Background
The accuracy of echocardiographic parameters of right ventricular (RV) function has not been sufficiently validated in patients with pulmonary hypertension (PH). The aim of this study was to evaluate whether echocardiographic measurements reliably reflect RV systolic function in PH using cardiac magnetic resonance imaging (CMRI)–derived RV ejection fraction (RVEF) as a gold standard.
Methods
A total of 37 consecutive patients with PH, 20 with pulmonary arterial hypertension, 12 with chronic thromboembolic PH, and five others, were prospectively studied. All patients underwent echocardiography, CMRI, and right-heart catheterization within a 1-week interval. Associations between five echocardiography-derived parameters of RV systolic function and CMRI-derived RVEF were evaluated.
Results
All five echocardiography-derived parameters were significantly correlated with CMRI-derived RVEF (percentage RV fractional shortening: r = 0.48, P = .0011; percentage RV area change: r = 0.40, P = .0083; tricuspid annular plane systolic excursion [TAPSE]: r = 0.86, P < .0001; RV myocardial performance index: r = −0.59, P < .0001; and systolic lateral tricuspid annular motion velocity: r = 0.63, P < .0001). Compared with the other indices, TAPSE exhibited the highest correlation coefficient. Of the five echocardiographic measurements, only TAPSE significantly predicted CMRI-derived RVEF in multiple regression analysis ( P < .0001). Intraobserver and interobserver reproducibility was favorable for all five indices and was particularly high for TAPSE and systolic lateral tricuspid annular motion velocity.
Conclusions
Echocardiographic measurements are promising noninvasive indices of RV systolic function in patients with PH. In particular, TAPSE is superior to other indices in accuracy.
Pulmonary hypertension (PH) is defined as a mean pulmonary artery pressure > 25 mm Hg at rest. PH imposes pressure overload on the right ventricle through elevated pulmonary artery pressure, which can cause premature death in advanced cases. Precise evaluation of right ventricular (RV) function is thus critical in the management of patients with PH, although complex RV chamber geometry limits the accurate assessment of RV function.
Recently, however, progress in cardiac magnetic resonance imaging (CMRI) has enabled the accurate calculation of RV ejection fraction (RVEF) in patients with PH. However, quantitative assessment of RV function using CMRI requires a dedicated program and expertise, which limits its widespread application and use.
In contrast, echocardiography is a convenient and readily accessible tool for the evaluation of RV function. To date, several echocardiographic parameters have been introduced for the assessment of RV function. However, their accuracy has been tested mostly in patients with non-PH diseases or in control subjects. Moreover, a gold standard with sufficient accuracy was not applied in most studies, hampering their broad use as reliable indices of RV function. In addition, no studies have systematically compared the accuracy and reproducibility of these echocardiographic indices. Accordingly, in the present study, we attempted to validate whether currently available echocardiographic measurements reliably reflect RV systolic function in patients with PH using CMRI-derived RVEF as a gold standard. We also sought to determine which echocardiographic measurement is superior to other indices in terms of accuracy.
Methods
Patients with PH were prospectively enrolled between April 2010 and February 2011. A mean pulmonary artery pressure ≥ 25 mm Hg and pulmonary wedge pressure ≤ 15 mm Hg evaluated by right-heart catheterization (RHC) were used as entry criteria. Exclusion criteria were (1) inability to obtain or analyze images from CMRI or echocardiography for any reason, (2) comorbid left-heart diseases that may affect RV geometry and function, and (3) signs or symptoms indicative of an unstable condition of PH. All enrollees underwent echocardiography, CMRI, and RHC within 1 week.
Echocardiography was performed using the Vivid q system (GE Healthcare, Milwaukee, WI), and images were analyzed offline after the procedure. A two-dimensional apical four-chamber view was used to obtain percentage RV fractional shortening (%RVFS) and percentage RV area change. An established method was used to calculate both indices. Briefly, %RVFS was obtained by calculating regional changes of the distance from the interventricular septum to the RV free wall at the mid-RV level between end-diastole and end-systole ( Figure 1 A). Percentage RV area change was calculated by dividing the difference in RV area between the end-diastolic and end-systolic phases by end-diastolic RV area. The endomyocardial contour was traced from the tricuspid annulus along the free wall to the apex, then back to the annulus, along the interventricular septum. Trabeculation, tricuspid leaflets, and chords were included in the chamber ( Figure 1 B). M-mode images were used to obtain tricuspid annular plane systolic excursion (TAPSE), as has been reported previously. In short, the M-mode cursor was oriented to the junction of the tricuspid valve plane with the RV free wall, and the total displacement of the tricuspid annulus from end-diastole to end-systole was measured ( Figure 1 C). The RV myocardial performance index (MPI) was calculated by subtracting RV outflow velocity time ( b ) from the interval between the cessation and onset of tricuspid flow velocity ( a ), and the difference ( a − b ) was divided by RV outflow velocity time ([ a − b ]/ b ; Figure 1 D). Pulsed-wave Doppler tissue imaging of systolic lateral tricuspid annular motion velocity (TV lat ) was obtained from the apical four-chamber view using a sampling gate of 2 to 4 mm and a sweep velocity of 100 to 150 mm/sec. An ultrasound cursor was carefully placed parallel to the direction of the tricuspid annular motion, and TV lat was measured in Doppler tissue imaging mode using minimal gain and a reduced high-pass filter, minimizing signals from noise ( Figure 1 E). Image acquisition and analysis were performed by a single experienced cardiologist unaware of the CMRI measurements (T.S.).

CMRI studies were performed using a 1.5-T Philips Achieva magnetic resonance imaging system (Philips Medical Systems, Best, The Netherlands) with Master gradients (maximum gradient amplitude, 33 mT/m; maximum slew rate, 100 mT/m/msec). Imaging was performed with subjects in the supine position using a five-element cardiac phased-array coil with breath holding in expiration and with a vector-cardiographic method for electrocardiographic gating. Localizing scans were followed by breath-hold cine imaging in the axial orientation. From the coronal localizing images that demonstrated gross cardiac anatomy, a transverse stack of slices was planned to cover the heart from a level just below the diaphragm to the bronchial bifurcation, covering the heart in diastole. A total of 12 axial slices were acquired using a steady-state free precession pulse sequence (repetition time, 2.8 msec; echo time, 1.4 msec; flip angle, 60°; acquisition matrix size, 192 × 256; field of view, 380 mm; slice thickness, 10 mm; interslice gap, 0 mm; phases per cardiac cycle, 20).
Image analysis of the right and left ventricles was performed offline using commercially available analysis software (Extended MR Work Space version 2.6.3; Philips Medical Systems). Contour tracing was aided by reviewing the multiple-phase scans in movie mode. In the axial data sets, the endocardial contours of the right ventricle were traced manually at the first phase and at the two phases in the middle of the cardiac cycle with the smallest volumes. The workstation selected the first phase of each slice as end-diastole and the smaller of the two middle phases as end-systole. The contours were traced starting at the most apical slice and finishing with the uppermost slice. The RV contours were traced up to the tricuspid valve and closed by a straight line across the tricuspid valve ring. The uppermost slice was defined as the first slice at which a pulmonary cusp could be identified. In this uppermost slice, both in end-diastole and in end-systole, the area of the pulmonary cusp was excluded from the volume. Slices above that level were considered to include the main pulmonary artery and were excluded from the RV volume. The most apical slice was defined as the last slice to contain blood volume. RV and left ventricular (LV) end-diastolic volume and end-systolic volumes were computed. RV and LV stroke volumes were calculated as end-diastolic volume − end-systolic volume and ejection fractions as (stroke volume/end-diastolic volume) × 100%. CMRI analysis was performed by a single experienced radiologist who was unaware of the results of echocardiographic evaluations (N.O.-M.). The same observer (N.O.-M.) repeated the RVEF measurements in 10 randomly selected patients, with ≥2 weeks between measurements, to assess intraobserver variability. A second observer (T.S.) also repeated RVEF measurements in the same 10 patients for assessment of interobserver variability. Bland-Altman analysis and intraclass correlation coefficients (ICCs) between the two measurements were used to assess intraobserver and interobserver reproducibility.
The relationship between echocardiographic measurements and CMRI-derived RVEF was assessed using Pearson’s correlation coefficient. Multiple regression analysis was also conducted to examine if any of the five echocardiographic measurements were associated with CMRI-derived RVEF in an independent manner. Intraobserver agreement for echocardiographic measurements was assessed by comparing the measurements of repeated analysis in 10 randomly chosen patients (T.S.). This repeated analysis was performed 1 to 3 months after the initial assessment. Interobserver agreement was assessed using the same patients ( n = 10) by comparing the results measured by T.S. and by a second experienced cardiologist (I.T.). The second cardiologist was not aware of either the echocardiographic measurements by the first examiner or the CMRI measurements. Bland-Altman analysis and ICCs between the two measurements were used to assess intraobserver and interobserver reproducibility.
All statistical analyses were performed using JMP version 8 (SAS Institute Inc., Cary, NC), and P values < .05 were considered statistically significant. The present study was approved by the ethics committee of the Hokkaido University Graduate School of Medicine, and written informed consent was obtained from all patients.
Results
A total of 39 patients met the entry criteria during the study period, but two were excluded because of prespecified exclusion criteria (inability to undergo CMRI because of claustrophobia in one patient and hypertrophic cardiomyopathy in another). Clinical characteristics and CMRI and RHC measurements of the 37 patients with PH are summarized in Table 1 . Bland-Altman analysis of CMRI-derived RVEF showed high agreement for both intraobserver and interobserver reproducibility (mean ± limits of agreement: intraobserver, 0.73 ± 3.3; interobserver, −1.5 ± 3.2). The ICCs for intraobserver and interobserver reproducibility were 0.99 and 0.98, respectively.
Variable | Value |
---|---|
Diagnosis | |
Pulmonary arterial hypertension ∗ | 20 |
Chronic thromboembolic pulmonary hypertension | 12 |
Other | 5 |
Age (y) | 53 ± 15 |
Men/women | 11/26 |
World Health Organization functional class | |
I | 1 (3%) |
II | 15 (41%) |
III | 16 (43%) |
IV | 5 (14%) |
Use of PH-specific vasodilators † | |
Beraprost | 14 (38%) |
Bosentan | 12 (32%) |
Ambrisentan | 1 (3%) |
Sildenafil | 11 (30%) |
Tadalafil | 1 (3%) |
Epoprostenol | 4 (11%) |
6-min walking distance (m) ‡ | 386 ± 117 |
Brain natriuretic peptide (pg/mL) | 218 ± 395 |
Hemodynamics | |
Systolic systemic blood pressure (mm Hg) | 118 ± 20 |
Diastolic systemic blood pressure (mm Hg) | 67 ± 12 |
Pulmonary capillary wedge pressure (mm Hg) | 8 ± 2 |
Mean pulmonary artery pressure (mm Hg) | 39 ± 10 |
Systolic pulmonary artery pressure (mm Hg) | 64 ± 20 |
Diastolic pulmonary artery pressure (mm Hg) | 24 ± 7 |
Mean right atrium pressure (mm Hg) | 6 ± 2 |
Cardiac index (L/min/m 2 ) § | 2.8 ± 0.8 |
Pulmonary vascular resistance (dyne · s · cm −5 ) | 612 ± 271 |
Mixed venous oxygen saturation (%) ¶ | 68 ± 7 |
CMRI results | |
LV ejection fraction (%) | 59 ± 10 |
LV end-diastolic volume (mL) | 100 ± 33 |
LV end-systolic volume (mL) | 44 ± 26 |
RVEF (%) | 38 ± 11 |
RV end-diastolic volume (mL) | 183 ± 66 |
RV end-systolic volume (mL) | 118 ± 61 |
∗ Eight with idiopathic pulmonary arterial hypertension, eight with connective tissue disease–associated pulmonary arterial hypertension, and four with other diagnoses.
† PH-specific drugs were used in combination in 14 patients (39%).
‡ Not performed in 5 patients who were in World Health Organization functional class IV.
§ Mean of at least three measurements obtained using the thermodilution method.
¶ Obtained during oxygen inhalation (0.5–8 L/min) in seven patients.
Table 2 shows echocardiographic parameters. All five echocardiography-derived parameters were shown to significantly correlate with CMRI-derived RVEF (%RVFS: r = 0.48, P = .0011; percentage RV area change: r = 0.40, P = .0083; TAPSE: r = 0.86, P < .0001, RV MPI: r = −0.59, P < .0001; and TV lat : r = 0.63, P < .0001). Of these echocardiographic indices, TAPSE exhibited the highest correlation coefficient with RVEF ( Figure 2 ). In multiple regression analysis, among the five echocardiographic measurements, only TAPSE significantly predicted CMRI-derived RVEF (%RVFS, P = .12; percentage RV area change, P = .89; TAPSE, P < .0001; RV MPI, P = .74; and TV lat , P = .32).
Variable | Value |
---|---|
LV diastolic diameter (mm) | 41 ± 6 |
LV systolic diameter (mm) | 23 ± 6 |
LV fractional shortening (%) | 43 ± 9 |
Interventricular septal wall thickness (mm) | 8.9 ± 1.6 |
LV posterior wall thickness (mm) | 8.6 ± 2.1 |
Diastolic eccentricity index | 1.3 ± 0.2 |
Systolic eccentricity index | 1.5 ± 0.4 |
LV MPI | 0.41 ± 0.20 |
Tricuspid regurgitation grade (none/mild/moderate/severe) | |
None | 4 |
Mild | 19 |
Moderate | 10 |
Severe | 4 |
Tricuspid regurgitation pressure gradient (mm Hg) ∗ | 65 ± 20 |
%RVFS | 20 ± 8 |
Percentage RV area change † | 31 ± 17 |
TAPSE (mm) | 19 ± 4 |
RV end-diastolic area (cm 2 ) | 21 ± 7 |
RV end-systolic area (cm 2 ) | 15 ± 6 |
RV MPI | 0.51 ± 0.23 |
TV lat (cm/sec) | 9.3 ± 2.4 |
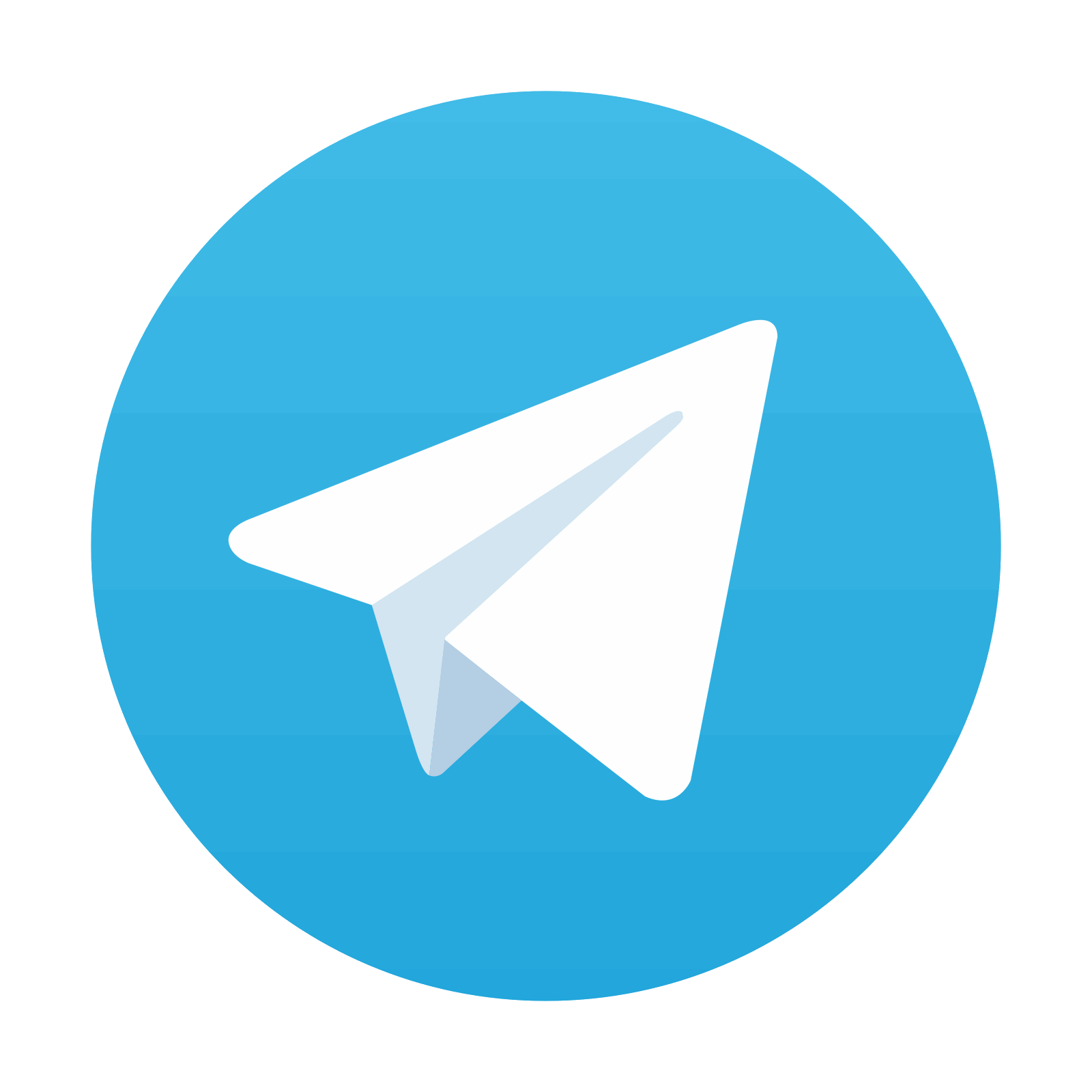
Stay updated, free articles. Join our Telegram channel

Full access? Get Clinical Tree
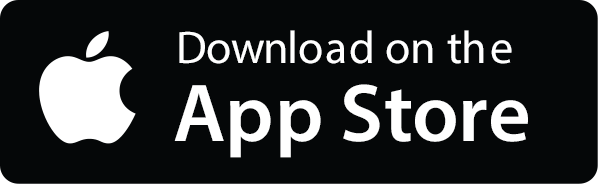
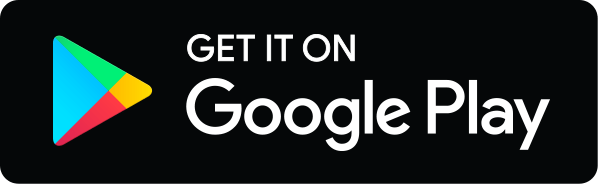
