It is well recognized that ST-segment depression is due to subendocardial ischemia secondary to an increase in left ventricular end-diastolic pressure. The increase in left ventricular end-diastolic pressure is associated with increased left atrial pressure, resulting in left atrial wall distension that contributes to increasing P-wave duration (PWD). The objective of this study was to determine if PWD measured in leads II and V 5 during maximum exercise stress testing could be a reliable predictor of myocardial ischemia. Patients with suspected coronary disease underwent maximum exercise stress testing with myocardial perfusion imaging. PWD was measured using leads II and V 5 at rest and after exercise, with electrocardiographic complexes magnified 4 times (100 mm/s, 40 mm/mV). The change in PWD was calculated as Δ = PWD recovery − PWD rest . ΔPWD and ST-segment changes were related to the absence or presence of ischemia (localized reversible perfusion abnormalities) on myocardial perfusion imaging scans. ΔPWD had sensitivity of 72%, specificity of 82%, negative predictive power (NPP) of 90%, and positive predictive power of 57%. ST-segment change had sensitivity of 34%, specificity of 87%, NPP of 80%, and positive predictive power of 47%. When ΔPWD and ST changes were combined, sensitivity increased to 79% and NPP increased to 91%. In conclusion, ΔPWD outperformed ST-segment changes in predicting myocardial ischemia on myocardial perfusion imaging scans. Furthermore, when ΔPWD and ST-segment changes were combined, sensitivity and NPP were also significantly increased. In this study population, measuring ΔPWD substantially increased the diagnostic value of maximum exercise stress testing.
Exercise stress testing (EST) using the electrocardiogram as an indicator of myocardial ischemia was first introduced by Feil and Siegel in 1928, and >80 years later, the ST segment remains the primary indicator of this process. Subsequent studies have revealed that ischemia involves a reduction in left ventricular compliance that causes an increase in left ventricular end-diastolic pressure and left atrial diastolic pressure. In 1973, Heikkila et al demonstrated that the change in the P wave during electrocardiographic monitoring in the critical care unit could be used as a surrogate for left ventricular filling pressure. The sequence of atrial excitation in the isolated human heart was documented in great detail by Durrer et al. The early third of right atrial P-wave forces are directed anteriorly and predominantly inferiorly, and the latest third of left atrial P-wave forces are directed leftward and posteriorly. Selvester and associates studying atrial physiology and atrial electrocardiology confirmed the time-phase relations of these P-wave vectors. Thus, on 12-lead electrocardiography, P-wave onset is best identified in lead II, and P-wave offset is best identified in lead V 5 . These clinical and electrophysiologic findings led us with Myrianthiefs to measure P-wave duration (PWD) during EST and during balloon inflation for angioplasty. During these studies, there was much difficulty when measuring PWD on median beat recordings, which failed to display significant data before P-wave onset to accurately confirm PWD. Nonetheless, our experience using optical magnifiers suggested that PWD might be more sensitive in predicting myocardial ischemia than ST-segment depression. The increase in left atrial pressure resulting in changes in P-wave morphology has been demonstrated to be predictive of ischemia but has not yet been generally recognized or applied, probably partly because of the difficulty of the measurements. The aim of this study was to test the hypothesis that changes in PWD measured in leads II and V 5 are not only useful but are more reliable in detecting increased left ventricular end-diastolic pressure due to exercise-induced myocardial ischemia than changes in the ST segment.
Methods
We reviewed cases of patients who underwent EST with myocardial perfusion imaging (MPI) from January 2005 to February 2009 at the Memorial Heart and Vascular Institute of Long Beach Memorial Medical Center. Patients had to have completed symptom-limited EST with rest and stress single-photon emission computed tomographic (SPECT) MPI studies (n = 455). Excluded from enrollment were patients aged <25 years (n = 2) and those with atrial fibrillation (n = 10), artificially paced rhythms (n = 8), or excessive motion artifacts on electrocardiography (n = 34). Patients who satisfied these requirements (n = 401) were enrolled in the study. All patients underwent EST using a Mortara X-Scribe version 3.0 (Mortara Instrument Inc., Milwaukee, Wisconsin) or a GE Case version 6.1 (GE Medical Systems, Freiburg, Germany) stress testing system. Patients were continuously monitored by simultaneous 12-lead electrocardiography before and during exercise and until the end of recovery using the Mason-Likar lead system. The 500 samples/s simultaneous 12-lead electrocardiographic digital data were recorded continuously with the 2 systems.
Rest and stress SPECT MPI was performed by the Nuclear Medicine Division of the Department of Radiology. Rest and stress images were acquired after the injection of 23 to 26 mCi technetium-99m tetrofosmin on 2 separate days with the patient supine and the arms above the head. SPECT imaging was performed with a dual-head Siemens e.cam (Siemens Medical Solutions, Knoxville, Tennessee). Sixty projections over 180° for 30 s/projection were acquired in a 64 × 64 matrix with the heads oriented at 90° to each other, using low-energy, high-resolution collimation.
Stress testing was performed using the Ellestad protocol under direct supervision of the attending cardiologist. Exercise was continued for 1 minute after the simultaneous injection of 23 to 26 mCi technetium-99m tetrofosmin. Exercise end points were the achievement of 85% of maximum age-predicted heart rate (220 − age), or higher if tolerated, or chest pain with horizontal or downsloping ST-segment depression or elevation ≥1 mm. Poststress SPECT MPI was performed in the same sequence and using the same parameters as the rest study. The presence of ischemia was determined by the presence of an exercise-induced localized reversible perfusion defect as reported by the interpreting radiologist. All patients with localized reversible perfusion defects, indicative of ischemia, were classified as ischemic (n = 99). Those without reversible perfusion defects were classified as nonischemic (n = 302).
Two subgroups were also created. Patients who completed EST on the GE Case stress system (n = 310) were placed in set A, and those who completed EST on the Mortara X-Scribe stress system (n = 91) were placed in set B. On the 2 systems, the individual median and average beats did not include significant pre-P-wave data to confidently confirm the earliest P-wave onset across the 12-lead electrocardiogram. Thus, the continuous electrocardiographic tracings of set A were reprinted directly from digital storage at 4× magnification (100 mm/s, 40 mm/mV) of standard electrocardiographic tracings (25 mm/s, 10 mm/mV). The 12-lead electrocardiographic tracings, including continuous lead II rhythm strip, of set B were enlarged 4× (100 mm/s, 40 mm/mV) using a photocopier. Each set was analyzed separately selecting the individual P wave with the least signal noise in simultaneous leads II and V 5 , and the results were compared.
PWD was measured at rest and within the first minute of recovery, at a heart rate <140 beats/min, while the patient was standing. Time was allowed to obtain the highest signal-to-noise ratio to aid in PWD measurements. Total atrial excitation time and PWD measurements were performed on 4× magnified tracings. PWD was measured in lead V 5 . The P-wave onset in lead V 5 of the least noisy waveform was confirmed in the simultaneous beat in lead II ( Figure 1 ). At high heart rates, special attention was given to the overlap of the T wave and/or U wave with P-wave onset. To more accurately identify P-wave onset, care was taken to identify the inflection point on the downslope of the T wave and/or U wave that was contiguous with the main body of the P wave ( Figure 1 ). The same attention was given to identify P-wave offset. Interference by the Ta wave, reflective of atrial repolarization, displaces the PQ segment downward from isoelectric baseline. To more accurately identify P-wave offset, care was taken to identify the inflection point at the end of the P-wave as it is merged with the PQ segment ( Figure 1 ). This point has been shown to most accurately identify the termination of the P-wave.
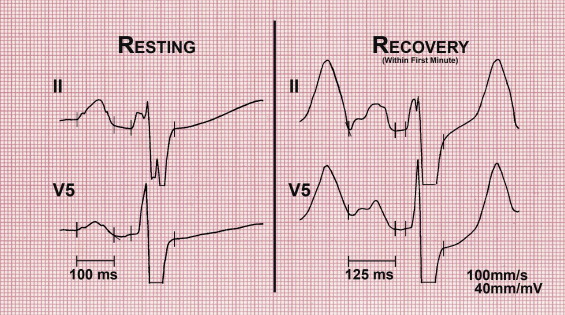
The change in PWD (ΔPWD) was calculated as PWD recovery − PWD rest . PWD was analyzed with the reviewer blinded to MPI and statistical results. The optimal cutoff of 20 ms was established from histograms of PWD in nonischemic and ischemic MPI cases. A ΔPWD ≥20 ms correlated with ischemic MPI cases, and a ΔPWD <20 ms correlated with nonischemic MPI cases. The 20-ms cutoff for ΔPWD was designated to calculate sensitivity, specificity, positive predictive power (PPP), and negative predictive power (NPP).
Sensitivity, specificity, NPP, and PPP for ST-segment change were calculated for comparison. The ST segment was evaluated for changes throughout exercise and recovery, in leads aVR, V 4 , V 5 , and V 6 on standard electrocardiographic tracings (25 mm/s, 10 mm/mV). Patients with ST-segment depression or elevation <1 mm at the J point plus 60 ms correlated with nonischemic MPI cases. Cases with upsloping ST-segment depression, in which the ST segment at the J point plus 80 ms is depressed but 1 mm greater than the J point (at standard gain, 10 mm/mV), also correlated with nonischemic MPI cases. Patients with horizontal or downsloping ST-segment depression or elevation ≥1 mm at the J point plus 60 ms were correlated with ischemic MPI cases. Within the upsloping ST-segment change set (n = 58), sensitivity, specificity, PPP, and NPP were also calculated for ΔPWD.
Demographic data were extracted from patient histories obtained in the exercise stress laboratory. Data were analyzed using SPSS version 15.0 (SPSS, Inc., Chicago, Illinois). Differences in interval and ratio variables and MPI scan results were analyzed using independent Student’s t tests. The level of significance used was 0.05. The relations between demographic and clinical variables and MPI scan results were tested using contingency table analysis and Fisher’s exact test.
Results
Demographic and clinical factors by MPI results are listed in Table 1 . The difference in ΔPWD between nonischemic MPI cases and ischemic MPI cases was statistically significant (p <0.001). The association between demographic data and clinical variables is listed in Table 2 . Men were significantly more likely to have ischemic MPI results (p = 0.010). Patients with reported histories of myocardial infarction, angioplasty, coronary artery bypass grafting, hyperlipidemia, and smoking were significantly associated with ischemic MPI results (p = 0.045, p = 0.011, p = 0.005, p = 0.027, and p = 0.044, respectively). Patients who reported histories of chest pain or reported chest pain on EST were not significantly associated with ischemic MPI scans (p = 0.901 and p = 0.273, respectively). There were no significant differences between the type of magnification, digital magnification (set A) versus photocopier enlargement (set B) of electrocardiograms, and MPI scan results (p = 0.491).
Variable | Ischemic MPI | p Value | |
---|---|---|---|
Yes | No | ||
(n = 302) | (n = 99) | ||
Age (years) | 57.44 ± 10.88 | 61.49 ± 10.97 | 0.001 |
Ejection fraction (%) | 64.34 ± 10.6 | 60 ± 9.58 | <0.001 |
PWD at rest (ms) | 123.03 ± 16.88 | 118.79 ± 16.48 | 0.03 |
PWD during recovery (ms) | 128.23 ± 19.96 | 140.86 ± 19.98 | <0.001 |
ΔPWD (ms) | 5.2 ± 17.68 | 22.07 ± 17.31 | <0.001 |
Variable | Ischemic MPI | p Value | |
---|---|---|---|
Yes | No | ||
(n = 302) | (n = 99) | ||
Men | 163 (54.0%) | 68 (69%) | 0.010 |
Chest pain | 208 (68.9%) | 69 (70%) | 0.901 |
Chest pain during EST | 20 (6.6%) | 10 (10%) | 0.273 |
Hypertension ⁎ | 176 (58.3%) | 60 (61%) | 0.725 |
Diabetes mellitus | 58 (19.2%) | 22 (22%) | 0.562 |
Hyperlipidemia ⁎ | 146 (48.3%) | 61 (62%) | 0.027 |
Previous myocardial infarction | 36 (11.9%) | 20 (20%) | 0.045 |
Previous angioplasty | 66 (21.9%) | 35 (35%) | 0.011 |
Previous coronary bypass | 25 (8.3%) | 19 (19%) | 0.005 |
Previous arrhythmias | 89 (29.5%) | 26 (26%) | 0.609 |
Smokers | 113 (37.4%) | 49 (50%) | 0.044 |
Set B (photocopier enlargement) | 66 (21.9%) | 25 (25%) | 0.491 |
ST-segment change | <0.001 | ||
None | 223 (73.8%) | 48 (49%) | |
Upsloping | 41 (13.6%) | 17 (17%) | |
Horizontal | 33 (10.9%) | 18 (18%) | |
Downsloping | 5 (1.7%) | 15 (15%) | |
Elevation | 0 (0.0%) | 1 (1.0%) | |
ST-segment change abnormal | 38 (12.6%) | 34 (34%) | <0.001 |
ΔPWD ≥20 ms | 54 (17.9%) | 71 (72%) | <0.001 |
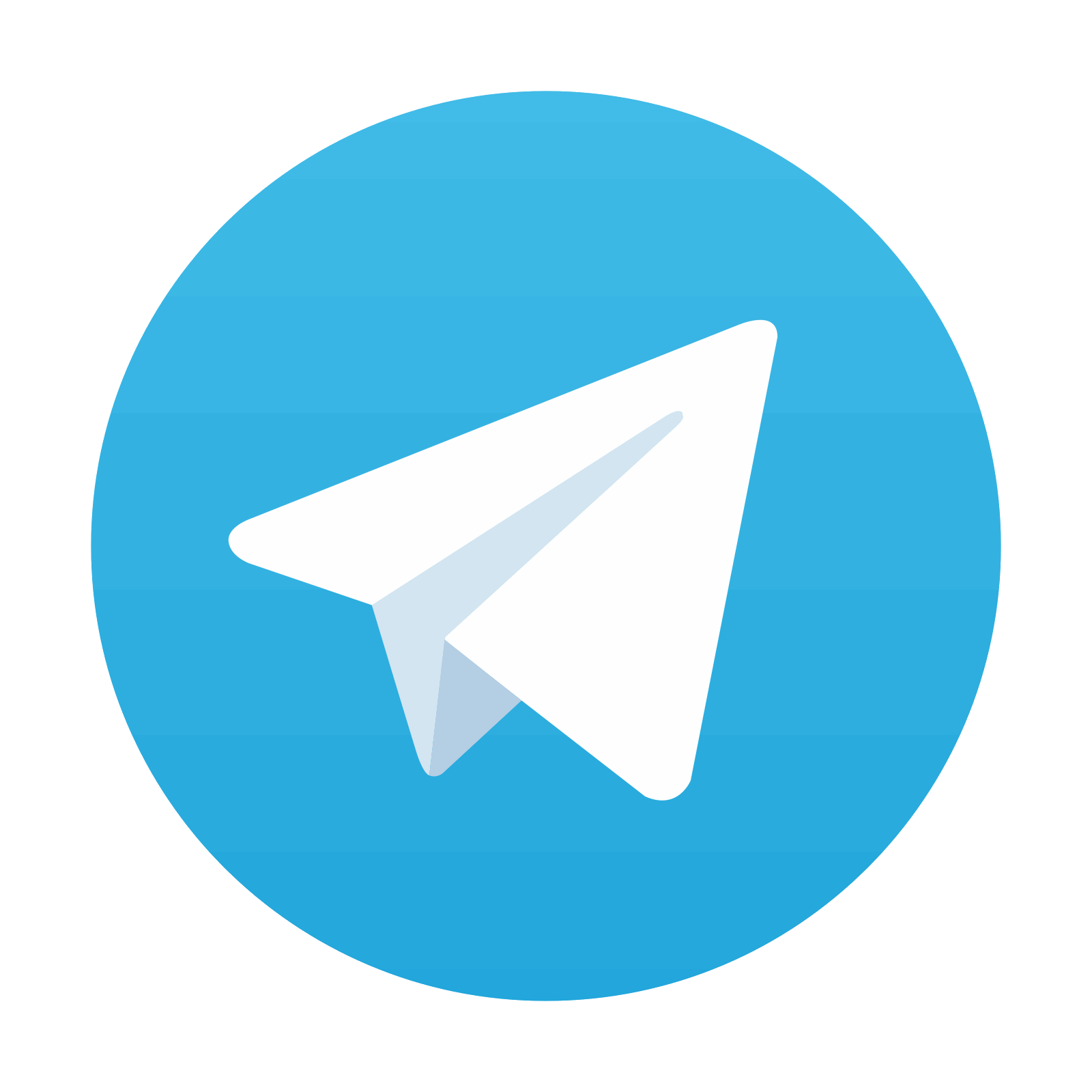
Stay updated, free articles. Join our Telegram channel

Full access? Get Clinical Tree
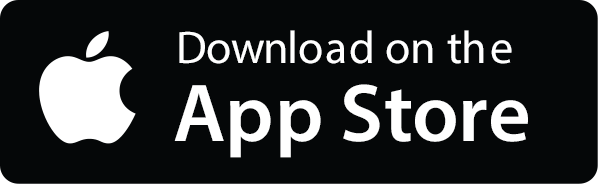
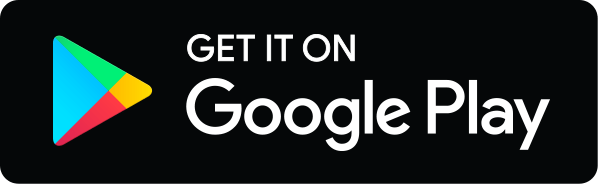
