least positive values of transverse and radial strains). Accordingly, as shown in Figure 5 , mean (and standard deviation) of global strain measurements performed was stable over the course of the measurement period.
Global strain measure | Measurement (%) | Within-subject CV | Overall ICC | Tests of equal means among occasions, P value ∗ |
---|---|---|---|---|
A2C longitudinal strain | −21.6 ± 5.1 | 6.0 ± 3.2 | 0.92 | .12 |
A2C transverse strain | 30.8 ± 6.6 | 5.8 ± 2.9 | 0.91 | .81 |
A4C longitudinal strain | −21.6 ± 4.6 | 5.0 ± 3.0 | 0.92 | .95 |
A4C transverse strain | 32.0 ± 9.2 | 6.7 ± 4.7 | 0.93 | .07 |
SAX circumferential strain | −33.8 ± 9.3 | 3.8 ± 2.4 | 0.98 | .69 |
SAX radial strain | 48.7 ± 20.3 | 8.2 ± 6.8 | 0.95 | .91 |
∗ P values < .01 are considered statistically significant, accounting for multiple tests.
Secondary Analyses
Results of interobserver and intraobserver reproducibility analyses of global strain measures were not substantially different after adjusting for frame rate ( Supplementary Tables 3 and 4 ; available at www.onlinejase.com ). Interobserver and intraobserver reproducibility results were also similar in analyses adjusting for systolic blood pressure and LV mass, considered as surrogate measures of hemodynamic load (data not shown). We also performed interobserver and intraobserver reproducibility analyses for regional measures of basal, midwall, and apical regional strain ( Tables 3 and 4 ). As reflected by coefficients of variation and ICCs, reproducibility was better for midwall regional strain measures than for apical and basal strain measures. Measurements of midwall regional strain were the most reproducible, with coefficients of variation <7% between readers and between occasions. Overall, mean values of regional strain were not significantly different among readers or among occasions for measurements performed by any of the three readers.
Regional strain measure | Measurement (%) | Within-subject CV | Overall ICC | Tests of equal means among readers, P value ∗ |
---|---|---|---|---|
Basal longitudinal strain | −23.6 ± 3.4 | 8.5 ± 5.6 | 0.62 | .05 |
Midwall longitudinal strain | −19.8 ± 2.2 | 6.3 ± 3.5 | 0.67 | .91 |
Apical longitudinal strain | −26.0 ± 2.2 | 9.0 ± 5.2 | 0.23 | .04 |
Basal transverse strain | 35.6 ± 7.4 | 11.1 ± 8.8 | 0.69 | .64 |
Midwall transverse strain | 31.6 ± 6.6 | 6.3 ± 4.3 | 0.86 | .43 |
Apical transverse strain | 28.3 ± 6.9 | 13.1 ± 7.4 | 0.72 | .70 |
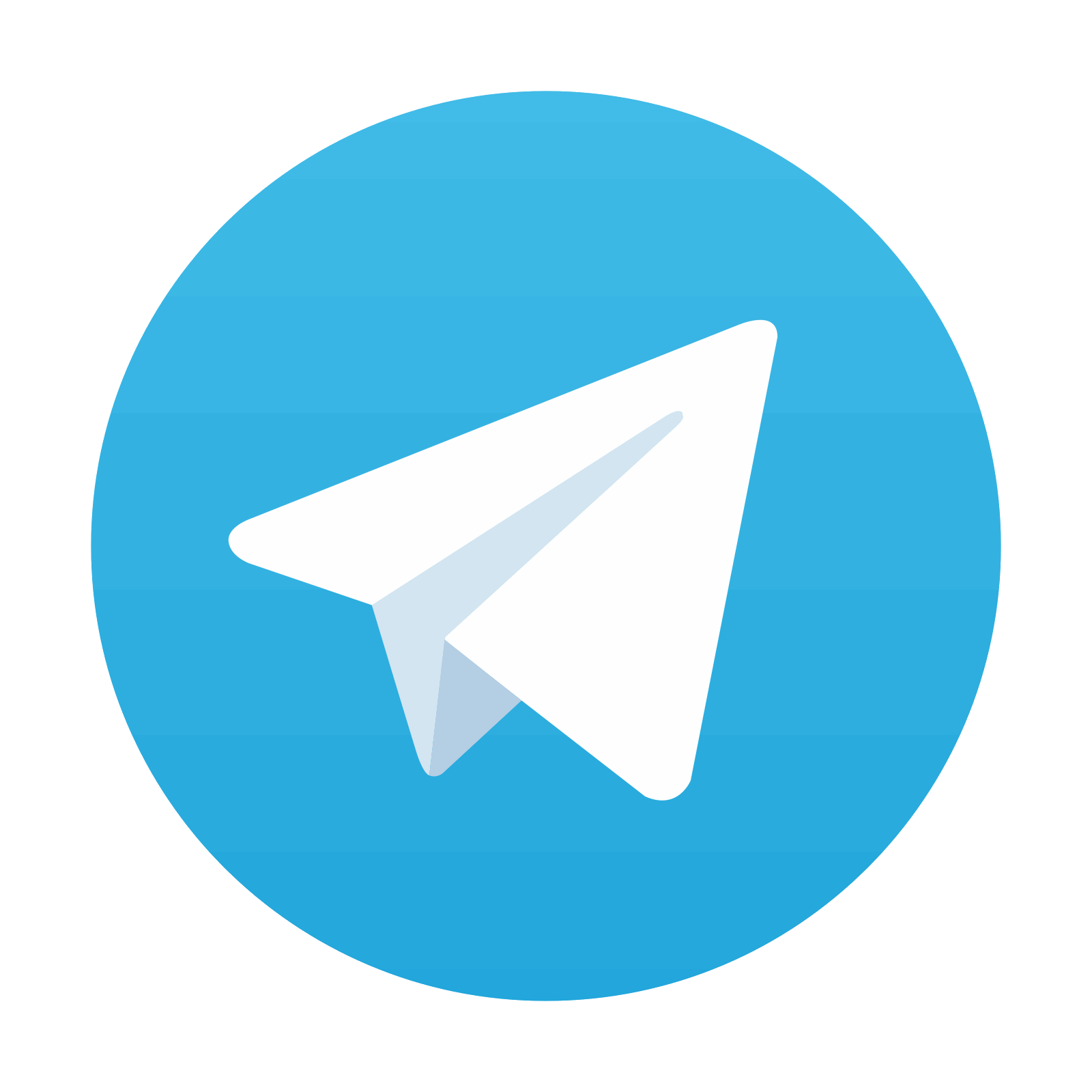
Stay updated, free articles. Join our Telegram channel

Full access? Get Clinical Tree
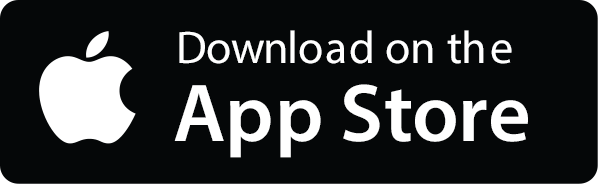
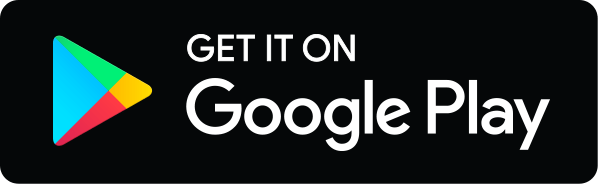
