Ultrasound Contrast Agents— Fundamentals and Principles of Use
4.1Structure and Properties of Ultrasound Contrast Agents
4.2Equipment and Software: Settings and Transducers
4.3Vessel- and Organ-Specific Contrast Doses
4 Ultrasound Contrast Agents—Fundamentals and Principles of Use
4.1 Structure and Properties of Ultrasound Contrast Agents
4.1.1 Structure
Ultrasound contrast agents are composed of microscopic bubbles approximately 2 to 10 μm in diameter, comparable in size to red blood cells. The microbubbles are filled with gas and encapsulated in a stabilizing shell. The gas in the first generation of microbubbles consisted of ordinary room air. But the main chemical components of air (nitrogen, oxygen, and carbon dioxide) are highly soluble in blood and therefore diffuse quickly out of the microbubbles, causing them to become unstable shortly after injection. Air microbubbles must be stabilized by a very thick shell (e.g., of glucose or denatured albumin) to ensure an adequate duration of enhancement.
Newer, second-generation contrast agents have a lipophilic gas core that is poorly soluble in water and resists diffusion from the microbubbles in the aqueous medium of blood. This gives the microbubbles much longer stability in vivo, and a very thin (elastic) shell can be used. The gas core normally consists of sulfur hexafluoride or perfluorocarbons, which are pharmacologically inert, nontoxic, and extremely stable. Phospholipid membranes provide an ideal shell for these gases. Amphiphilic phospholipids consist of a hydrophilic head and two lipophilic fatty acid tails, which self-align in aqueous solution to form a membrane in which all the heads point outward toward the aqueous medium while the fatty acid tails point inward toward the gas core (Fig. 4.1). This phospholipid monolayer forms a thin, elastic membrane that stabilizes the gas microbubbles and prevents their aggregation and coalescence owing to its negative surface charge.
Fig. 4.1 Diagram of an ultrasound contrast microbubble. The gas-filled microbubble is encapsulated by a thin phospholipid shell. Amphiphilic phospholipids self-assemble to form a monolayer in which the hydrophilic phosphate heads point outward toward the blood and the lipophilic fatty acid chains point inward toward the gas core.
The microbubble shell creates an interface between the gas core and surrounding blood. An incoming ultrasound wave is backscattered at this interface owing to the acoustic impedance mismatch between the gas and blood. As a result, the microbubbles generate a strong echo, making them an effective ultrasound contrast agent (Table 4.1). Different formulations differ in the type of gas used, the composition and elasticity of the membrane shell, the range of approved indications, and the geographic availability.
The echo returning from contrast microbubbles is considerably stronger than one would expect based on their scattering cross section (backscattering of the echo signal). This results from the manner in which the incident sound wave interacts with the microbubbles. The transmission frequencies used in diagnostic ultrasound (approximately 2–15 MHz) are within the resonant frequency range of the microbubbles; this causes them to oscillate and generate a very intense, microbubble-specific signal (Chapter 3 and Fig. 4.3).
4.1.2 Pharmacologic Properties of Ultrasound Contrast Agents
Ultrasound contrast microbubbles have the same velocity and distribution in the bloodstream as red blood cells. Their size and elastic compliance enable them to pass through the capillaries, but they cannot leave the vascular system. This distinguishes ultrasound contrast agents from the standard iodinated and gadolinium contrast media used in computed tomography (CT) and magnetic resonance imaging (MRI). The latter are extracellular media that extravasate from the blood vessels and become distributed throughout the interstitial fluid space, whereas ultrasound contrast agents are distributed exclusively in the blood (true blood-pool contrast agents).
Because of its lipophilic properties, almost none of the microbubble gas can diffuse into the blood. The only site where it can leave the microbubbles is in the lung capillaries, where it can pass directly into the alveolar airspace. From there the gas is expelled from the body by exhalation. The total gas volume administered in microbubbles is extremely small (a few μL) and is completely cleared from the lungs in approximately 20 to 30 minutes.1 The phospholipids in the microbubble shell are natural constituents of the cell membrane and are metabolized through the natural pathways of phospholipid metabolism.
Given their inert properties, ultrasound contrast agents are very safe and well tolerated.2 They are non-nephrotoxic, making them a good alternative to iodinated and gadolinium-based media in patients with impaired renal function. Side effects are rare and are usually mild.3 Due to their colloidal properties, however, there have been rare instances of anaphylactoid reactions with potentially severe respiratory and cardiovascular complications or even cardiac arrest. Anaphylactoid reactions have a reported incidence of approximately 1 in 10,000 patients. Thus, centers where ultrasound contrast agents are used should have an emergency protocol in place for the immediate management of these complications.
4.1.3 Acoustic Properties of Ultrasound Contrast Agents
The behavior of microbubbles in a sound field depends greatly on the intensity of the sound wave, or more precisely on the local acoustic pressure (acoustic power) and its fluctuations in the ultrasound field (Fig. 4.2).4 When the pressure fluctuations are very small, an incident sound wave is simply backscattered at the microbubble interface. But as the pressure fluctuations increase, the microbubbles will contract in the positive-pressure phase and expand in the negative-pressure phase due to the elastic properties of the shell. This induces a rapid oscillation of the microbubbles, which is strongest at their resonant frequency. That frequency depends on the size of the microbubbles and coincides with the range of transmission frequencies used in medical ultrasound devices. When exposed to an ultrasound field, therefore, microbubbles become highly efficient resonators that produce a characteristic (nonlinear) signal response.
Fig. 4.2 Oscillation of ultrasound contrast microbubbles in a sound field. At a very low acoustic pressure (low power output), the transmitted signal is simply backscattered. But when the acoustic pressure is increased by only a slight amount, the microbubbles begin to oscillate and produce a microbubble-specific echo signal at their resonant frequency, along with associated upper and lower harmonic echoes. This signal is specific for the contrast agent. As the acoustic pressure continues to rise, the microbubbles oscillate so vigorously that their shell finally ruptures and all the gas escapes. Thus, high power outputs destroy the microbubbles and render them ineffective as contrast agents. MI, mechanical index.
In modern formulations that have a very thin, elastic shell (LumasonTM, DefinityTM), these resonant oscillations occur even at an extremely low sound intensity, meaning that these products are excellent for ultrasound imaging at a low power setting. The acoustic power output on most ultrasound machines is displayed as the mechanical index (MI), so scanning at a reduced power setting is also known as “low MI imaging.”
As sound intensity increases, the microbubbles undergo increasingly strong resonant oscillations until finally their shell ruptures and the contrast microbubbles are destroyed. This is particularly common in regions with slow blood flow (e.g., capillary beds) where the circulating microbubbles are exposed to the sound field for a longer period of time. In this case microbubble destruction can occur even at standard B-mode sound intensities, and therefore the power setting for contrast-enhanced imaging should be substantially reduced, especially in parenchymal organs.
The specific signal response of oscillating microbubbles includes harmonic frequency components (“overtones”) that are not present in the signals returning from blood and tissue. With suitable technology, therefore, tissue signals can be distinguished from contrast signals and displayed separately (Fig. 4.3).5 In this technology, called contrast-enhanced ultrasound (CEUS), the contrast signal is often displayed in a specific color (e.g., gold) that is clearly distinguishable from tissue signals in the B-mode image. The contrast-enhanced portion of the image can be isolated (by suppressing the tissue signal) and displayed side-by-side with the tissue image in a split-screen format or superimposed over the gray scale tissue image.
Various filtering techniques are used to separate the nonlinear contrast signals from tissue signals in real time during the examination. Originally a simple frequency filter technique (second harmonic imaging) was used for this purpose. In this technique the transmission frequency is filtered out of the signal response and only higher frequencies are displayed. These occur mainly at the resonant frequencies of the microbubbles. In second harmonic imaging, however, a large portion of the contrast signal is filtered out (the nonlinear portions in the fundamental frequency range) so that imaging requires a relatively high power output in order to obtain a sufficiently strong contrast signal.
Fig. 4.3 Principle of pulse summation techniques. The transducer emits multiple pulses per scan line, which differ in their phase and/or amplitude (phase and amplitude modulation). The backscattered signals from tissue are similar in waveform to the transmitted echo pulses (linear signals), while the signals from microbubbles are produced by resonant oscillations and differ significantly from the transmitted signal in their frequency, phase, and amplitude (nonlinear signals). Electronic summation of the received signals in the contrast mode then leads to extinction (suppression) of the linear tissue signals while the nonlinear signals from the microbubbles are selectively displayed in the image.
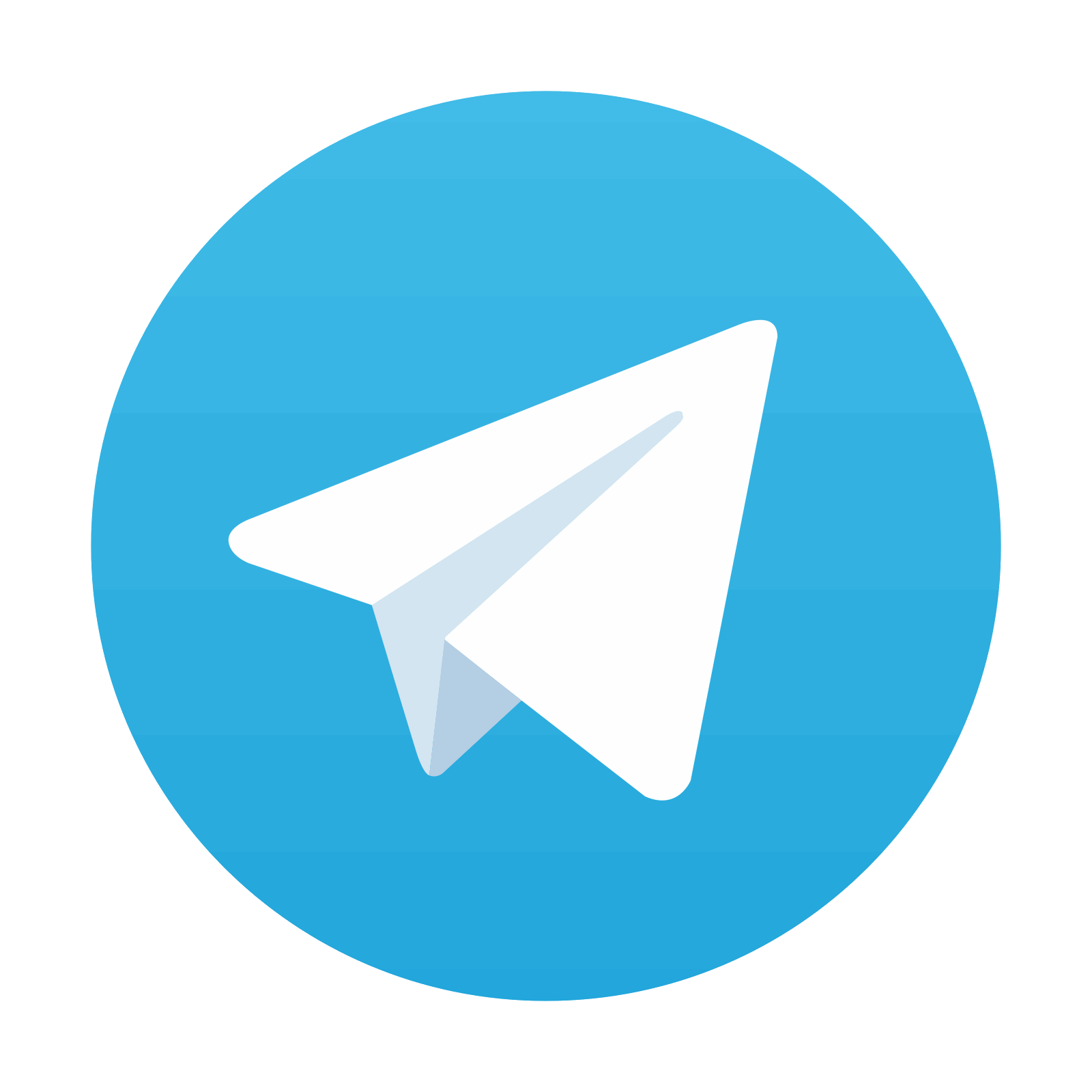
Stay updated, free articles. Join our Telegram channel

Full access? Get Clinical Tree
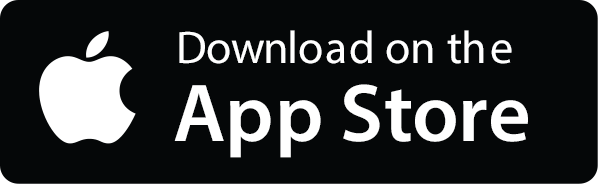
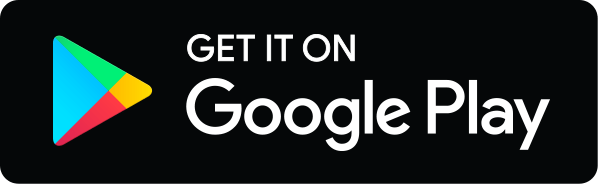