Background
The aim of this study was to determine whether global strains derived from three-dimensional (3D) speckle-tracking echocardiography (STE) are as accurate as left ventricular (LV) ejection fraction (LVEF) obtained by two-dimensional (2D) and 3D echocardiography in the quantification of LV function.
Methods
Two-dimensional and 3D echocardiography and 2D and 3D STE were performed in 88 patients (LVEF range, 17%–79%). Two-dimensional and 3D global longitudinal strain (GLS), global circumferential strain (GCS), global radial strain, and global area strain were quantified and correlated with LV function determined by 2D and 3D echocardiographic LVEF. Reproducibility, feasibility, and duration of study to perform 3D STE were assessed by independent, blinded observers.
Results
A total of 78 patients (89%) underwent 3D STE. All 3D speckle-tracking echocardiographic parameters had strong correlations with assessment of LV function determined by 2D and 3D echocardiographic LVEF. Three-dimensional GCS was the best marker of LV function ( r = −0.89, P < .0001). Subgroup analysis demonstrated that 3D speckle-tracking echocardiographic parameters were particularly useful in identifying LV dysfunction (LVEF < 50%). Receiver operating characteristic curve analysis demonstrated areas under the curve of 0.97 for 3D GCS, 0.96 for 3D global radial strain, 0.95 for 3D global area strain, and 0.87 for 3D GLS. An optimal 3D GCS cutoff value of magnitude < −12% predicted LV dysfunction (LVEF obtained by 2D echocardiography < 50%) with 92% sensitivity and 90% specificity. There was good correlation between 2D GLS and 3D GLS ( r = 0.85, P < .001; mean difference, −1.7 ± 6.5%). Good intraobserver, interobserver, and test-retest agreements were seen with 3D STE. Time for image acquisition to postprocessing analysis was significantly reduced with 3D STE (3.7 ± 1.0 minutes) compared with 2D STE (4.6 ± 1.5 min) ( P < .05).
Conclusions
Global strain by 3D STE is a promising novel alternative to quantitatively assess LV function. Three-dimensional STE is reproducible, feasible, and time efficient.
Left ventricular (LV) systolic function is an important prognostic predictor of cardiac mortality and morbidity. LV ejection fraction (LVEF) remains the most commonly used conventional parameter to quantify global LV function and has been incorporated into guidelines for clinical decision making. However, despite its widespread clinical use, assessment of LVEF is limited by problems with reproducibility, dependence on image quality, subjective interpretation, and LV geometric assumptions.
The recent development of two-dimensional (2D) speckle-tracking echocardiography (STE) has allowed advances in the quantification of LV function beyond traditional assessment of LVEF. Global strain measurements by 2D STE have excellent correlations with LVEF validated by both three-dimensional (3D) echocardiography and cardiac magnetic resonance imaging (MRI). Furthermore, global strain by 2D STE may be superior to LVEF by echocardiography as a powerful prognostic predictor of cardiac events in patients with heart failure.
However, myocardial mechanics of LV function, generated by real-time cardiac motion, are complex and 3D, and they involve multidirectional axes of motion. The limitation of 2D STE is that tracking of speckles in a 2D plane may be subject to out-of-plane motion, which may contribute to noise and reduced accuracy. The newly developed modality 3D STE may overcome this limitation because it allows the acquisition of a full 3D volumetric data set with simultaneous evaluation of multidirectional components of strain and LVEF in a single session with a single postprocessing algorithm. Additionally, LVEF obtained by 3D STE will overcome the limitation of geometric assumptions with LVEF obtained by 2D echocardiography, resulting in improved accuracy and reproducibility of LV functional quantification.
The objectives of our study were (1) to validate the novel modality of 3D STE as an alternative quantifier of LV function by comparing the accuracy of four different components of 3D global strain (global longitudinal strain [GLS], global circumferential strain [GCS], global radial strain [GRS], and global area strain [GAS]) with that of LVEF obtained by 2D and 3D echocardiography and (2) to validate the reproducibility and feasibility of 3D STE in a wide range of LVEFs.
Methods
Study Population
This was a single-center prospective study. We enrolled 88 patients (mean age, 72 ± 15 years; 3D echocardiographic LVEF range, 17%–79%) referred for echocardiographic assessment at our institution. All patients underwent conventional 2D and 3D echocardiography on the same day. Patients with (1) unstable clinical conditions, (2) suboptimal image quality for analysis (defined as more than three poorly visualized segments), or (3) arrhythmias such as atrial fibrillation, atrial flutter, or frequent ventricular ectopy were excluded.
Echocardiography
Experienced sonographers using a Vivid E9 ultrasound scanner (GE Vingmed Ultrasound AS, Horten, Norway) with a transthoracic matrix phased-array transducer (4V-D; GE Vingmed Ultrasound AS) scanned patients in the left lateral recumbent position.
Standard 2D echocardiographic images in the parasternal long-axis and short-axis views, and LV apical four-chamber and two-chamber and long-axis views were obtained. Grayscale images were obtained with frame rates of 50 to 70 frames/second.
Real-time 3D echocardiographic images in the LV apical four-chamber, two-chamber, and long-axis views were acquired using the matrix-array transducer (4V-D). Image acquisition occurred during four cardiac cycles to ensure optimal temporal and spatial resolution at a frame rate of 25 to 30 frames/second, which can be manually adjusted from the machine settings to ensure correct frame rate. Multibeat image acquisition was performed while the patient’s breath was held to eliminate breathing-related motion artifacts. Careful attention was taken to adjust the sector width and depth to include the entire LV myocardium, including the epicardial surface, within the pyramidal scan volume.
Acquired 2D and 3D data were digitally transferred to a separate workstation for offline analysis of LV volumes, LVEF, and strain using EchoPAC BT011 software (GE Vingmed Ultrasound AS). End-systolic and end-diastolic LV volumes were obtained from the apical four-chamber and two-chamber views using the method of disks, and LVEF obtained by 2D echocardiography was calculated using Simpson’s biplane method.
2D STE Strain Analysis
GLS obtained by 2D STE was measured using a software-specific semiautomated function image tool. Endocardial borders were manually defined, and myocardial motion was tracked by a semiautomated algorithm in LV apical four-chamber, two-chamber, and long-axis views. GCS and GRS were obtained from the parasternal short-axis view at the mid-LV level. Endocardial borders were adjusted as required. The observer was able to override an unsatisfactory tracking result if deemed visually satisfactory, and vice versa. Automated frame-by-frame tracking of speckles throughout all 17 LV myocardial segments to measure GLS and six segments at the mid-LV level to measure GRS and GCS was obtained by 2D STE.
3D STE Strain Analysis
LV volumes, LVEF, and GLS obtained by 3D STE were measured using the software-specific semiautomated function image tool. The operator first ensured adequate alignment of apical views to minimize foreshortening. Second, endocardial borders for end-diastole and end-systole were defined by marking the midpoint of the mitral annular plane and the LV apex. Automated definition of the LV endocardial border was performed, with subsequent manual adjustment by the operator to ensure good approximation with the LV endocardial surface. This analysis defined LV end-systolic and end-diastolic volumes and LVEF obtained by 3D STE. Thereafter, definition of the myocardial wall was performed by confirming correct alignment of endocardial and epicardial contours, allowing automated 3D speckle-tracking echocardiographic strain analysis of all 17 LV segments. Peak GLS, GCS, GRS, and GAS values obtained by 3D STE were measured. The user interface allows manual rejection of segments with suboptimal tracking. Global strain values could not be calculated if more than three segments were suboptimal.
Image Acquisition and Analysis Time
Image acquisition and analysis time was measured in all patients. Acquisition and analysis time was compared between 2D GLS and 3D STE. Analysis time for 3D speckle-tracking echocardiographic parameters included calculation of 3D LV volumes, 3D LVEF, and all four 3D speckle-tracking echocardiographic parameters from a single vendor-specific algorithm as described earlier.
Reproducibility Analysis
Twenty cases were randomly selected to assess interobserver and intraobserver agreement and test-retest reliability during strain analysis. Interobserver agreement was assessed between two independent, blinded observers. Intraobserver assessment was performed ≥1 week apart, with blinding to prior results. For assessment of test-retest reliability, a complete echocardiographic study was performed <1 hour after the first study, without alteration of hemodynamics or therapy. Reproducibility results were expressed as coefficients of variation and standard errors of the mean between two measurements. Intraclass correlation coefficients with 95% confidence intervals also were calculated.
Statistical Analysis
Continuous variables are expressed as mean ± SD or as percentages. Correlation between strain measurements and LVEF were assessed using linear regression analysis with Pearson’s correlation coefficient ( r ). Times required for image acquisition and postprocessing analysis between 2D STE and 3D STE were compared using paired t tests. Receiver operating characteristic curves were computed to determine optimal strain cutoff values to detect LV dysfunction (LVEF obtained by 2D echocardiography < 50%). P values < .05 were considered statistically significant. Data were analyzed using standard statistical software (SPSS version 13.0; SPSS, Inc, Chicago, IL).
Results
Baseline Patient and Echocardiographic Characteristics
The final study population consisted of 78 patients after the exclusion of 10 patients because of unstable clinical condition, arrhythmias, or suboptimal image quality. The study population consisted of 41 patients with nonischemic cardiomyopathy, 24 with ischemic heart disease, and 13 without echocardiographic abnormalities, histories of cardiac disease, or other significant medical conditions. Baseline patient and echocardiographic characteristics are illustrated in Table 1 .
Variable | Value |
---|---|
Men | 67% |
Age (y) | 71 ± 15 |
Body surface area (m 2 ) | 1.87 ± 0.21 |
Heart rate (beats/min) | 70 ± 12 |
2D LVEF (%) | 48 ± 17 (10 to 80) |
3D LVEF (%) | 47 ± 16 (17 to 79) |
LV end-diastolic volume (mL), 3D STE | 140 ± −81 (42 to 508) |
LV end-systolic volume (mL), 3D STE | 83 ± 72 (9 to 416) |
2D GLS (%) | −13.5 ± 6.2 |
3D GAS (%) | −22.8 ± 9.0 |
3D GCS (%) | −13.8 ± 6.1 |
3D GLS (%) | −11.7 ± 5.1 |
3D GRS (%) | 34.6 ± 17.1 |
Correlations between 3D STE and LVEF
Strong correlations were demonstrated between all four 3D speckle-tracking echocardiographic parameters and LVEF assessed using both 3D and 2D echocardiography ( Table 2 ). The strongest correlate for LVEF obtained using both 2D and 3D echocardiography was with 3D GCS ( r = −0.89, P < .0001). The results for 3D GAS were r = −0.87 for 2D LVEF and r = −0.86 for 3D LVEF ( P < .0001) and for 3D GRS were r = −0.88 for 2D LVEF and r = −0.87 for 3D LVEF ( P < .0001). The weakest correlate of LVEF was 3D GLS ( r = −0.74 for 2D LVEF, r = −0.75 for 3D LVEF; P < .0001).
Variable | 2D LVEF | 3D LVEF |
---|---|---|
3D global strain | ||
GLS | −0.74 ∗ | −0.75 ∗ |
GCS | −0.89 ∗ | −0.89 ∗ |
GRS | 0.86 ∗ | 0.87 ∗ |
GAS | −0.87 ∗ | −0.88 ∗ |
2D global strain | ||
GLS | −0.86 ∗ | −0.86 ∗ |
GCS | −0.82 ∗ | −0.84 ∗ |
GRS | 0.67 ∗ | 0.64 ∗ |
Subgroup analysis dividing 2D LVEF into three groups (<30%, 30%–50%, and >50%) demonstrated better correlations at lower LVEFs across all 3D speckle-tracking echocardiographic parameters ( Table 3 ). Correlations improved significantly when 2D LVEF was <50%.
Variable | 2D LVEF < 30% ( n = 13) | 2D LVEF 30%–50% ( n = 24) | 2D LVEF >50% ( n = 41) | |||
---|---|---|---|---|---|---|
r | P | r | P | r | P | |
3D GLS | −0.36 | .23 | −0.68 | .0002 | −0.15 | .34 |
3D GCS | −0.70 | .008 | −0.61 | .002 | −0.55 | .0002 |
3D GRS | 0.73 | .005 | 0.73 | <.0001 | 0.42 | .007 |
3D GAS | −0.72 | .006 | −0.69 | .0002 | −0.34 | .029 |
Receiver operating characteristic curve analysis demonstrated strong predictive value for all four 3D speckle-tracking echocardiographic parameters in predicting LV dysfunction ( Table 4 , Figure 1 ), particularly 3D GCS, for which a cutoff strain value of magnitude < −12% predicted LV dysfunction (2D LVEF < 50%) with an area under the curve of 0.97, sensitivity of 92%, and specificity of 90%.
Variable | Cutoff (%) | Area under the curve | Sensitivity (%) | Specificity (%) |
---|---|---|---|---|
2D GLS | −12.0 | 0.97 | 84 | 95 |
3D GLS | −11.2 | 0.87 | 81 | 71 |
3D GCS | −12.0 | 0.97 | 92 | 90 |
3D GRS | 33.0 | 0.96 | 95 | 86 |
3D GAS | −23.0 | 0.95 | 95 | 88 |
Correlations between 2D STE and LVEF
The strongest 2D STE correlate for LVEF obtained using both 2D and 3D echocardiography was 2D GLS ( r = −0.86, P < .0001). The correlations of 2D GCS with 2D and 3D LVEF were −0.82 and −0.84, respectively ( P < .001). The weakest correlate of LVEF was 2D GRS ( r = 0.67 for 2D LVEF, r = 0.64 for 3D LVEF; P < .0001), which was inferior to 3D STE correlations ( Table 2 ).
Correlations between 3D STE and 2D STE
A strong correlation was demonstrated between 2D and 3D GLS ( r = 0.85, P < .0001), with a mean error of measurement of −1.7 ± 6.5% ( Figure 2 ).
Feasibility and Timing
Analysis was possible in 90.9% and 88.6% of the enrolled patients by 2D STE and 3D STE, respectively. The 10 patients excluded from 3D speckle-tracking echocardiographic analysis included six for poor image quality; two for severe LV dilatation, which was unable to be adequately included within the sector width to allow analysis; and two for atrial fibrillation. In the 2D STE group, two patients were excluded for atrial fibrillation and six because of poor image quality.
Image acquisition time was significantly faster for 3D STE compared with 2D STE, whereas data analysis time was mildly prolonged, as illustrated in Table 5 . Overall, combined duration for image acquisition and data processing remained significantly shorter with 3D STE. The total analysis time for 3D STE was 3.7 ± 1.0 minutes, which equals a 22% reduction in overall time consumption.
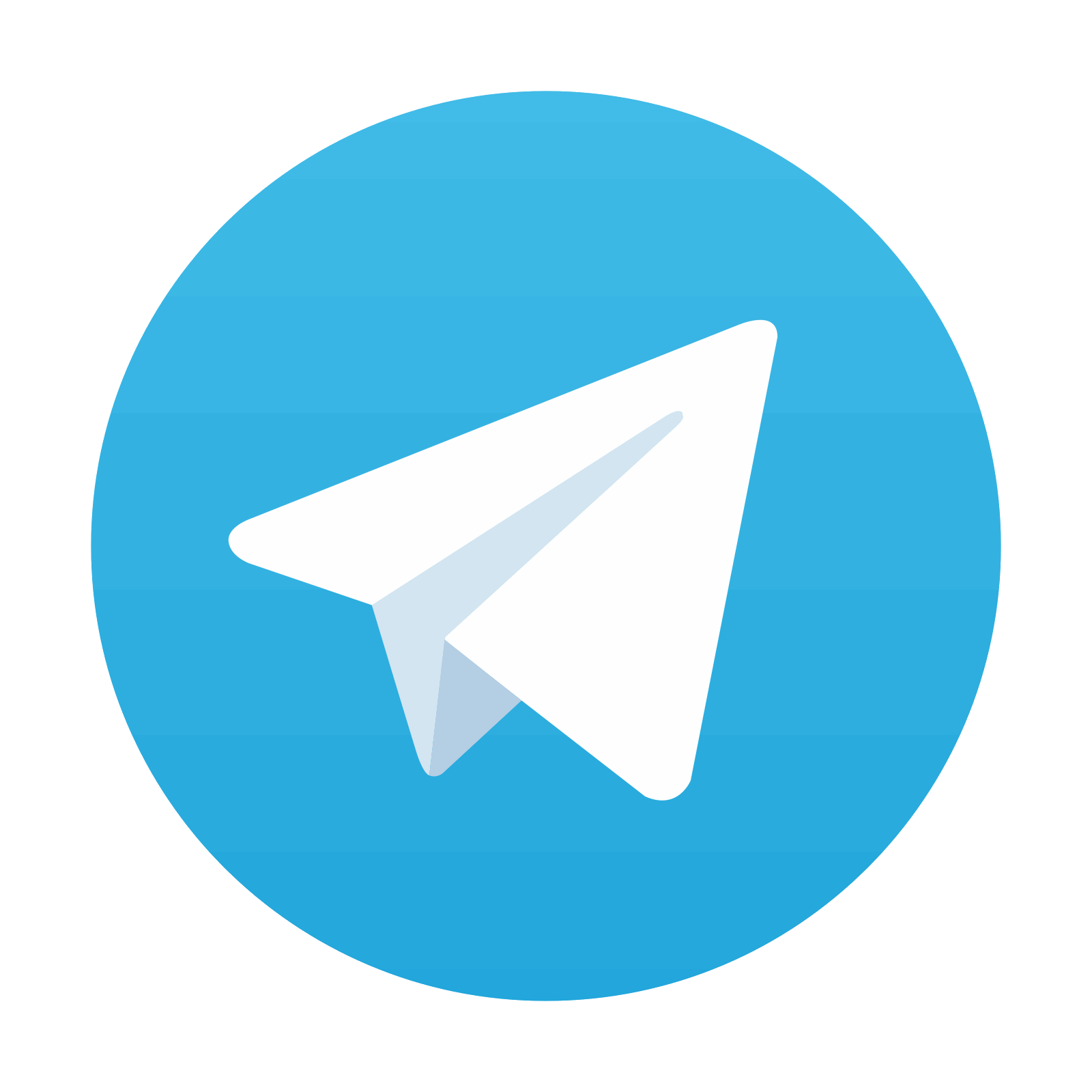
Stay updated, free articles. Join our Telegram channel

Full access? Get Clinical Tree
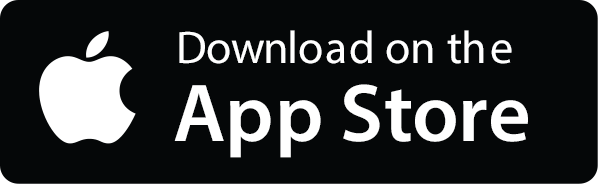
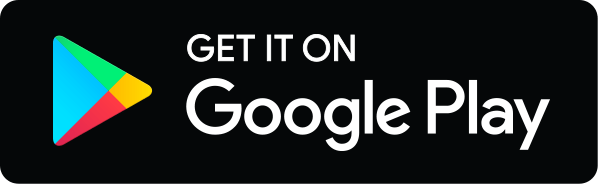
