Patients with type 2 diabetes mellitus are at increased risk for cardiovascular disease (CVD) and mortality. Beyond traditional CVD risk factors, novel measures reflecting additional aspects of disease pathophysiology, such as biventricular volume (BiVV), may be useful for risk stratification. The aim of this study was to examine the relationship between BiVV and risk for mortality in European Americans with type 2 diabetes mellitus from the Diabetes Heart Study (DHS). BiVV was calculated from 771 noncontrast computed tomographic scans performed to image coronary artery calcified plaque. Relationships between BiVV and traditional CVD risk factors were examined. Cox proportional-hazards regression was performed to determine risk for mortality (all-cause and CVD mortality) associated with increasing BiVV. Area under the curve analysis was used to assess BiVV utility in risk prediction models. During 8.4 ± 2.4 years of follow-up, 23% of the patients died. In unadjusted analyses, BiVV was significantly associated with increasing body mass index, height, coronary artery calcified plaque, history of hypertension, and previous myocardial infarction (p <0.0001 to 0.012). BiVV was significantly associated with all-cause (hazard ratio 2.45, 95% confidence interval 1.06 to 5.67, p = 0.036) and CVD (hazard ratio 4.36, 95% confidence interval 1.36 to 14.03, p = 0.014) mortality in models adjusted for other known CVD risk factors. Area under the curve increased from 0.76 to 0.78 (p = 0.04) and from 0.74 to 0.77 (p = 0.02) for all-cause and CVD mortality with the inclusion of BiVV. In conclusion, in the absence of echocardiography or other noninvasive imaging modalities to assess ventricular volumes, or when such methods are contraindicated, BiVV from computed tomography may be considered a tool for the stratification of high-risk patients, such as those with type 2 diabetes mellitus.
Coronary artery calcified plaque (CAC) scores, determined by computed tomographic scanning, are an independent predictor of all-cause mortality and cardiovascular disease (CVD) mortality in the Diabetes Heart Study (DHS), a family-based study enriched for type 2 diabetes mellitus (DM). In addition to CAC, other reported cardiovascular-related predictors of all-cause and CVD mortality include arterial stiffness, conduction wave abnormalities (as determined by electrocardiography), and left ventricular hypertrophy (LVH). More recently, biventricular volume (BiVV) determined from noncontrast computed tomographic scans (as used for the measurement of CAC) has been identified as a safe, noninvasive, and cost-effective surrogate of left ventricular mass, with correlation coefficients >0.8 compared with left ventricular mass derived from magnetic resonance imaging. Several methods have been described to calculate ventricular volumes, however, relationships between BiVV and other traditional markers of CVD risk remain unclear. In addition, whether BiVV is similarly predictive of mortality has not been investigated. The aim of the present investigation was to assess the relationships between BiVV and traditional cardiac risk factors and to examine the ability of BiVV to predict risk for all-cause and CVD mortality in patients with DM from the DHS.
Methods
This investigation included 771 self-described European American patients (from 352 families) with overt type 2 DM from the DHS. Briefly, the DHS includes siblings concordant for type 2 DM without advanced renal insufficiency. Type 2 DM was clinically defined as DM developing after the age of 35 years and treated with insulin and/or oral agents, in the absence of historical evidence of ketoacidosis. Ascertainment and recruitment have been described in detail previously.
Study protocols were approved by the institutional review board at the Wake Forest School of Medicine, and all participants provided written informed consent before participation. Participant examinations were conducted at the General Clinical Research Center of the Wake Forest Baptist Medical Center and included interviews for medical history and health behaviors, recording of anthropometric measures and rest blood pressure, fasting blood sampling for laboratory analyses, and spot urine collection. Standard laboratory analyses included fasting glucose, glycosylated hemoglobin, total cholesterol, low-density lipoprotein cholesterol, high-density lipoprotein cholesterol, triglycerides, and C-reactive protein concentrations. Patients reported histories of CVD on the basis of previous events (angina, myocardial infarction, stroke) and/or intervention (coronary angiography, coronary artery bypass grafting, carotid endarterectomy). Patients were classified as hypertensive if prescribed antihypertensive medications or if they had blood pressure measurements >140 mm Hg (systolic) or 90 mm Hg (diastolic) and as dyslipidemic on the basis of the criteria established in the third report of the National Cholesterol Education Program Expert Panel on Detection, Evaluation, and Treatment of High Blood Cholesterol in Adults.
Participant examinations included measurement of carotid artery intima-media thickness using high-resolution B-mode ultrasonography with a 7.5-MHz transducer and a Biosound Esaote (AU5) ultrasound machine (Biosound Esaote, Inc., Indianapolis, Indiana), as previously described. In addition, rest 12-lead electrocardiography was performed using a Marquette MAC 500 instrument (Marquette Electronics, Milwaukee, Wisconsin), as described previously. All data were transmitted electronically to a central reading center for processing (EPICARE Inc., Winston-Salem, North Carolina). Electrocardiographic (ECG) LVH was determined according to the Minnesota code criteria ( http://www.sph.umn.edu/epi/ecg/ ).
The imaging protocol has been reported previously. In brief, images to calculate BiVV were captured using fast-gated helical computed tomography. All cardiac computed tomographic examinations were performed on a single-slice helical computed tomograph or a 4-channel multidetector computed tomograph, both with cardiac gating and capable of 500-ms temporal resolution (HiSpeed LX and LightSpeed QXi with the SmartScore Cardiac scan package; GE Medical Systems, Waukesha, Wisconsin). After a scout image of the chest was obtained, the heart was scanned during suspended respiration at end-inspiration. Scan parameters for the single-slice system were 3-mm slice thickness, 26-cm display field of view, retrospective cardiac gating, tube voltage of 120 kV, tube current of 240 mA, and computed tomographic scan pitch adjusted to heart rate; parameters for multidetector computed tomography were 2.5-mm slice thickness in 4-slice mode, 26-cm display field of view, prospective cardiac gating at 50% of the RR interval, tube voltage of 120 kV, and tube current of 240 mA. BiVV was calculated using the modified Simpson’s formula : (maximum short-axis area + midventricular area) × (ventricular length/3) + [area at apex × (ventricular length) 2 × π]/108.
BiVV has been highly correlated (r = 0.804) with left ventricular mass obtained from magnetic resonance imaging. Determinations of CAC, carotid artery calcified plaque, and infrarenal abdominal aortic calcified plaque were also made from computed tomographic images, as previously described, and are reported as Agatston scores.
For all participants, vital status was determined from the Social Security Death Index maintained by the United States Social Security Administration. For participants confirmed as deceased, length of follow-up was determined from the date of the initial study visit to the date of death. For deceased participants, death certificates were obtained from relevant county vital records offices to confirm cause of death. For all other participants, length of follow-up was determined from the date of the initial study visit to the end of 2011. Cause of death was categorized on the basis of information contained in death certificates as CVD mortality (myocardial infarction, congestive heart failure, cardiac arrhythmia, sudden cardiac death, peripheral vascular disease, and stroke) or cancer, infection, end-stage renal disease, accidental, or other (including obstructive pulmonary disease, pulmonary fibrosis, liver failure and Alzheimer’s dementia).
Summary statistics were calculated, including means and SDs for continuous variables and counts and percentages for categorical variables. Because of the inclusion of related patients in this study, marginal models with the incorporation of generalized estimating equations were used to describe associations between BiVV and cardiovascular risk factors. First, analysis of the simple univariate associations between BiVV and each individual risk factor or demographic trait were performed, in which BiVV was treated as the outcome variable. Second, each of these associations was adjusted for age, gender, and body mass index (BMI) (for the analysis with height, the BMI adjustment was not performed). For the analysis with ECG LVH, patients with electrocardiographic readings failing to meet the minimum accepted quality standard were excluded (n = 13). Continuous variables (including BiVV and CAC) were log-transformed to better approximate the normality assumption as appropriate. The statistical inference (i.e., p value) is reported on the basis of the log-transformed BiVV analysis, but to allow easier interpretation, β coefficients for untransformed BiVV are reported. This approach is reasonable when the p values from transformed and untransformed analyses are similar.
To evaluate the association between BiVV and mortality, BiVV was considered as a continuous (log-transformed) and a categorical variable derived from quartile ranges (quartiles 1 to 4, on the basis of increasing BiVV). The curves of the cumulative incidence of all-cause and CVD mortality for each quartile of BiVV were plotted for exploratory analysis. Cox proportional-hazards models were used to assess the ability of BiVV to predict all-cause and CVD mortality. To account for the correlated family structure, a robust sandwich covariance estimate was calculated. The robust standard error for the parameter estimates were used for statistical inference. Follow-up duration started from the beginning of the study to the last updated assessment of vital status. When the end point is all-cause mortality, censoring may occur if one is alive. When the end point is CVD mortality, censoring may occur if one is alive or the death is due to other causes. Models were adjusted for (1) age and gender (model 1), (2) age, gender, height, and known CVD risk factors including current smoking, hypertension, dyslipidemia, and history of myocardial infarction (model 2), and (3) age, gender, height, known CVD risk factors, ECG LVH, and CAC (model 3). The test for trend across increasing BiVV quartiles was also performed. SAS version 9.2 (SAS Institute Inc., Cary, North Carolina) was used for the analysis.
Receiver-operating characteristic curves were computed for the basic (model 1) and fully adjusted (model 3) model and progressive addition of continuous measures of CAC and BiVV. The areas under the curves were used to assess the ability of BiVV to predict all-cause or CVD mortality after adjusting for other confounding variables and the known predictor CAC. The difference in areas under the curves between 2 models was tested using Delong’s method. Stata version 8.2 (StataCorp LP, College Station, Texas) was used for the receiver-operating characteristic curve analysis. Statistical significance was accepted at p <0.05.
Results
The demographic and clinical characteristics of the participants are listed in Table 1 . A predominance of known CVD risk factors, including high body mass, hypertension, and abnormal lipid concentrations, was evident. Vascular calcified plaque scores for CAC, carotid artery calcified plaque, and abdominal aortic calcified plaque reflect a substantial burden of subclinical CVD in these DM-affected patients. On the basis of available data, 4.3% of the sample had ECG LVH, defined using Minnesota code criteria. The cohort was followed for 8.4 ± 2.4 years, with 180 participants found to have died (23.3% of the sample) during this period. Among those patients with documented causes of death (n = 152), 82 (53.9%) had documented CVD-related causes of death.
Variable | All (n = 771) | Living (n = 591) | Deceased (n = 180) |
---|---|---|---|
Demographic information | |||
Age (yrs) | 61.9 ± 8.9 (36.3–86.0) | 60.5 ± 8.4 (36.3–82.8) | 66.7 ± 9.0 (41.8–86.0) |
Men | 49.2% | 47.2% | 55.6% |
Smoking (current or former) | 44.0% | 43.5% | 45.6% |
Metabolic syndrome | 89.0% | 89.0% | 88.9% |
DM duration (yrs) | 10.6 ± 7.1 (1.0–41.1) | 9.6 ± 6.3 (1.0–30.8) | 13.6 ± 8.5 (1.0–41.1) |
Self-reported CVD history | 44.0% | 38.9% | 60.6% |
Self-reported previous myocardial infarction | 21.4% | 16.4% | 37.8% |
Deceased, CVD causes | 9.3% | — | 40.0% |
Body composition | |||
Height (cm) | 168.8 ± 9.7 (122.8–195.8) | 168.8 ± 9.8 (122.8–195.8) | 168.8 ± 9.5 (147.4–189.0) |
Weight (kg) | 92.3 ± 20.1 (40.8–209.2) | 92.8 ± 20.0 (44.1–209.2) | 90.5 ± 20.6 (40.8−152.1) |
BMI (kg/m 2 ) | 32.4 ± 6.6 (17.1–58.0) | 32.6 ± 6.5 (17.1–58.0) | 31.7 ± 6.8 (17.5–56.9) |
Blood pressure | |||
Systolic (mm Hg) | 140 ± 19 (98–260) | 140 ± 17 (98–214) | 141 ± 23 (99–260) |
Diastolic (mm Hg) | 72 ± 10 (37–102) | 73 ± 10 (37–102) | 69 ± 10 (45–101) |
Pulse pressure (mm Hg) | 68 ± 17 (28–159) | 67 ± 16 (28–124) | 71 ± 19 (32–159) |
Hypertension | 88.7% | 87.1% | 93.9% |
Antihypertensive medication use | 78.7% | 76.1% | 87.2% |
Blood biochemistry | |||
Fasting glucose (mg/dl) | 150 ± 57 (16–463) | 148 ± 54 (16–463) | 156 ± 68 (46–436) |
Glycosylated hemoglobin (%) | 7.5 ± 1.6 (4.3–16.6) | 7.4 ± 1.5 (4.6–15.4) | 7.8 ± 1.8 (4.3–16.6) |
Cholesterol (mg/dl) | 186 ± 43 (74–427) | 186 ± 43 (74–427) | 187 ± 43 (105–386) |
Low-density lipoprotein (mg/dl) | 103 ± 32 (14–236) | 103 ± 32 (14–236) | 104 ± 32 (33–228) |
High-density lipoprotein (mg/dl) | 43 ± 12 (8–98) | 43 ± 12 (18–98) | 41 ± 12 (8–86) |
Triglycerides (mg/dl) | 208 ± 133 (30–1,068) | 204 ± 127 (30–1,068) | 221 ± 152 (38–1,065) |
Dyslipidemia | 94.3% | 93.6% | 96.7% |
C-reactive protein (mg/L) | 6.3 ± 9.7 (0.05–96) | 5.7 ± 8.3 (0.05–96) | 8.4 ± 12.8 (0.08–90) |
CVD severity | |||
CAC >0 | 96.0 % | 94.6% | 98.9% |
Mean CAC | 2,096 ± 3,557 (0–50,415; 604) | 1,640 ± 3,412 (0–50,415; 374) | 3,586 ± 3,622 (0–21,725; 2,506) |
CarCP >0 | 79.3% | 74.6% | 93.9% |
Mean CarCP | 390 ± 745 (0–6,122; 97) | 296 ± 656 (0–6,122; 51) | 692 ± 916 (0–5,605; 299) |
AACP >0 | 96.7% | 96.2% | 98.3% |
Mean AACP | 13,644 ± 17,113 (0–94,156; 6,766) | 10,998 ± 15,046 (0–75,567; 4,525) | 23,482 ± 20,497 (0–94,156; 18,732) |
Carotid intima-media thickness (mm) | 0.687 ± 0.137 (0.451–1.569) | 0.671 ± 0.132 (0.451–1.569) | 0.739 ± 0.140 (0.498–1.316) |
Ventricular volume | |||
BiVV (ml) | 384.5 ± 112.1 (156.6–884.5) | 374.6 ± 105.5 (156.6–884.5) | 416.8 ± 128.7 (188.1–798.3) |
BiVV quartile ranges | |||
Quartile 1 | 156.6–300.9 | 156.6–297.5 | 188.1–332.2 |
Quartile 2 | 300.9–375.6 | 297.5–364.1 | 332.2–395.9 |
Quartile 3 | 375.6–448.8 | 364.1–438.6 | 395.9–495.3 |
Quartile 4 | 448.8–884.5 | 438.6–884.5 | 495.3–798.3 |
In unadjusted analyses, BiVV was significantly associated with increasing BMI, height, CAC, and histories of hypertension and myocardial infarction ( Table 2 ). After adjustment for age, gender, and BMI, BiVV remained associated (p <0.01) with hypertension, previous myocardial infarction, CAC, and LVH ( Table 2 ). BiVV was not significantly associated with dyslipidemia or current smoking status.
Variable | Unadjusted | Adjusted for Age, Gender, and BMI | ||
---|---|---|---|---|
β | p Value | β | p Value | |
Age | −1.13 | 0.01 | — | — |
Gender (male) | 119.33 | <0.0001 | — | — |
BMI | 2.77 | <0.0001 | — | — |
Height | 5.96 | <0.0001 | 2.82 | <0.0001 |
Hypertension | 32.97 | 0.01 | 34.98 | 0.0003 |
Current smoking | 5.64 | 0.61 | −7.94 | 0.37 |
Dyslipidemia | 5.92 | 0.41 | −2.05 | 0.89 |
Previous myocardial infarction | 54.05 | <0.0001 | 28.05 | 0.0024 |
CAC | 13.66 | <0.0001 | 7.12 | 0.0010 |
ECG LVH | 47.31 | 0.07 | 56.41 | 0.0074 |
The cumulative incidence of all-cause and CVD mortality increased across increasing BiVV quartiles ( Figure 1 ). A linear relationship was observed whereby risk for all-cause and CVD mortality increased with greater heart size, although only the comparison between the highest quartile of BiVV values and the lowest quartile was significant ( Table 3 ). A formal test for trend of BiVV as an ordinal measure (using quartiles as cut points) supported an overall trend for increasing BiVV values (on the basis of quartiles) to be associated with increased risk for all-cause (hazard ratio 1.28, 95% confidence interval 1.12 to 1.47, p = 0.0005) and CVD (hazard ratio 1.31, 95% confidence interval 1.07 to 1.61, p = 0.011) mortality in a univariate analysis.
Mortality | Quartile | No. of Events | Events/100 Person-Years | Unadjusted | Partially Adjusted ∗ | Fully Adjusted † | |||
---|---|---|---|---|---|---|---|---|---|
HR (95% CI) | p Value | HR (95% CI) | p Value | HR (95% CI) | p Value | ||||
All-cause | 1 (reference) | 31 | 2.1 | 1.00 | — | 1.00 | — | 1.00 | — |
2 | 36 | 2.2 | 1.05 (0.63–1.72) | 0.86 | 1.05 (0.64 1.73) | 0.85 | 0.93 (0.56–1.56) | 0.80 | |
3 | 46 | 2.8 | 1.34 (0.84–2.11) | 0.22 | 1.47 (0.84–2.57) | 0.18 | 1.22 (0.64–2.30) | 0.55 | |
4 | 67 | 4.1 | 1.98 (1.29–3.05) | 0.002 | 2.31 (1.35–3.97) | 0.002 | 1.82 (1.02–3.25) | 0.04 | |
CVD | 1 (reference) | 15 | 1.0 | 1.00 | — | 1.00 | — | 1.00 | — |
2 | 15 | 0.9 | 0.90 (0.44–1.83) | 0.77 | 0.85 (0.42–1.71) | 0.64 | 0.69 (0.32–1.47) | 0.33 | |
3 | 19 | 1.1 | 1.14 (0.59–2.19) | 0.70 | 1.08 (0.50–2.35) | 0.84 | 0.90 (0.36–2.25) | 0.81 | |
4 | 33 | 2.0 | 2.01 (1.11–3.64) | 0.02 | 2.19 (1.10–4.37) | 0.03 | 1.78 (0.83–3.83) | 0.14 |
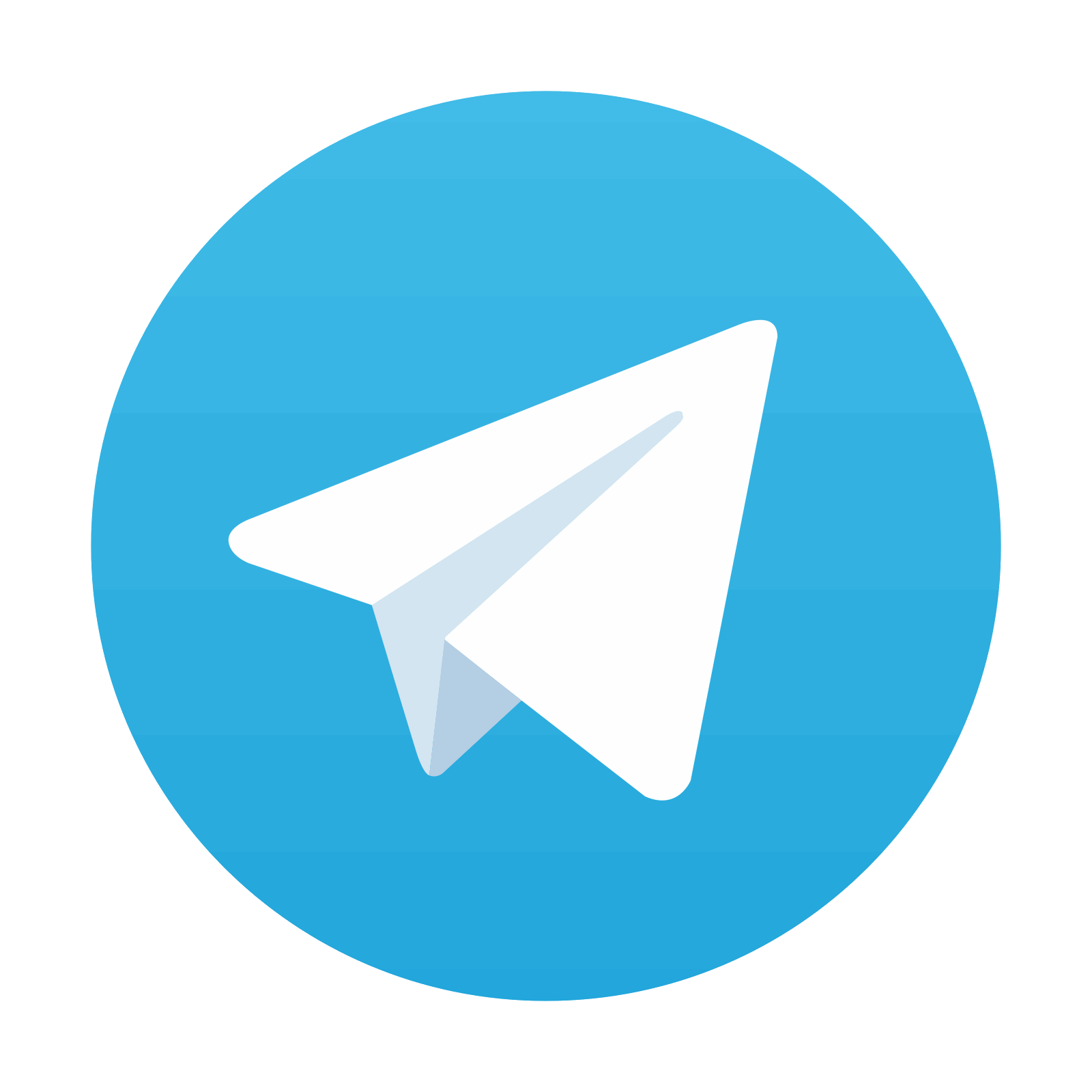
Stay updated, free articles. Join our Telegram channel

Full access? Get Clinical Tree
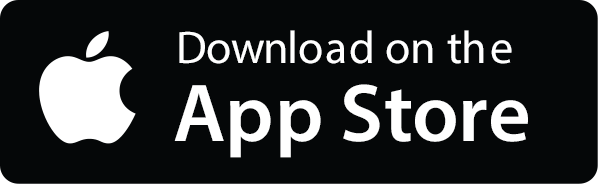
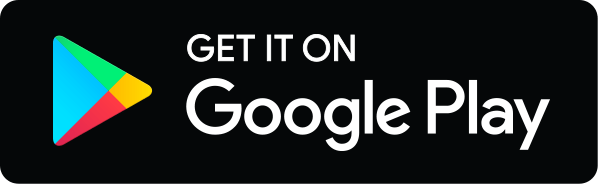
