This study sought to investigate the relation between myocardial perfusion and N-terminal pro-brain natriuretic peptide (NT-proBNP) in asymptomatic adults without overt coronary artery disease. NT-proBNP is a cardiac neurohormone secreted from the ventricles in response to ventricular volume expansion and pressure overload and may also be elevated in the setting of reduced myocardial perfusion. We hypothesized that reduced myocardial perfusion reserve (MPR) would be associated with elevated NT-proBNP in participants free of overt cardiovascular disease. MPR was measured by cardiac magnetic resonance, before and after adenosine infusion, in 184 MESA participants (mean age 60 ± 10.4, 58% white, 42% Hispanic, 44% women) without overt cardiovascular disease. MPR was modeled as hyperemic myocardial blood flow (MBF) adjusted for MBF at rest. A linear regression analysis, adjusted for demographics, established cardiovascular risk factors, left ventricular mass, coronary calcium score, body mass index, and medications, was used to determine the association between MPR and NT-proBNP. Participants with low hyperemic MBF were more likely to be older, male, diabetic, and have higher blood pressure and higher coronary artery calcium score. Mean hyperemic MBF was 3.04 ± 0.829 ml/min/g. MPR was inversely associated with NT-proBNP levels. In a fully adjusted model, every 1-SD decrement in MPR was associated with a 21% increment in NT-proBNP (p = 0.04). In conclusion, MPR is inversely associated with NT-proBNP level in this cross-sectional study of asymptomatic adults free of overt coronary artery disease, suggesting that higher NT-proBNP levels may reflect subclinical myocardial microvascular dysfunction.
To our knowledge, the relation between N-terminal pro-brain natriuretic peptide (NT-proBNP) and myocardial perfusion reserve (MPR) in asymptomatic adults without overt cardiovascular disease has not been described. These findings will be useful in understanding the effect of impaired microvascular structure on neurohormonal levels and may help explain the prognostic implications of elevated BNP in asymptomatic adults. We hypothesized that MPR would be inversely related to serum NT-proBNP level in participants of the Multi-Ethnic Study of Atherosclerosis (MESA).
Methods
MESA is a prospective, multicenter cohort study aimed to determine characteristics related to progression of subclinical to clinical cardiovascular disease. Information regarding the design and scope of the study has been published previously. At baseline, MESA participants were 45 to 84 years old and had no history or coronary artery disease. In a MESA ancillary study, all Minnesota participants (n = 1,066) were contacted immediately after the baseline clinic examination or later by mail in a recruitment effort to obtain cardiac magnetic resonance (CMR) perfusion studies. A total of 234 participants consented to and underwent CMR perfusion studies at the Minnesota field center.
Participants were excluded if they had any of the following: prevalent cardiovascular disease at baseline (n = 1), missing major perfusion measurements (MBF at rest, hyperemic MBF, or perfusion ratio, n = 5), caffeine intake within 12 hours before the CMR scanning (n = 7), missing NT-proBNP measurements (n = 20), or missing any other covariate information (n = 17). After exclusions, 184 participants were included in the final analysis.
CMR studies were performed on a 1.5 T clinical MRI scanner (Sonata 1.5 T; Siemens Medical Systems, Malvern, Pennsylvania). The protocol used has been described in previous publications. Briefly, intravenous access was obtained, and the patient was positioned supine. Vital signs and an electrocardiogram were monitored throughout the examination. Scout MR images were acquired to determine the orientation of the short and long axis of the left ventricle. T1-weighted imaging with a fast gradient echo sequence was used to track the first pass on an injected contrast agent through the right and left ventricles. The first perfusion scan was performed during rest, and the second was performed approximately 15 minutes later during maximal vasodilation induced by 0.14 mg/kg/min of intravenous adenosine injected for >3 minutes before the scan.
Myocardial blood flow (MBF) was estimated before and after adenosine infusion in 8 segments: anterior, anterolateral, posterolateral, posteroinferior, inferior, and posterior/mid and anterior septum. Region-of-interest signal intensity curves were generated with the MASS CMR image analysis software (Laboratory for Clinical and Experimental Image Processing, Leiden University, Leiden, The Netherlands). MBF was estimated from the initial amplitude of the myocardial impulse response by deconvolution analysis of the myocardial signal intensity curves. The global averages of MBF at rest and hyperemic MBF over the 8 myocardial segments and 2 to 3 slices were used for analysis. MPR was modeled as hyperemic MBF with adjustment for MBF at rest.
As part of the study protocol, all patients had serum samples drawn at enrollment and stored at −70°C. NT-proBNP levels were measured using a highly sensitive and specific Elecsys electrochemiluminescence immunoassay (Roche Diagnostics Corporation, Indianapolis, Indiana).
Demographic characteristics and established cardiovascular risk factors were examined across hyperemic MBF tertiles. Continuous variables are presented as a mean ± SD and categorical variables as percentages. Logarithmic transformation was applied to variables with skewed distributions. To explore the shape of the association between NT-proBNP and MPR (hyperemic MBF with adjustment for MBF at rest), we modeled MPR as a restricted cubic spline in a multivariable linear model adjusted for age, race, and gender. Linear regression was used to assess the association of MPR and NT-proBNP with adjustment for covariates listed subsequently. The regression analyses was carried out in stages as follows:
Model I is unadjusted. Model II is adjusted for age, gender, and race. Model III is adjusted for age, gender, race, body mass index, coronary artery calcium (CAC) score, education level, fasting glucose, systolic and diastolic blood pressures, heart rate, high-density lipoprotein cholesterol, low-density lipoprotein cholesterol, medications (antihypertensive, statins, and diabetic medications), physical activity, smoking status, and left ventricular mass.
Similarly, the association between regional MPR in each of the 8 myocardial segments and NT-proBNP was examined using linear regression with adjustment for covariates according to models I to III.
Statistical analyses were performed using SAS, v9.2 (SAS Inc., Cary, North Carolina). Statistical significance was inferred at 2-sided p value <0.05.
Results
Overall, 184 study participants had both serum NT-proBNP measurements and cardiac MR quantification of MPR. Table 1 lists the baseline characteristics of this cohort stratified by hyperemic MBF. Patients in the lowest tertile of hyperemic MBF were older and predominantly men. They also had higher BMI, CAC score, fasting glucose, systolic and diastolic blood pressures, and were more likely to be on antihypertensive, diabetic, or statin medications ( Table 1 ).
Variable | Hyperemic MBF (mL/min/g) | P-Value | ||
---|---|---|---|---|
0.98-2.64 (n=61) | 2.65-3.40 (n=62) | 3.41-5.63 (n=61) | ||
Age, years | 65 ± 9 | 59 ± 11 | 57 ± 10 | 0.0002 |
Women | 16 % | 45 % | 70 % | <0.0001 |
White | 56 % | 63 % | 54 % | 0.58 |
Hispanic | 44 % | 37 % | 46 % | 0.58 |
Body Mass Index (kg/m 2 ) | 30 ± 5 | 28 ± 4 | 29 ± 5 | 0.03 |
CAC Score >0 | 80 % | 50 % | 39 % | <0.0001 |
CAC Score* | 53 ± 383 | 7 ± 80 | 4 ± 19 | <0.0001 |
Current Smoker | 8 % | 16 % | 20 % | 0.06 |
Fasting Glucose (mg/dL) | 101 ± 24 | 91 ± 25 | 92 ± 24 | 0.05 |
Heart Rate (beats/min) | 63 ± 10 | 60 ± 10 | 64 ± 8 | 0.05 |
High School Graduate | 66 % | 60 % | 59 % | 0.81 |
HDL Cholesterol (mg/dL) | 47 ± 15 | 50 ± 14 | 49 ± 13 | 0.37 |
LDL Cholesterol (mg/dL) | 122 ± 26 | 116 ± 32 | 111 ± 24 | 0.12 |
Systolic Blood Pressure (mmHg) | 125 ± 16 | 118 ± 19 | 120 ± 21 | 0.08 |
Diastolic Blood Pressure (mmHg) | 74 ± 9 | 69 ± 10 | 69 ± 10 | 0.01 |
Antihypertensive Medication | 33 % | 13 % | 18 % | 0.02 |
Diabetes Medication | 15 % | 2 % | 8 % | 0.03 |
Statins | 21 % | 5 % | 11 % | 0.02 |
Physical Activity (MET-min/week) | 9290 ± 6440 | 6641 ± 4791 | 7081 ± 5967 | 0.03 |
Left Ventricular Mass (g) | 183 ± 41 | 158 ± 42 | 145 ± 35 | <0.0001 |
Left Ventricular Ejection Fraction | 64 ± 8 | 66 ± 4 | 69 ± 6 | 0.003 |
Diabetes | 18 % | 6 % | 10 % | 0.12 |
Calcium Channel Blocker | 10 % | 0 % | 7 % | 0.05 |
CAC >300 | 33 % | 10 % | 8 % | 0.0002 |
Resting Mean MBF (mL/min/g) | 0.9 ± 0.2 | 1.0 ± 0.2 | 1.1 0.2 | <0.0001 |
Hyperemic Mean MBF (mL/min/g) | 2.1 ± 0.4 | 3.0 ± 0.2 | 4.0 ± 0.5 | <0.0001 |
Myocardial Perfusion Reserve | 2.4 ± 0.7 | 3.2 ± 0.6 | 3.6 ± 0.7 | <0.0001 |
The mean and SD of hyperemic MBF was 3.04 ± 0.829 ml/min/g. The median NT-proBNP was 51.6 pg/μL (IQR 26.6 to 121.2, range 4.9 to 1,085). The association of MPR, modeled as a restricted cubic spline, with log(NT-proBNP) is presented in Figure 1 , which illustrates the predicted values and 95% confidence intervals for a 59-year-old white woman. MPR was inversely associated with log(NT-proBNP), and the association was roughly linear. Results of the multivariable linear regression analysis are summarized in Table 2 . Table 3 displays results of the multivariable linear regression analysis examining the relation between regional MPR and NT-proBNP. NT-proBNP level was significantly associated with reduced regional MPR in the inferoseptal segment (model I) and the inferior and inferoseptal segments (models II and III).
Model | n | NT-proBNP | ||
---|---|---|---|---|
β (95% CI) ∗ | % Difference (95% CI) † | p-value | ||
I | 184 | 0.18 (-0.01, 0.36) | 19 (-1, 44) | 0.06 |
II | 184 | 0.21 (0.03, 0.39) | 23 (3, 48) | 0.02 |
III | 184 | 0.19 (0.01, 0.37) | 21 (1, 45) | 0.04 |
∗ Increment in log(NT-proBNP) in pg/mL per 1-standard deviation (SD) decrement in MPR (1-SD = 0.829 mL/min/g).
n | Model 1 | Model 2 | Model 3 | |||||||
---|---|---|---|---|---|---|---|---|---|---|
β (95% CI) ∗ | % Difference (95% CI) † | P-value | β (95% CI) ∗ | % Difference (95% CI) † | P-value | β (95% CI) ∗ | % Difference (95% CI) † | P-value | ||
Anterior Wall | 180 | 0.10 (-0.09, 0.29) | 10 (-9, 33) | 0.30 | 0.15 (-0.02, 0.32) | 16 (-2, 38) | 0.09 | 0.13 (-0.04, 0.31) | 14 (-4, 36) | 0.13 |
Anterior-Lateral Wall | 184 | 0.11 (-0.07, 0.29) | 11 (-7, 33) | 0.24 | 0.13 (-0.04, 0.30) | 14 (-4, 34) | 0.13 | 0.10 (-0.07, 0.28) | 11 (-7, 32) | 0.24 |
Lateral-Posterior Wall | 182 | 0.12 (-0.08, 0.31) | 12 (-7, 36) | 0.23 | 0.17 (-0.004,0.34) | 18 (0, 40) | 0.06 | 0.14 (-0.04, 0.31) | 15 (-4, 37) | 0.12 |
Posterior Wall | 183 | 0.06 (-0.13, 0.24) | 6 (-12, 28) | 0.55 | 0.12 (-0.06, 0.30) | 13 (-6, 36) | 0.20 | 0.10 (-0.08, 0.29) | 11 (-8, 33) | 0.26 |
Inferior Wall | 181 | 0.14 (-0.05, 0.33) | 15 (-4, 40) | 0.13 | 0.22 (0.04, 0.40) | 24 (4, 49) | 0.02 | 0.21 (0.02, 0.39) | 23 (2, 47) | 0.03 |
Inferior-Septal Wall | 178 | 0.27 (0.08, 0.45) | 31 (9, 57) | 0.004 | 0.27 (0.11, 0.43) | 31 (12, 54) | 0.001 | 0.24 (0.08, 0.41) | 28 (9, 50) | 0.003 |
Mid-Septal Wall | 170 | 0.19 (0.004, 0.37) | 20 (0, 45) | 0.05 | 0.21 (0.04, 0.38) | 23 (4, 46) | 0.02 | 0.21 (0.05, 0.38) | 24 (5, 46) | 0.01 |
Anterior-Septal Wall | 169 | 0.15 (-0.04, 0.34) | 17 (-3,41) | 0.11 | 0.13 (-0.04, 0.30) | 14 (-4, 35) | 0.13 | 0.11 (-0.06, 0.28) | 12 (-6, 32) | 0.21 |
Global | 184 | 0.18 (-0.01, 0.36) | 19 (-1, 44) | 0.06 | 0.21 (0.03, 0.39) | 23 (3, 48) | 0.02 | 0.19 (0.01, 0.37) | 21 (1, 45) | 0.04 |
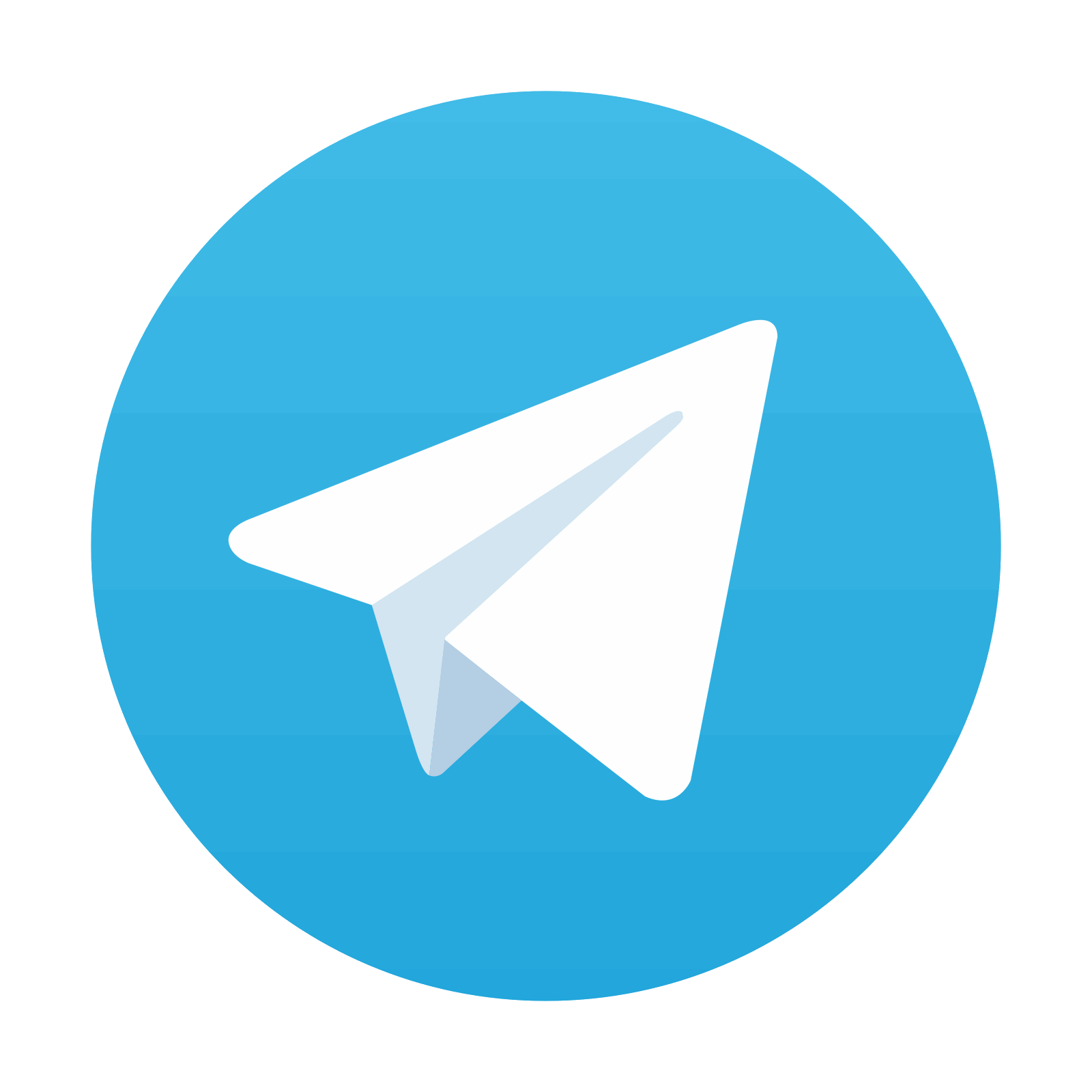
Stay updated, free articles. Join our Telegram channel

Full access? Get Clinical Tree
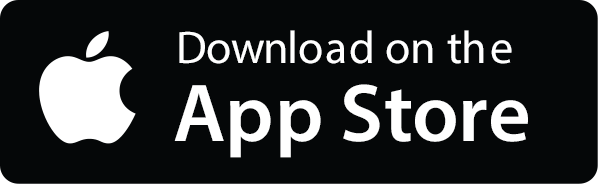
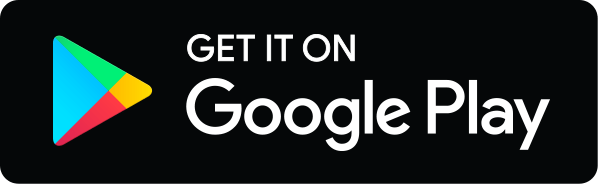
