Ultrastructure and Morphometry of the Human Lung
Matthias Ochs
Peter H. Burri
Joan Gil
Ewald R. Weibel
Organization of the Lung
The application of electron microscopy and quantitative methods in morphology (morphometry) has widened the general understanding of lung structure and set the course for a more functional approach to the study of pulmonary architecture. It cannot be the aim of this chapter, however, to cover all aspects of lung microanatomy; in this respect, the reader is referred to the specialized literature. The authors would rather present the morphologic and quantitative background needed to understand the functioning of the gas-exchange apparatus.
The lung is composed of three phases: air, tissue, and blood. The tissue forms a complete barrier between air and blood; it is a stable structural framework, whereas air and blood are continuously exchanged. In describing the ultrastructure of the lung, the authors emphasize the specializations of the tissue in forming boundary spaces for air and blood. Morphometry reveals the quantitative relations among these three phases.
From the functional point of view, the organization of the lung may be defined in relation to the hierarchy of airways and blood vessels, from the trachea down to alveoli, or from the main stem of the pulmonary artery through the capillary network to the pulmonary veins entering the left atrium.
All of this is jointly considered in the scheme of Figure 3-1. Besides showing the three phases, the diagram introduces the three major functional zones of the lung: first, the conductive zone, consisting of air channels and blood vessels, the function of which is to guide and distribute air and blood into the peripheral lung units; second, the respiratory zone, composed of alveoli and capillaries; and third, the intermediate or transitory zone, containing elements of both.
Compartmental Distribution of Lung Volume
Any morphologic analysis of the functional capacity of the gas-exchange apparatus requires exact knowledge of the total lung volume and of its compartmental distribution. To illustrate the distribution of the lung volume among the various zones and constituents, consider the lung of a medium-sized adult inflated to about three-fourths of total lung capacity; the total lung volume then amounts to about 5.7 L. Table 3-1 gives the approximate distribution of this volume among the lung compartments as derived from morphometric analysis of fixed lungs. The greatest compartment is the airspace, of which about two-thirds is in alveoli and only a small fraction in conductive airways, representing the anatomic dead space.
Fine Structure of the Lung
Fine Structure of Conducting Airways
The conducting airways are a system of tubes that multiply toward the periphery by division according to the principle of irregular dichotomy. From the trachea to bronchi to bronchioles, the structure of the airway gradually changes. Common to all is the general scheme of a three-layered wall made of a mucosa, a muscle layer, a connective tissue sheath (Fig. 3-2), and the presence of a typical ciliated epithelium, which is described here first.
Lining Epithelium of Conductive Airways
The inspired air must be humidified and warmed before it reaches the delicate gas-exchange area; furthermore, air pollutants and dust, as well as airborne microorganisms, must be removed. Although the upper respiratory tract, in particular the nasal portion, is especially designed for these functions, the respiratory epithelium (Fig. 3-3), of all conducting airways, shows special features for the handling of airborne particles. The respiratory epithelium is a ciliated pseudostratified columnar epithelium with numerous scattered goblet cells. Ciliated cells occur from the trachea down to the last respiratory bronchiole, but their height decreases with the reduction of the airway diameter; ciliated cells of the trachea are columnar (Fig. 3-4), whereas those of the respiratory bronchioles are cuboidal (Fig. 3-5). The frequency of goblet cells also decreases toward the periphery; in bronchioles, they are replaced by Clara cells (see Fig. 3-5).59,72 Clara cells account for about 11% of the total epithelial cell number in terminal bronchioles and about 22% in respiratory bronchioles.5 A characteristic feature of Clara cells is the presence of membrane-bound electron-dense granules. Among the secretory products of Clara cells is the so-called Clara cell
secretory protein (CCSP). Although the detailed functions of CCSP in the human lung remain to be elucidated, experimental studies suggest immunomodulatory functions for CCSP. The Clara cell is also the main site of cytochrome P450 activity in the lung, illustrating their role in detoxification of foreign substances. Cytologic, kinetic, and histochemical studies7,43,44,78,79 have provided insights into the cell types of the airway epithelium of various species, including humans. In investigations, great emphasis also has been put on the neuroendocrine cells interspersed in the epithelium, often called Feyrter, Kulchitsky, amine precursor uptake and decarboxylation (APUD), or small granule cells. These neuroendocrine cells are present in the respiratory tract of all vertebrate species investigated so far. Occasionally, they are arranged in groups termed neuroepithelial bodies.1,19,38,51,75,77 Neuroepithelial bodies are extensively innervated and are assumed to function as chemoreceptors; they may act on pulmonary vascular or airway smooth muscle by secreting vasoactive substances.
secretory protein (CCSP). Although the detailed functions of CCSP in the human lung remain to be elucidated, experimental studies suggest immunomodulatory functions for CCSP. The Clara cell is also the main site of cytochrome P450 activity in the lung, illustrating their role in detoxification of foreign substances. Cytologic, kinetic, and histochemical studies7,43,44,78,79 have provided insights into the cell types of the airway epithelium of various species, including humans. In investigations, great emphasis also has been put on the neuroendocrine cells interspersed in the epithelium, often called Feyrter, Kulchitsky, amine precursor uptake and decarboxylation (APUD), or small granule cells. These neuroendocrine cells are present in the respiratory tract of all vertebrate species investigated so far. Occasionally, they are arranged in groups termed neuroepithelial bodies.1,19,38,51,75,77 Neuroepithelial bodies are extensively innervated and are assumed to function as chemoreceptors; they may act on pulmonary vascular or airway smooth muscle by secreting vasoactive substances.
![]() Figure 3-1. Schematic representation of lung zones. A, airways; PA, pulmonary artery; PV, pulmonary vein. |
The frequently found basal cells represent a proliferative pool of undifferentiated cells that are thought to replace the overlying cells on differentiation and maturation. Less common are the brush cells and migratory cells. Finally, the occurrence of naked nerve endings between individual cells, more frequent in the trachea and large bronchi, should be mentioned. They are thought to be irritant receptors.
Table 3-1 Approximate Distribution of Total Lung Volume in Milliliters for Adult Human Lung at Three-fourths Total Lung Capacitya | ||||||||||||||||||||||||||||
---|---|---|---|---|---|---|---|---|---|---|---|---|---|---|---|---|---|---|---|---|---|---|---|---|---|---|---|---|
|
Figure 3-3 is a schematic representation of a portion of the respiratory epithelium of a bronchus. The function of this epithelium is to capture airborne particles in a sticky mucous layer and to remove them efficiently from the lung. For this purpose, cilia show a synchronized rhythmic beat within a thin layer of low-viscosity fluid. On top, a blanket of mucus is moved in the direction of the pharynx, carrying intercepted particles. This cleaning mechanism can be compared with a conveyor belt and is often called the mucociliary escalator. The mechanism of mucus propulsion has been described in detail.105 The mucous layer is secreted onto the epithelial surface by goblet cells and by seromucous glands located in the walls of trachea and bronchi (see Fig. 3-2). The small bronchioles are most likely devoid of mucus because their wall contains neither goblet cells nor glands. Their surface is formed by a fluid layer of low viscosity that is topped by a thin osmiophilic film representing airway surfactant.31 Finally, the presence in the bronchial secretion of several humoral agents, which would protect the airways against infections, has been reported.
Trachea and Bronchi
Trachea and bronchi are characterized by the presence of cartilage within the fibrous sheath of their walls (see Fig. 3-2). In the trachea and stem bronchi, the cartilage is in the form of incomplete rings; in the trachea, these cover the ventral and lateral aspects, whereas the dorsal wall contains a strong layer of transverse smooth muscle. After about the second or third generation, these rings are gradually replaced by irregular cartilage plates, and a layer of smooth muscle appears between mucosa and cartilage.
All conducting airways are surrounded by an external, loose connective tissue sheath (see Fig. 3-2), which is continuous with the other connective elements of the lung. It is a structure of considerable physiologic significance because it contains both bronchial vessels to supply the bronchial wall with blood from the systemic circulation as well as nerves and lymphatic vessels.
Only a small part of the arterial bronchial flow, in some species as little as 25%, is drained by the bronchial veins. Most of it goes into the peribronchial venous plexus and from there into the pulmonary veins, forming a small right-to-left shunt. The bronchus is accompanied usually by a branch of the pulmonary artery, which is enveloped by connective tissue continuous with the peribronchial sheath, thus forming the so-called peribronchovascular space, a preferential site of leukocyte infiltration or edema formation under pathologic conditions.
Only a small part of the arterial bronchial flow, in some species as little as 25%, is drained by the bronchial veins. Most of it goes into the peribronchial venous plexus and from there into the pulmonary veins, forming a small right-to-left shunt. The bronchus is accompanied usually by a branch of the pulmonary artery, which is enveloped by connective tissue continuous with the peribronchial sheath, thus forming the so-called peribronchovascular space, a preferential site of leukocyte infiltration or edema formation under pathologic conditions.
Lymphatic vessels contained in these peribronchial and perivascular sheaths, as well as in the subpleural and septal connective tissue, constitute the main drainage path for the interstitial fluid.
Bronchioles
A bronchiole is an airway devoid of cartilage and seromucous glands; goblet cells are rare. Because airway structure does not change abruptly, either seromucous glands or goblet cells may still be present in transitional zones. Bronchioles are rather small conducting airways, measuring about 1 mm or less in diameter. Their added cross-sectional area is such, however, that they are not supposed to contribute substantially to the flow resistance of the airways in the normally breathing healthy individual. Their walls are generally thin and molded into the surrounding parenchyma. They are supplied with blood from the lesser circulation rather than from bronchial arteries. The bronchiolar mucosa is lined by a simple cuboidal epithelium (see Fig. 3-5)
composed of ciliated cells and Clara cells, which, as mentioned in the section discussing epithelial properties, have a dual detoxifying and secretory function. They are the object of intense study, and their exocrine secretory activity is being linked with a modulation of the inflammatory and immunologic response to injury. Their position as defensive cells is crucial, because in the normal respiratory tidal range, bronchioles are the last conducting airways to receive bulk air in conjunction with inspiration. In bronchioles, Clara cells take the place of goblet cells found in larger airways.
composed of ciliated cells and Clara cells, which, as mentioned in the section discussing epithelial properties, have a dual detoxifying and secretory function. They are the object of intense study, and their exocrine secretory activity is being linked with a modulation of the inflammatory and immunologic response to injury. Their position as defensive cells is crucial, because in the normal respiratory tidal range, bronchioles are the last conducting airways to receive bulk air in conjunction with inspiration. In bronchioles, Clara cells take the place of goblet cells found in larger airways.
In bronchioles, the smooth muscle cells form a well-developed, relatively thick layer arranged in a geodesic network, capable of narrowing the airway.
Resistance to Airflow in Conducting Airways
The partition of airflow resistance between large and small airways both in health and disease has been controversial. It is universally accepted that, in the healthy lung, the major site of resistance is the large, central airways, whereas the bronchioles contribute less than 20%, but it is important to know that pathologic increases of resistance always occur in the bronchiolar region. Airflow in the trachea is turbulent; in the bronchioles, it is laminar. In between, it is often referred to as transitional, implying an admixture of both, although experimental studies are difficult to perform. One of the major contributions to the understanding of the pathophysiology of emphysema was the clarification of the mechanisms of early airway closure.
A priori examination of the bronchiolar anatomy immediately reveals the factors that account for their active and passive narrowing: smooth muscle, compression by neighboring parenchyma during inflation, and internal surface tension. The elements that counteract the narrowing and act to cause bronchiolar dilation are less evident, however, made possible only by the radial insertion of alveolar walls in their periphery and by the principle of mechanical interdependence. The integrity of alveolar walls is therefore essential in keeping bronchioles open during deflation. In conditions such as emphysema, in which alveolar walls are lost, the loss of bronchiolar support causes a calamitous early collapse of small bronchioles at the onset of expiration, with trapping of air in all areas of the parenchyma located behind the obstruction.
Fine Structure of Transitory Airways
Respiratory Bronchioles
The last generation of exclusively conducting bronchioles is the terminal bronchioles. These branch to form about three generations of respiratory bronchioles (Fig. 3-6), which have essentially the same structure as other bronchioles except that, here and there and increasingly toward the periphery, the continuity of their wall is interrupted by areas of typical gas-exchanging tissue. The cuboidal epithelial cells of respiratory bronchioles are in most cases ciliated; short cilia can even be demonstrated in close proximity to alveoli.
Alveolar Ducts and Sacs
The mammalian airways form a blind-ending system. Dichotomy as a branching pattern can be demonstrated up to the last ranks of the airway system, the alveolar ducts and alveolar sacs (see Fig. 3-6). These structures differ from the bronchioles described previously in that they lack a proper wall; instead, their wall is formed by the openings of alveoli (Fig. 3-7), and their epithelial lining is nothing more than extensions of squamous alveolar epithelial cells. It is generally admitted that three generations of alveolar ducts immediately follow the last respiratory bronchioles. Finally, the last ducts give rise to two alveolar sacs. An alveolar sac represents the blind end of the airway branching system.
Fine Structure of the Gas-Exchange Region
In the respiratory zone of the lung, the blood is spread in capillaries in the walls of the alveoli. The air–blood contact becomes intimate, and gas exchange can take place.
Alveoli
Alveoli are small pouches placed in groups around respiratory bronchioles, alveolar ducts, and alveolar sacs. They are polyhedral structures lacking one side (the mouth, which opens into the airways), and they have been compared with the cells of a
honeycomb (see Fig. 3-7) or with the air bubbles in foam. A polygonal shape in general is economical because it allows a close packing of the alveoli. Studies on human pulmonary acini revealed that the shape of alveoli is not simple and that often an alveolus appears like a cluster of several connected pouches, as in Figure 3-7B.34 Furthermore, alveolar shape also depends on the degree of lung inflation.28 Only in fully inflated lungs has the alveolar configuration some similarity to the cells of a honeycomb. At lower inflation degrees, alveoli are often cup-like.
honeycomb (see Fig. 3-7) or with the air bubbles in foam. A polygonal shape in general is economical because it allows a close packing of the alveoli. Studies on human pulmonary acini revealed that the shape of alveoli is not simple and that often an alveolus appears like a cluster of several connected pouches, as in Figure 3-7B.34 Furthermore, alveolar shape also depends on the degree of lung inflation.28 Only in fully inflated lungs has the alveolar configuration some similarity to the cells of a honeycomb. At lower inflation degrees, alveoli are often cup-like.
The alveolar wall is always common to two adjacent alveoli and is called the alveolar or interalveolar septum (see Fig. 3-7B). The most conspicuous feature of the septum is a single but dense network of capillaries, which is shown in Figure 3-8 in face view. Sometimes, the septa are interrupted by interalveolar pores of Kohn, which are covered by surfactant. The septa also contain a skeleton of connective tissue fibers that is especially well developed around the mouth of the alveoli, where it forms a polygonal ring, the alveolar entrance ring (see Fig. 3-7), and may contain smooth muscle cells. The collagenous and elastic fibrous elements form a three-dimensional continuum that extends from the pleura to the hilum. This continuum ensures transmission of chest and diaphragmatic movements into the deeper regions of the lung, but it contributes only a smaller part to the retracting force of the lung, the major part being caused by surface forces.96,91
In the adult, the number of alveoli averages 300 to 500 million.69,89 This number is related to body length and lung volume and may vary largely between 200 and 800 million.2,69 For a lung of an adult inflated to three-fourths of its maximal volume, the average alveolar diameter lies between 200 and 290 μm.69,89 It has been shown in dog lungs that alveolar size is not identical in all parts of the lung, but that in an erect lung, the upper parts contain larger alveoli than the dependent parts because of the weight of the lung tissue.32
Alveolocapillary Tissue Barrier
Figure 3-9 shows a section of a small portion of an interalveolar septum with a capillary. The septum is lined on both sides by alveolar epithelial cells, which, in this instance, are thin. The capillary is lined also by a single squamous cell layer, the endothelium. Together with the intercalated connective tissue, these two cell layers constitute the alveolocapillary tissue barrier, which is the structure separating air and blood in the pulmonary gas-exchange region. It is supplemented by an extremely thin extracellular lining layer that contains macrophages (Figs. 3-10 and 3-11).
The morphometric characteristics of the cell population that constitutes this tissue barrier in the human lung are shown in Table 3-2.
The morphometric characteristics of the cell population that constitutes this tissue barrier in the human lung are shown in Table 3-2.
![]() Figure 3-9. Electron micrograph of alveolar capillary (C) from monkey lung with erythrocyte (EC). Note endothelial cell lining of capillary (EN), processes of pericytes (P), and the thin extensions of squamous type I alveolar epithelial cells (EP) covering the alveolar surface. The interstitial space (IN) is bounded by two basement membranes (BM) and contains some fibroblast processes (FB) as well as a few connective tissue fibrils. This lung was fixed by instillation of fixative into airways, resulting in a loss of the surface lining layer; hence, only parts 2 (tissue barrier), 3 (blood plasma), and 4 (erythrocyte) of the gas-exchange pathway are preserved, whereas the first layer, the surface-lining layer (Fig. 3-10), is missing (×8,600). (From Weibel ER. Morphometric estimation of pulmonary diffusion capacity. I. Model and method. Respir Physiol 1971;11:54. With permission.) |
Epithelium
The epithelium of the alveoli is continuous, although its thickness in places reaches only 0.1 to 0.3 μm, which is at the limit of resolution of the light microscope. Early electron microscopic studies brought the first conclusive evidence for an uninterrupted epithelial lining of alveoli.52 It consists of the following cell types (see Table 3-2):
Alveolar epithelial type I cells (Fig. 3-12), also called squamous cells or type I pneumocytes, send out broad, thin cytoplasmic extensions. Although they are some 30% to 40% less numerous than the type II cells, they cover 92% to 95% of the total alveolar surface. The nuclei lie in depressions
between two capillaries. These cells are poor in organelles, such as mitochondria or endoplasmic reticulum, which are confined to the perinuclear cytoplasm, whereas the cytoplasmic extensions essentially contain only pinocytotic vesicles. In humans, a single type I cell covers some 5,000 μm2 of the alveolar surface, on the average.18 Type I cells cannot divide by mitosis. Thus, normal alveolar epithelial cell turnover and epithelial regeneration after injury is accomplished by proliferating and differentiating type II cells.
Table 3-2 Morphometric Characteristics of Cell Population in the Human Alveolar Septal Tissue
Cell number
Average cell
Absolute (n × 109)
Relative (%)
Volume (μm3)
Apical surface (μm2)
Pneumocytes I
19
8.3
1,763
5,098
Pneumocytes II
37
15.9
889
183
Endothelial cells
68
30.2
632
1,353
Interstitial cells
84
36.1
637
—
Macrophages
23
9.4
2,491
—
Source: From Crapo JD, et al. Cell numbers and cell characteristics of the normal human lung. Am Rev Respir Dis 1982;126:332. With permission.
Alveolar epithelial type II cells are cuboidal (Fig. 3-13). These cells have also been called granular pneumocytes, type II pneumocytes, or great alveolar cells, although they are smaller than type I cells. They have no cytoplasmic extensions and typically are in niches between capillaries of the alveolar septum. Their free surface is covered by somewhat irregular microvilli. The cells occupy 5% to 8% of the alveolar surface and form tight junctional complexes with neighboring alveolar type I cells. Compared with alveolar type I cells, the type II cell is rich in mitochondria, endoplasmic reticulum, Golgi apparatus, and multivesicular bodies. Their most distinctive morphologic feature, however, is the presence of lamellar osmiophilic inclusions, the lamellar bodies, unique organelles known as the sites of storage of pulmonary surfactant.3,29,36,87 The synthesis, storage, secretion and at least partial recycling of surfactant components is one of the two main functions of type II cells. The other is the contribution to alveolar epithelial regeneration under physiologic and pathologic conditions. Thus, the alveolar epithelial type II cell is regarded as the “defender of the alveolus.”22,57
A third pneumocyte, the brush cell, was first described in the rat lung.64 In the rat, this cell can be found in terminal bronchioles; it is, however, rare in the gas-exchange zone. The brush cell is characterized by a spray of rather thick and regular cylindrical microvilli at the surface and by thick bundles of microfibrils in the cytoplasm. Brush cells are large, but only a small part of their membrane reaches the epithelial surface. Similar cells occur in larger airway epithelia and in other organs also; their significance is still obscure.73
Interstitium
The interstitium is the space between the basal laminae of alveolar epithelium and capillary endothelium (see Fig. 3-9). It contains connective tissue and interstitial fluid. The connective tissue comprises cells, fibers, and an amorphous substance containing proteoglycans, allegedly in a gel matrix. The distribution of connective tissue can vary considerably. In places where the air–blood barrier is thin, connective tissue may be reduced to a few isolated fine fibrils or may even be absent, in which case the adjoining basement membranes fuse. These last regions are particularly important for gas exchange. In lung edema, they are usually not widened by interstitial fluid and can therefore be called restricted, as opposed to those unrestricted, thicker portions of interstitium between capillaries, where interstitial fluid can accumulate under pathologic conditions. The interstitial fibroblasts have been demonstrated to contain contractile
filaments (see Fig. 3-12); it has therefore been suggested that they could regulate blood flow through the alveolar septum.45,46 In view of the interstitial structure described, an alternative function for these cells has been proposed95: they could control the compliance of the unrestricted interstitial regions by regulating the width of the septum. In rodents, particularly during postnatal development, the interstitial cells form two distinct populations of cells: a lipid-containing and a non–lipid-containing type. The lipid droplets of the lipid-containing type mostly disappear, however, before weaning.53,62 Lymphatic vessels are never found in alveolar septa. Nevertheless, a continuous path of the interstitial fluid toward the lymphatics of the subpleural space and of the peribronchial and perivascular connective sheaths has been postulated; the fluid probably follows connective fibers.
filaments (see Fig. 3-12); it has therefore been suggested that they could regulate blood flow through the alveolar septum.45,46 In view of the interstitial structure described, an alternative function for these cells has been proposed95: they could control the compliance of the unrestricted interstitial regions by regulating the width of the septum. In rodents, particularly during postnatal development, the interstitial cells form two distinct populations of cells: a lipid-containing and a non–lipid-containing type. The lipid droplets of the lipid-containing type mostly disappear, however, before weaning.53,62 Lymphatic vessels are never found in alveolar septa. Nevertheless, a continuous path of the interstitial fluid toward the lymphatics of the subpleural space and of the peribronchial and perivascular connective sheaths has been postulated; the fluid probably follows connective fibers.
Endothelium
The endothelial cells form a capillary wall that is similar in structure to the endothelium in some other organs (Fig. 3-14; see Figs. 3-9 and 3-10). The cells form thin cytoplasmic extensions and hence resemble the alveolar type I epithelial cells. A single cell covers between 1,000 and 1,500 μm2 of the capillary lumen. Lung capillaries have no fenestrations. Further details are discussed below.
Extracellular Lining Layer and Pulmonary Surfactant
On the basis of theoretical considerations, as early as 1929, von Neergaard84 predicted that the alveolar surface must be lined by a surface-active substance, now commonly called pulmonary surfactant.17 It is an essential element, ensuring the stability of the air-filled lung. Its basic biophysical characteristics are twofold: first, it lowers the surface tension at the air–liquid interface of the alveoli; and second, its surface tension is variable with the degree of inflation of alveoli. In addition, certain surfactant components have important immunomodulatory functions in the lung.104
Morphologic demonstration of pulmonary surfactant is possible only with the electron microscope. In routine preparations, usually no traces of this material are found (see Fig. 3-9). In lungs fixed by vascular perfusion, an extracellular duplex lining layer on the alveolar surface, consisting of a surface film and a hypophase, can be preserved (see Fig. 3-10).30 Much of this material forms pools in pits and irregularities of the alveolar wall, which smoothes it out. These pools in the hypophase are polymorphous, consisting of several surfactant subtypes representing different stages in intra-alveolar surfactant metabolism. Freshly secreted surfactant is present as lamellar body-like forms that rapidly transform into tubular myelin figures with a characteristic lattice-like structure. Although the precise function of tubular myelin is still unknown, it is thought to be the immediate precursor of the surface film. Inactive surfactant components can be found in the hypophase as small unilamellar vesicles.
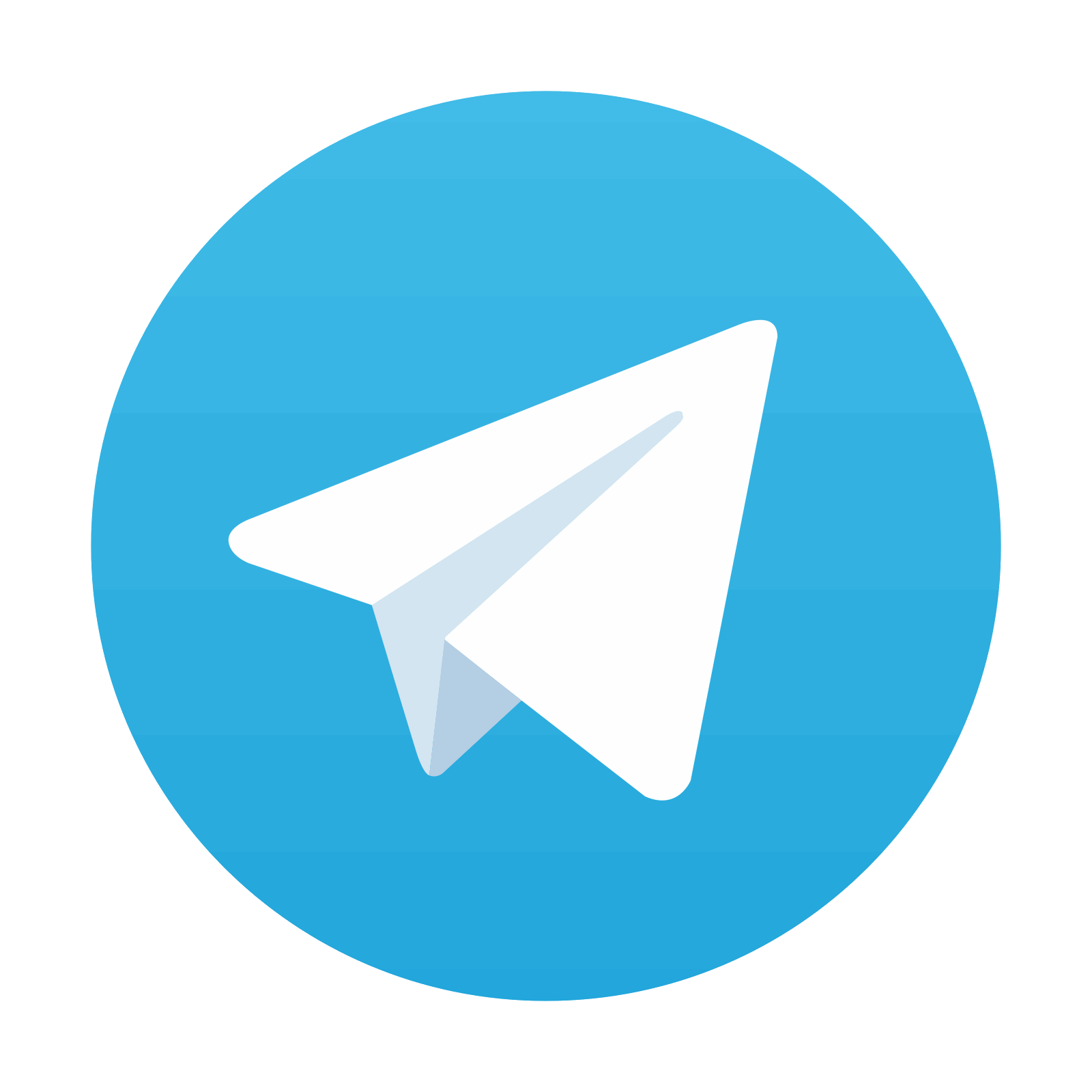
Stay updated, free articles. Join our Telegram channel

Full access? Get Clinical Tree
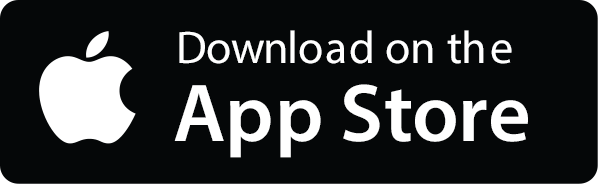
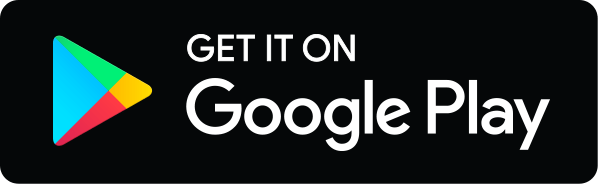
