We hypothesized that the longitudinal changes in peak oxygen uptake, ventilatory efficiency, and exercise-induced right-to-left shunting in patients with pulmonary arterial hypertension (PAH) would predict outcomes better than baseline measurements alone. Patients with PAH die prematurely. Identifying prognostic markers is critical for treating patients with PAH; however, longitudinal prognostic information of PAH is limited. We enrolled 103 patients with PAH into a long-term, prospective outcome study using serial cardiopulmonary exercise testing to measure the peak oxygen uptake, ventilatory efficiency (ratio of ventilation to carbon dioxide output at the anaerobic threshold), right-to-left shunting, and other factors in patients treated with optimal therapy. The patients were followed up for a mean of 4.7 years. During the study period, 20 patients died, and 3 underwent lung transplantation. The baseline peak oxygen uptake and ventilatory efficiency was 0.79 L/min and 49 (normal <34), respectively, reflecting severe disease. Poorer ventilatory efficiency and greater New York Heart Association classification were associated with poor outcome at baseline and at follow-up. On multivariate analysis, the persistence or development of an exercise-induced right-to-left shunt strongly predicted death or transplantation (p <0.0001), independent of the hemodynamics and all other exercise measures, including peak oxygen uptake and ventilatory efficiency. The absence of a shunt at baseline was associated with a 20% rate of nonsurvival, which decreased to 7% at follow-up. A poorer ventilatory efficiency appeared to be associated with a poor outcome in patients without a shunt. In conclusion, a persistent exercise-induced right-to-left shunt and poor ventilatory efficiency were highly predictive of poor outcomes in patients with pulmonary arterial hypertension.
The use of cardiopulmonary exercise testing (CPET) for diagnosing pulmonary vascular disease, to establish pulmonary artery hypertension (PAH) severity, and to demonstrate a response to therapy has been well established. The baseline measurements of aerobic capacity and ventilatory efficiency with CPET have been shown to predict survival in patients with PAH. In addition, creation of a right-to-left shunt by way of atrial septostomy has been used as a means of reducing right atrial pressure and to “decompress” the right ventricle in an attempt to increase cardiac output and increase systemic oxygen transport. In some series, ≥40% of patients have had evidence of right-to-left shunting during exercise, with oxygen desaturation during CPET. Because of the progressive nature of PAH, we hypothesized that serial measurements of aerobic capacity and ventilatory efficiency would predict the outcome better than a single measurement and would be useful in the noninvasive serial assessment of patients with PAH. In addition, we hypothesized that the presence of an exercise-induced right-to-left shunt in our patients would predict better outcomes.
Methods
We prospectively enrolled 103 patients with idiopathic PAH (IPAH), familial PAH, and associated PAH (APAH), as defined by currently accepted diagnostic World Health Organization group I criteria into a long-term, noninvasive outcome study evaluating serial CPET measurements. All patients underwent diagnostic right heart catheterization with measurements of the right atrial, right ventricular, pulmonary arterial, and pulmonary capillary wedge pressure and cardiac output. Patients with disorders other than PAH were excluded according to the recommended diagnostic guidelines for PAH. Most patients were receiving PAH-specific treatment, including endothelin antagonists, phosphodiesterase-5 inhibitors, or prostacyclin analogs.
Patients underwent CPET twice: first at baseline and again after a median of 0.7 year. CPET was performed using cycle ergometry, as previously described. Patients underwent exercise testing using a progressively increasing work rate test to maximum tolerance on an electromagnetically braked cycle ergometer. Gas exchange was measured breath-by-breath using MedGraphics (St. Paul, Minnesota) CPET equipment that recorded the heart rate, ventilation, carbon dioxide output, oxygen uptake, work rate, and end-tidal carbon dioxide and end-tidal oxygen pressure, as well as other gas exchange variables. From these data, the peak oxygen uptake, peak heart rate, peak oxygen pulse, respiratory exchange ratio (which equals carbon dioxide output/oxygen uptake), anaerobic threshold, ventilatory equivalent for carbon dioxide at the anaerobic threshold, and other parameters were calculated, as previously described. The reproducibility for CPET in our laboratory has been previously reported, with coefficients of variation <2.2% for peak oxygen uptake and most other CPET variables.
The presence of exercise-induced right-to-left shunting was determined by an investigator evaluating the CPET studies, as previously described in a similar cohort of patients with PAH. The investigator was unaware of the CPET sequence and the patient’s treatment and clinical course. Each 9-panel CPET plot was reviewed to identify an exercise-induced right-to-left shunt at the start of unloaded cycling exercise or during exercise, using the following criteria: (1) an abrupt and sustained increase in end-tidal oxygen pressure, with (2) a simultaneous, sustained decrease in end-tidal carbon dioxide pressure, (3) an abrupt and sustained increase in the respiratory exchange ratio, (4) an abrupt and sustained increase in ventilation/oxygen uptake, (5) an abrupt and sustained increase in ventilation/carbon dioxide output, and (6) an associated decrease in pulse oximetry.
Each of these criteria was weighted equally, with ≥4 criteria identifying an exercise-induced shunt in each CPET. These criteria were the same (and were evaluated in the same manner) as those used by one of the investigators of the reference method, who was also an investigator in the present study (JEH).
All subjects were treated with conventional and PAH-specific therapies, as needed, during their participation in the study. The New York Heart Association (NYHA) classification was assigned without knowledge of the CPET results.
The primary outcome was the survival time, defined as interval from the first CPET to death by any cause or urgent lung or heart/lung transplantation, and it was analyzed using a prespecified sequence of survival analyses implemented with the nonparametric Cox proportional hazards regression model. Proportionality was tested and verified by assessing the significance of interactions between the survival predictors and survival time. First, the potential effect on survival of each of 12 prespecified CPET measures, weight, body mass index, NYHA classification (class II vs greater than II), and shunt status at baseline were first examined in individual models. Next, the potential effect of change per month from the baseline CPET to the second CPET on survival time was examined in the individual models, and the additional potential effect of CPET changes, independent of the current (second visit) value, for the corresponding CPET measure was assessed. The set of significant (p <0.05) characteristics from these analyses, as well as the peak oxygen uptake and ventilation/peak carbon dioxide output at the anaerobic threshold (which were a priori selected for inclusion because of their clinical relevance and regardless of the statistical significance) were then included in multivariate analyses of survival from visit 2 using the data current at that time.
All available data for the measures included in any particular analysis were used, and no imputation of missing data was performed. Several secondary sensitivity analyses were conducted to assess the robustness of the conclusions from the primary analyses. Those analyses included survival from the second visit, ignoring all baseline visit information; and restriction to the subgroup of subjects with NYHA class greater than II. A p value of <0.05 was considered statistically significant, and no mathematical correction was made for multiple statistical testing.
The present study was conducted in accordance with Good Clinical Practices, the current version of the Declaration of Helsinki, and with local institutional regulations. The local ethics review committee (Human Subjects Committee) approved the protocol, and all patients gave written informed consent. All investigators had full access to the data and take responsibility for its integrity. All investigators have read and agreed to the report as written.
Results
All patients survived at least to their second CPET. The patients were followed up for a mean of 4.7 years after their first CPET. The baseline patient demographics, CPET findings, and invasive hemodynamics are listed in Table 1 . Our population was mostly women, consistent with previously reported cohorts from our institution. The baseline peak oxygen uptake and ratio of ventilation to carbon dioxide output at the anaerobic threshold was 0.79 ± 0.26 L/min and 49 ± 13 (normal <34), respectively, and the pulmonary vascular resistance was 12 ± 6 Wood units, reflecting severe pulmonary vascular disease.
Characteristic | Value |
---|---|
Age (years) | 49 ± 13 |
Gender (n) | |
Women | 91 |
Men | 12 |
Pulmonary arterial hypertension (n) | |
Idiopathic | 63 |
Familial | 2 |
Associated | 38 |
New York Heart Association functional class (n) | |
II | 14 |
III | 85 |
IV | 4 |
Weight (kg) | 73 ± 21 |
Cardiopulmonary exercise testing | |
Peak oxygen consumption (L/min) | 0.79 ± 0.26 |
Ventilatory equivalent for carbon dioxide at anaerobic threshold | 49 ± 13 |
End-tidal carbon dioxide pressure at anaerobic threshold (mm Hg) | 28 ± 6 |
Anaerobic threshold (L/min) | 0.6 ± 0.2 |
Peak work rate (Watts) | 53 ± 26 |
Peak heart rate (beats/min) | 132 ± 20 |
Systolic blood pressure at rest (mm Hg) | 123 ± 17 |
Hemodynamics | |
Right atrial pressure (mm Hg) | 10 ± 5 |
Mean pulmonary arterial pressure (mm Hg) | 54 ± 16 |
Cardiac output (L/min) | 3.7 ± 1.4 |
Pulmonary vascular resistance [(dyne × second) / cm 5 ] | 12 ± 6 |
During the study period, 20 patients died, and 3 underwent lung transplantation, 1 of whom died subsequently. The overall survival rate for the entire patient population was 81% at 5 years (95% confidence interval 0.70 to 0.88). The survival status related to the exercise values at the baseline visit is listed in Table 2 . A greater ratio of ventilation to carbon dioxide output at the anaerobic threshold, lower anaerobic threshold, lower end-tidal carbon dioxide pressure at anaerobic threshold, greater NYHA classification, and marginally lower peak oxygen uptake increased the hazard of nonsurvival. At baseline, the systolic blood pressure neither at rest nor at peak exercise was significantly different between survivors and nonsurvivors. None of the patients with NYHA class II at baseline died. Restricting the analyses for all the other factors to only those with NYHA class greater than II gave results similar to those listed in Table 2 (data not shown). After a median of 0.7 year (range 0.2 to 5.9), the repeat CPET showed similar associations between the ratio of ventilation to carbon dioxide output at the anaerobic threshold, anaerobic threshold, end-tidal carbon dioxide pressure at anaerobic threshold, and NYHA classification (data not shown). For the 17 patients with APAH in the present study, survival was similar to that of patients with IPAH. Also, no difference in survival was found for the patients already receiving PAH-specific treatments at study entry compared to those who were not.
Measure | SD (n = 103) | All (n) | Surviving | Not Surviving | Cox Hazard Ratio ⁎ | 95% Confidence Interval | p Value | ||
---|---|---|---|---|---|---|---|---|---|
Patients (n) | Mean | Patients (n) | Mean | ||||||
Peak oxygen consumption (L/min) | 0.26 | 103 | 80 | 0.82 | 23 | 0.71 | 0.67 | 0.43–1.05 | 0.08 |
Peak oxygen consumption (ml/min/kg) | 2.84 | 103 | 80 | 11.0 | 23 | 11.0 | 0.95 | 0.62–1.45 | 0.80 |
Ventilatory equivalent for carbon dioxide at anaerobic threshold | 12.6 | 103 | 80 | 47.0 | 23 | 54.2 | 1.48 | 1.05–2.09 | 0.03 † |
Peak work rate (Watts) | 25.9 | 96 | 75 | 54.6 | 21 | 47.8 | 0.78 | 0.49–1.24 | 0.30 |
Systolic blood pressure at rest (mm Hg) | 17.4 | 96 | 74 | 123 | 22 | 121 | 0.94 | 0.60–1.47 | 0.79 |
Peak systolic blood pressure (mm Hg) | 27.3 | 97 | 75 | 159 | 22 | 152 | 0.80 | 0.53–1.21 | 0.29 |
Diastolic blood pressure at rest (mm Hg) | 12.4 | 91 | 70 | 76.9 | 21 | 76.4 | 1.04 | 0.68–1.58 | 0.86 |
Peak diastolic blood pressure (mm Hg) | 17.2 | 91 | 70 | 87 | 21 | 82 | 0.94 | 0.60–1.48 | 0.80 |
Anaerobic threshold (L/min) | 0.19 | 90 | 68 | 0.61 | 22 | 0.51 | 0.53 | 0.32–0.90 | 0.02 † |
End-tidal carbon dioxide pressure at anaerobic threshold (mm Hg) | 6.2 | 96 | 77 | 28.5 | 19 | 25.0 | 0.62 | 0.39–0.97 | 0.04 † |
Peak heart rate (beats/min) | 19.5 | 94 | 73 | 132 | 21 | 135 | 1.09 | 0.70–1.71 | 0.69 |
Weight (kg) | 20.6 | 103 | 80 | 75.4 | 23 | 67.1 | 0.72 | 0.46–1.13 | 0.16 |
NYHA Class II Deaths | NYHA Class III–IV Deaths | ||||||||
New York Heart Association functional class (III–IV vs II) | — | 103 | 0/14 (0%) | 23/89 (25.8%) | 0.03 † | ||||
Visit | No shunt deaths | Shunt deaths | |||||||
1 | 103 | 13/64 (20.3%) | 10/39 (25.6%) | 1.71 | 0.74–3.97 | 0.21 | |||
2 | 102 | 5/70 (7.1%) | 18/32 (56.2%) | 10.6 | 3.87–28.8 | <0.0001 † |
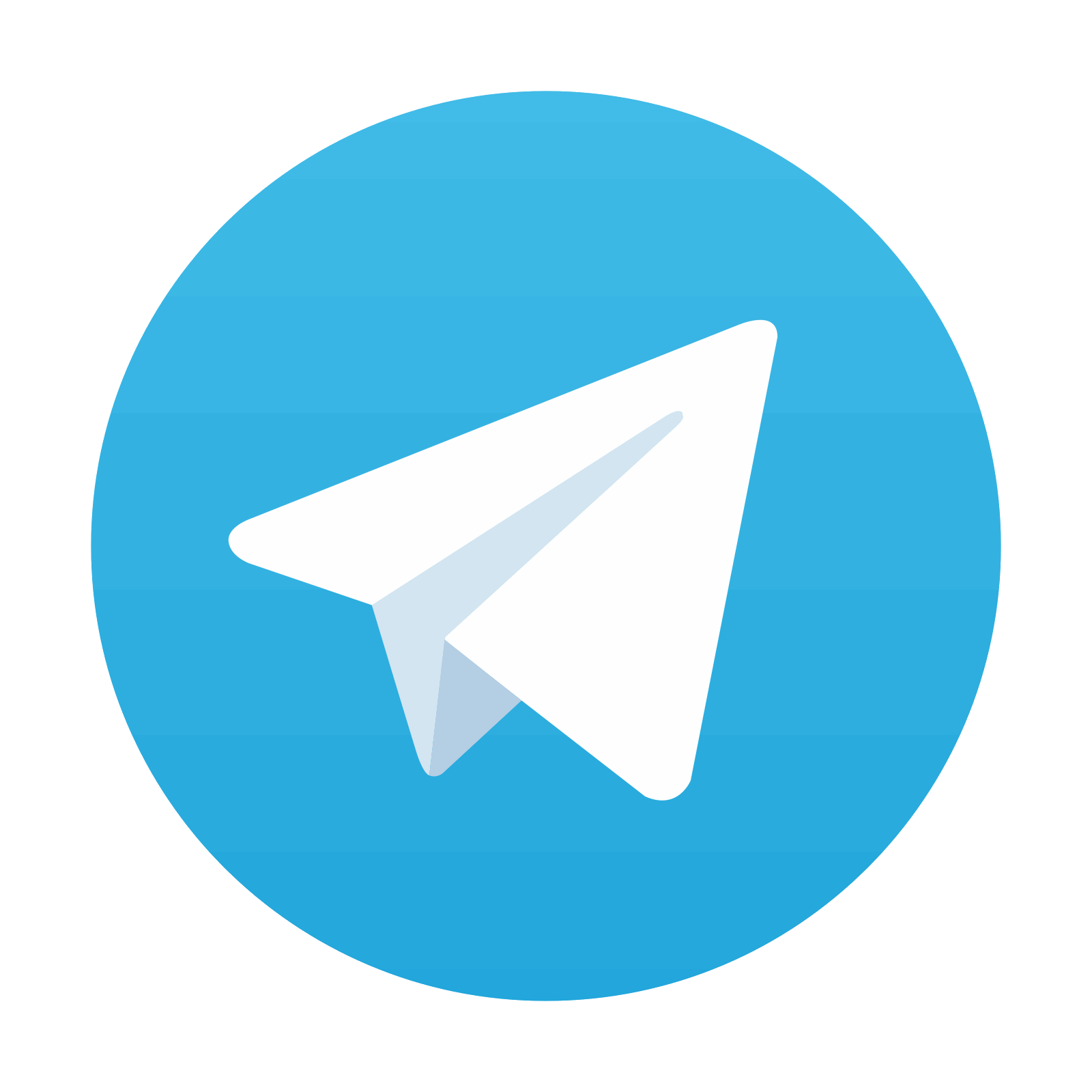
Stay updated, free articles. Join our Telegram channel

Full access? Get Clinical Tree
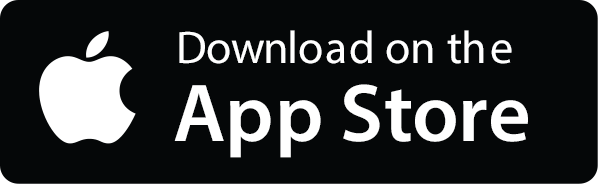
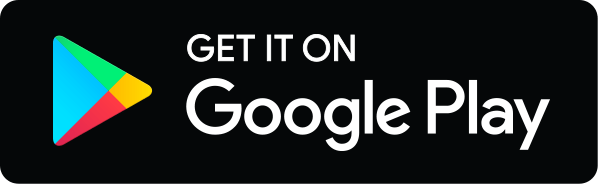
